The inflammatory microenvironment in brain metastases: potential treatment target?
Introduction
Brain metastases (BM) are devastating complications occurring in up to 40% of cancer patients. Only limited treatment options exist upon the occurrence of BM, resulting in the limited survival prognosis of BM patients ranging from only about few weeks to months (1). There is a strong need for the identification of novel treatment modalities to improve patient outcomes.
Recently the inflammatory microenvironment was identified to have prognostic and therapeutic implications in various malignancies. Infiltration with T-cell as well as tumor associated macrophages (TAMs) correlate with patients’ survival times in several extracranial malignancies (2-5). Of particular importance is the recent introduction of effective immunomodulatory anti-cancer agents. The monoclonal antibodies ipilimumab, nivolumab and pembrolizumab activate the anti-tumor T-cell response by interfering with tumor-associated immunosuppression induced via the immune checkpoint molecules. Ipilimumab inhibits the T-cell co-receptor cytotoxic T lymphocyte antigen 4 (CTLA4), while nivolumab and pembrolizumab inhibit the T-cell co-receptor programmed cell death 1 (PD1). These drugs have shown remarkable and durable responses in several tumor types including melanoma, lung cancer, Hodgkin’s lymphoma and others (6-8). A large number of clinical trials evaluating immune checkpoint inhibitors in various malignancies are underway and immunotherapy is very likely to quickly become a standard treatment modality in oncology. However, so far little is known about the inflammatory microenvironment of BM and whether it could serve as feasible treatment target. The CNS has long been considered an “immune-privileged” organ with limited capacity of inflammatory response. However, this concept has been challenged and it is becoming clear that neoplastic processes in the brain may induce prominent anti-tumor immune responses. In the following we will discuss the characteristics of the inflammatory microenvironment in BM and its potential as clinical biomarker and therapeutic target.
Interaction of immune system and BM initiating cell in the brain metastatic cascade
Immune escape is an emerging hallmark of cancer (9). Presumably, BM initiating cancer cells have to evade immune attack several times during the brain metastatic cascade. However, besides immune escape, immune cells were postulated to have supporting functions in the process of metastatic spread and are adapted by the cancer cell to support their growth. In the following paragraph we discuss the steps of the brain metastatic cascade and the involvement of the immune system in the single steps of BM development.
First BM initiating cells have to disconnect from the primary tumor (or an extra-cranial metastasis) and enter the blood stream. Here, TAMs were shown to have a metastasis promoting function as they contribute to the degradation of the extracellular matrix and thereby facilitate intravasation (2). Macrophages were identified to enhance cancer cell intravasation upon physical contact as observed by using high resolution imaging techniques including repetitive in vivo multi-photon laser microscopy. Cancer cell—macrophage contact promotes formation of actin-rich degradative protrusion enabling the cancer cell to degrade matrix barriers in the process of trans-endothelial migration (10). Further, tumor cells were shown to synthesize colony-stimulating factor 1 (CSF1) to stimulate macrophages. This causes the clustering of TAMs and cancer cells around blood vessels and promotes intravasation and the formation of metastases (11). In line, experiments with macrophage-deficient mice showed normal progression of the primary tumor but prevention of metastases outgrowth (12).
Further, T cells were shown to impact the metastatic potential of a primary tumor. Dense infiltration with tumor infiltrating lymphocytes (TILs) was shown to correlate with survival prognosis in various extracranial malignancies, arguing that TIL density might reduce metastatic spread (3). In line, preclinical models revealed that depletion of CD4+ and CD8+ T cells results in the increased development of BM (13).
Upon arrival in the brain vasculature, cytokines were shown to promote the site-specific metastatic behavior of BM initiating cancer cell. Of particular interest in the brain metastatic cascade are the chemokine receptor CXCR4 and its ligand CXCL12 (stromal cell derived factor 1 alpha). The chemokine pair CXCR4/CXCL12 were shown to contribute to brain-specific metastatic behavior by promoting adhesion of the BM initiating cells to the brain vasculature and further facilitate the migration trough the brain microvascular endothelial cells (14,15).
The process of extravasation from the vasculature into the parenchyma was shown to be promoted by TAMs in the case of extracranial malignancies. Co extravasation might have tumor promoting properties, as TAMs can express growth and survival factors, promote angiogenesis and remodeling of the extracellular matrix like during the process of intravasation (2,12,16). The specific recruitment of TAMs might even proceed the BM development as they were shown to be involved in the preparation of the “pre-metastatic niche”, i.e., the preparation of a certain site for the homing of circulating tumor cells (17). Further, preclinical models suggest that myeloid derived suppressor cells are also involved in the formation of the “pre-metastatic niche” in breast cancer BM (18). Importantly, inhibition of TAMs as well as of myeloid derived suppressor cells was postulated to have preventive potential, as the interaction of these two cell types with BM initiating cells represents a pivotal step in the brain metastatic cascade (18,19). However, so far only few preclinical models have investigated this theory and further studies on the exact interaction of hosT cells and BM initiating cells are warranted.
Due to increased expression of vascular endothelial growth factor (VEGF) by TAMs, vascular permeability is increased and thereby extravasation is facilitated. After the trans-passing of the endothelium, TAMs promote cell survival, growth and invasion via the maintenance of CSF1 signaling within the perivascular niche (20,21). However, the exact involvement of TAMs and especially the importance of microglia cells with phagocytic activity in comparison to blood derived macrophages within the brain metastatic cascade remain to be investigated.
TAMs were associated with the “angiogenic switch”, i.e., induction of angiogenesis during the outgrowth from micro- to macrometastases. Neoangiogenesis is promoted by the production of VEGF by TAMs (22). This is of major interest in the brain metastatic cascade, as the induction of neoangiogenesis is considered one of the pivotal steps during BM outgrowth. The formation of NSCLC macrometastases was shown to depend on the induction of neoangiogenesis, as the prophylactic application of the VEGF antibody bevacizumab resulted in demise of micrometastases before the outgrowth of macrometastases (23).
Further, TAMs were shown to express interleukin 10 and transforming growth factor β (TGF-β) and thereby stimulate regulatory T cells, an immunosuppressive subpopulation of T cells characterized by the expression of FOXP3, which contribute to an immunosuppressive tumor microenvironment (24). In line, the combination of dense infiltration with TAMs and a high ratio of regulatory versus cytotoxic T cells are associated with poor survival in breast cancer (2).
Overall, the immune system seems to be important throughout multiple steps of BM formation and deeper insight into the interaction of cancer cells and the immune system may offer opportunities for rational drug-mediated interference with the goal to prevent of CNS metastases in cancer patients.
Composition and potential clinical value of the inflammatory microenvironment of established brain metastases (BM)
The main cell types involved in the inflammatory microenvironment of BM microglia/macrophages, B lymphocytes and T lymphocytes.
Microglia cell/macrophages
Microglia cells are the main effector cells of the innate immune system in the brain parenchyma (25). Under healthy condition, microglia cells are motile and constantly move through the brain parenchyma. Therefore a heterogeneous distribution of microglia cells can be observed within the healthy brain (26). Upon activation, microglia cells may exert several functions. First, microglia cell can induce cytotoxicity via the release of nitric oxide (NO). However, only minor expression of factors associated with NO release was observed within and around human BM, indicating that this cytotoxic pathway has only minor importance in BM (27). A second function of microglia encompasses phagocytosis (25). Microglia cells with phagocytic properties belong to the population of TAMs within BM. The population of TAMs however also encompasses blood-derived macrophages and no clear cut difference between macrophages of microglia origin and of blood origin can be made by the current available methods (21). Two functional phenotypes of TAMs were postulated. The M1 TAMs are defined by tumor suppressive functions like inhibition of cell proliferation. The M2 TAMs are postulated to have rather tumor promoting functions including suppression of adaptive immune response and promotion of tumor cell migration, invasion, as well as intravasation into the vascular system, angiogenesis and persistent growth (28,29).
A further important function of microglia cells in the inflammatory microenvironment of BM is T-cell activation via expression of the HLA ABC/major histocompatibility antigen class I. Therefore, microglia activation is mandatory for the induction of a specific immune response including T and B cells (25). However, microglia cells are also capable of immune suppressive functions. PD-L1 expression, the ligand of the inhibitory T-cell co-receptor PD1, was observed on microglia cells (30). Via activation of CTLA4 or PD1, microglia cells can suppress the anti-tumor T-cell response and participate in the generation of an immune suppressive tumor microenvironment. Microglia has also been described to enhance invasion and colonization of brain tissue by cancer cells by serving both as active transporters and guiding rails (31).
Myeloid-derived suppressor cells
Myeloid-derived suppressor cells are a heterogeneous population of immature myeloid cells and are involved in several immunosuppressive functions. Within cancer tissue they were shown to be highly involved of the formation of the “pre-metastatic niche” as they prepare the tissue for the infiltration of metastatic tumor cells (32). Further, myeloid-derived suppressor cells are known to inhibit T-cell activation via cytokine production and promote angiogenesis and facilitate tumor metastasis and invasion (3). Increased circulating of myeloid-derived suppressor cells is associated with poor prognosis in lung cancer patients (33).
T cells
T cells are the main effector cells of the adaptive immune system. Lymphocytes are mostly absent in the healthy brain parenchyma and migrate into the CNS under pathological condition (34). Various subtypes of TILs can be observed in the inflammatory microenvironment of BM and they differ in function and prognostic relevance (35). CD3+ TILs reflect the overall population of effector T cells. CD8+ TILs represent cytotoxic T-cell and CD45RO+ TILs, the so-called memory T cells, which are observed after an initial immune response as a sign of immunological memory. FOXP3+ TILs are so called regulatory T cells and have immunosuppressive function. They suppress the function of CD8+ cytotoxic T-cell and participate in the generation of an immune suppressive microenvironment. Importantly, dense infiltration with CD3+, CD8+ or CD45RO+ TILs is associated with improved survival prognosis in several extracranial malignancies like colorectal cancer, breast cancer and ovarian cancer (4,36,37). Dense infiltration with the immunosuppressive subtype of FOXP3+ TILs however is associated with impaired survival prognosis e.g. in lung cancer and breast cancer (38,39). Similar impact has been observed in liver and lung metastases of colorectal cancer as well as in BM, as patients with dense infiltration of TILs presented with an improved survival prognosis as compared to patients with only spare or absent TIL infiltration in the resected metastases (35,40,41).
B cells
The role of B cells in the inflammatory microenvironment has only been poorly investigated so far. Fewer B cells were observed in the matched BM as compared to the primary tumor in triple negative breast cancer (42). The involvement of B cell is supported by the observation that in preclinical models the metastatic burden is increased as a result of B cell depletion (43). The B cell depletion causes reduced T-cell response, thus arguing for a strong interaction of B cells and tumor repression via T-cell activation (16).
Potential role of TILs infiltration of brain metastases (BM) as biomarker
TIL infiltrates of variable density can be observed in BM and are typically composed of various cell types with a higher fraction of T cells than B cells (16,35,44). An accumulation of TILs was reported within the tumor stroma and in the border region to the surrounding brain parenchyma, while the intratumoral density was less pronounced. The lymphocytic infiltrates presented with a mixed composition of both immuno-activating TIL subsets such as CD3+ effector and CD8+ cytotoxic TILs, memory TIL subsets CD45R0+ and immunosuppressive TIL subsets such as FOXP3+, and PD1+ TILs. A strong correlation with survival prognosis was observed as patients with dense infiltration with of CD3+, CD8+ and CD45RO+ TILs presented with a significantly prolonged survival as compared to patients with only spare or scatted TIL infiltration. Furthermore, the Immunoscore, which is based on automated calculation of the CD3+/CD8+ ratio among TILs and has been elaborated in colorectal cancer specimens, showed an independent and strong impact also in BM patients (5,35). These findings argue for an impact of TIL infiltration irrespective of tumor stage and strongly support the application of immune modulatory agents also in patients with metastatic disease. Future studies are needed to determine whether TIL infiltration correlates with response to immunomodulators and may serve as clinically relevant predictive biomarker.
The inflammatory microenvironment as a potential therapeutic target in brain metastases (BM)
Recently a new class of anti-cancer agent, namely immune checkpoint inhibitors, has shown remarkable clinical activity in several extracranial malignancies, including some of the most frequent primary tumors causing BM, such as melanoma and non-small cell lung cancer (8,45,46). Immune checkpoint inhibitors exert their anti-cancer activity via the activation of the T-cell response, as they target inhibitory co-receptors of the T-cell receptor and thereby boost the anti-tumor T-cell response (47). The so far approved agents targeted the CTLA4 (ipilimumab) and the PD1 (pembrolizumab, nivolumab) axis.
Ideally BM patients would be selected based on predictive biomarkers for new targeted therapies, however little is known so far on predictive biomarkers for the response to immune checkpoint inhibitors (48). Dense infiltration with TILs was postulated as a predictive marker for response to ipilimumab, as in theory the increased T-cell response is more potent in an immune active microenvironment (49). An immune active microenvironment, as resembled by a dense infiltration of TILs, was observed in neuro-surgically resected BM, especially in melanoma, renal cell carcinoma and NSCLC BM (35). As re-biopsy is not always feasible, radiological surrogate parameters for the identification of an immune active microenvironment are of special interest in BM patients. Interestingly, a dense infiltration with CD8+ TILs showed an association with large peritumoral edema, which could serve as a surrogate parameter (50). Prospective clinical trials are warranted to further investigate the correlation of TIL infiltration and radiological parameters as well as the response to immune checkpoint Inhibitors.
Another proposed predictive biomarker for response to PD1 axis targeting immune checkpoint inhibitors is expression of the ligand of PD1, namely PD-L1, on the cancer cells (51). PD-L1 expression was frequently observed in melanoma and NSCLC BM specimens, indicating that the PD1 axis is also active BM and might serve as a potential treatment target (44,52).
Clinical data on immune checkpoint inhibitors in BM patients are scarce. So far only treatment with ipilimumab in patients with newly diagnosed melanoma BM has been investigated in a prospective clinical trial. ipilimumab monotherapy presented with intracranial response rates up to 24% in patients with newly diagnosed, asymptomatic melanoma BM, while symptomatic patients in need for steroid treatment presented only with response rates around 10% (53). The extracranial response rate for ipilimumab in newly diagnosed melanoma patients also ranges around 25%, indicating that the intracranial response rate in patients not in need for steroid treatment is comparable to the extracranial one (54). The reasons for the different response rates between symptomatic and asymptomatic patients might be caused by the steroid treatment. Steroid treatment is routinely used in BM patients to reduce the peritumoral edema and might resemble a challenge in the treatment of BM patients with immune checkpoint inhibitors as steroids suppress the immune response and therefore the immune checkpoint inhibitor treatment might not reveal its full potential in symptomatic patients under steroid treatment (53). So far little is known on the interaction of steroid treatment and the possibility of continued response to Immune Checkpoint Inhibitors. Retrospective investigations suggest that patients with immune related adverse events caused by immune checkpoint inhibitors might still respond after the need of steroid treatment (55). However, the up-front steroid treatment in BM patients might have a negative impact on antineoplastic efficacy of immune checkpoint inhibitions (56). Another challenge in the treatment of BM patients is the possibility of pseudo-progression and subsequent symptom burden due to increased edema. Patients might present with stabilization or even an increase in tumor size due to infiltrating lymphocytes before actual tumor shrinkage (57). In the special situation of BM, this phenomenon may be of particular concern and may require symptomatic treatment e.g., with steroids, which might influence the treatment potency. A possibility to overcome the clinical challenges associated with intracranial pseudoprogression might be the combination with an anti-angiogenic agent like bevacizumab. The VEGF antibody bevacizumab has shown favorable results in the treatment of peritumoral edema and (radio)necrosis in BM patients and might further have synergistic treatment effects (50). Further, BM patients have an impaired survival prognosis and are in need for an immediate tumor control. Therefore, BM patients might not experience the delayed tumor response of immune checkpoint inhibitors. The combination of a local radiotherapy with an immune checkpoint inhibitor treatment might therefore be a treatment option, proving local control and time for the immune checkpoint inhibitor to reveal its therapeutic potential (58). Further the so-called “abscopal” effect is of special interest in BM patients. The abscopal effect refers to the observation that an increased response to Immune Checkpoint Inhibitor treatment may occur in non-irradiated lesions after radiation of distinct tumor manifestations. In theory, the radiation causes increased antigen reveal and therefore an increased activation stimulus for the T cells (59). Some retrospective observations underscore the abscopal effect theory as patients receiving ipilimumab before or after radiation had an improved survival as compared to patients receiving ipilimumab before radiation only (58). However, increased rates of radio necrosis were observed in patients with melanoma BM treated with the combination of ipilimumab and stereotactic radiosurgery (58).
A further challenge is the response assessment as already mentioned an initial increase in tumor size and well as number of metastases followed by delayed response can be observed (57). Therefore, the Response Assessment in Neuro-Oncology (RANO) group founded a dedicated subdivision to formulate intracranial response criteria for patients treated with an immune checkpoint inhibitor (iRANO). Currently some studies investigate the impact of Immune Checkpoint Inhibitors as well as the sequencing with radiotherapy in patients with BM (Table 1).
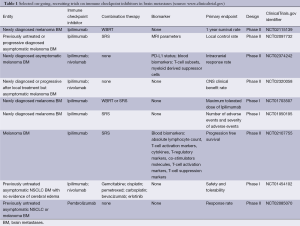
Full table
Conclusions
Several lines of evidence show a prominent involvement of the immune system in the pathobiology of BM. The interaction of cancer cells and the immune system seems to crucially involved in several steps of BM formation and established BM contain considerable inflammatory infiltrates composed of various immune cells. The density of TILs in BM has been described to correlate with patient survival times and emerging data support a clinically relevant activity of immunomodulatory drug therapy with immune checkpoint inhibitors in patients with BM. More research is needed to better understand the role of the immune system in BM and clinical trials should specifically enroll BM patients to investigate the risks and benefits of immune therapies in this patient population.
Acknowledgements
Disclosure: The authors declare no conflict of interest.
References
- Sperduto PW, Kased N, Roberge D, et al. Summary report on the graded prognostic assessment: an accurate and facile diagnosis-specific tool to estimate survival for patients with brain metastases. J Clin Oncol 2012;30:419-25. [PubMed]
- Noy R, Pollard JW. Tumor-associated macrophages: from mechanisms to therapy. Immunity 2014;41:49-61. [PubMed]
- Fridman WH, Pagès F, Sautès-Fridman C, et al. The immune contexture in human tumours: impact on clinical outcome. Nat Rev Cancer 2012;12:298-306. [PubMed]
- Galon J, Costes A, Sanchez-Cabo F, et al. Type, density, and location of immune cells within human colorectal tumors predict clinical outcome. Science 2006;313:1960-4. [PubMed]
- Galon J, Mlecnik B, Bindea G, et al. Towards the introduction of the 'Immunoscore' in the classification of malignant tumours. J Pathol 2014;232:199-209. [PubMed]
- Garon EB, Rizvi NA, Hui R, et al. Pembrolizumab for the Treatment of Non-Small-Cell Lung Cancer. N Engl J Med 2015;372:2018-28. [PubMed]
- Robert C, Schachter J, Long GV, et al. Pembrolizumab versus Ipilimumab in Advanced Melanoma. N Engl J Med 2015. [Epub ahead of print]. [PubMed]
- Motzer RJ, Rini BI, McDermott DF, et al. Nivolumab for Metastatic Renal Cell Carcinoma: Results of a Randomized Phase II Trial. J Clin Oncol 2015;33:1430-7. [PubMed]
- Hanahan D, Weinberg RA. Hallmarks of cancer: the next generation. Cell 2011;144:646-74. [PubMed]
- Roh-Johnson M, Bravo-Cordero JJ, Patsialou A, et al. Macrophage contact induces RhoA GTPase signaling to trigger tumor cell intravasation. Oncogene 2014;33:4203-12. [PubMed]
- Pignatelli J, Goswami S, Jones JG, et al. Invasive breast carcinoma cells from patients exhibit MenaINV- and macrophage-dependent transendothelial migration. Sci Signal 2014;7:ra112. [PubMed]
- Lin EY, Pollard JW. Macrophages: modulators of breast cancer progression. Novartis Found Symp 2004;256:158-68; discussion 68-72, 259-69.
- Lu W, Su J, Kim LS, et al. Active specific immunotherapy against occult brain metastasis. Cancer Res 2003;63:1345-50. [PubMed]
- Hinton CV, Avraham S, Avraham HK. Role of the CXCR4/CXCL12 signaling axis in breast cancer metastasis to the brain. Clin Exp Metastasis 2010;27:97-105. [PubMed]
- Lee BC, Lee TH, Avraham S, et al. Involvement of the chemokine receptor CXCR4 and its ligand stromal cell-derived factor 1alpha in breast cancer cell migration through human brain microvascular endothelial cells. Mol Cancer Res 2004;2:327-38. [PubMed]
- Hamilton A, Sibson NR. Role of the systemic immune system in brain metastasis. Mol Cell Neurosci 2013;53:42-51. [PubMed]
- Mareel M, Madani I. Tumour-associated host cells participating at invasion and metastasis: targets for therapy? Acta Chir Belg 2006;106:635-40. [PubMed]
- Liu Y, Kosaka A, Ikeura M, et al. Premetastatic soil and prevention of breast cancer brain metastasis. Neuro Oncol 2013;15:891-903. [PubMed]
- Chuang HN, Lohaus R, Hanisch UK, et al. Coculture system with an organotypic brain slice and 3D spheroid of carcinoma cells. J Vis Exp 2013;e50881. [PubMed]
- Qian B, Deng Y, Im JH, et al. A distinct macrophage population mediates metastatic breast cancer cell extravasation, establishment and growth. PLoS One 2009;4:e6562. [PubMed]
- Lorger M, Felding-Habermann B. Capturing changes in the brain microenvironment during initial steps of breast cancer brain metastasis. Am J Pathol 2010;176:2958-71. [PubMed]
- Du R, Lu KV, Petritsch C, et al. HIF1alpha induces the recruitment of bone marrow-derived vascular modulatory cells to regulate tumor angiogenesis and invasion. Cancer Cell 2008;13:206-20. [PubMed]
- Kienast Y, von Baumgarten L, Fuhrmann M, et al. Real-time imaging reveals the single steps of brain metastasis formation. Nat Med 2010;16:116-22. [PubMed]
- Adeegbe DO, Nishikawa H. Natural and induced T regulatory cells in cancer. Front Immunol 2013;4:190. [PubMed]
- Graeber MB, Streit WJ. Microglia: biology and pathology. Acta Neuropathol 2010;119:89-105. [PubMed]
- Osswald M, Winkler F. Insights into cell-to-cell and cell-to-blood-vessel communications in the brain: in vivo multiphoton microscopy. Cell Tissue Res 2013;352:149-59. [PubMed]
- Berghoff AS, Lassmann H, Preusser M, et al. Characterization of the inflammatory response to solid cancer metastases in the human brain. Clin Exp Metastasis 2013;30:69-81. [PubMed]
- Ojalvo LS, Whittaker CA, Condeelis JS, et al. Gene expression analysis of macrophages that facilitate tumor invasion supports a role for Wnt-signaling in mediating their activity in primary mammary tumors. J Immunol 2010;184:702-12. [PubMed]
- Sidani M, Wyckoff J, Xue C, et al. Probing the microenvironment of mammary tumors using multiphoton microscopy. J Mammary Gland Biol Neoplasia 2006;11:151-63. [PubMed]
- Berghoff AS, Kiesel B, Widhalm G, et al. Programmed death ligand 1 expression and tumor-infiltrating lymphocytes in glioblastoma. Neuro Oncol 2014. [Epub ahead of print]. [PubMed]
- Pukrop T, Dehghani F, Chuang HN, et al. Microglia promote colonization of brain tissue by breast cancer cells in a Wnt-dependent way. Glia 2010;58:1477-89. [PubMed]
- Hiratsuka S, Watanabe A, Aburatani H, et al. Tumour-mediated upregulation of chemoattractants and recruitment of myeloid cells predetermines lung metastasis. Nat Cell Biol 2006;8:1369-75. [PubMed]
- Tian T, Gu X, Zhang B, et al. Increased circulating CD14(+)HLA-DR-/low myeloid-derived suppressor cells are associated with poor prognosis in patients with small-cell lung cancer. Cancer Biomark 2015. [Epub ahead of print]. [PubMed]
- Platten M, Ochs K, Lemke D, et al. Microenvironmental clues for glioma immunotherapy. Curr Neurol Neurosci Rep 2014;14:440. [PubMed]
- Berghoff AS, Fuchs E, Ricken G, et al. Density of tumor-infiltrating lymphocytes correlates with extent of brain edema and overall survival time in patients with brain metastases. Oncoimmunol 2015;
- Adams S, Gray RJ, Demaria S, et al. Prognostic Value of Tumor-Infiltrating Lymphocytes in Triple-Negative Breast Cancers From Two Phase III Randomized Adjuvant Breast Cancer Trials: ECOG 2197 and ECOG 1199. J Clin Oncol 2014;32:2959-66. [PubMed]
- Hamanishi J, Mandai M, Iwasaki M, et al. Programmed cell death 1 ligand 1 and tumor-infiltrating CD8+ T lymphocytes are prognostic factors of human ovarian cancer. Proc Natl Acad Sci U S A 2007;104:3360-5. [PubMed]
- Takenaka M, Seki N, Toh U, et al. FOXP3 expression in tumor cells and tumor-infiltrating lymphocytes is associated with breast cancer prognosis. Mol Clin Oncol 2013;1:625-32. [PubMed]
- Tao H, Mimura Y, Aoe K, et al. Prognostic potential of FOXP3 expression in non-small cell lung cancer cells combined with tumor-infiltrating regulatory T cells. Lung Cancer 2012;75:95-101. [PubMed]
- Pugh SA, Harrison RJ, Primrose JN, et al. T cells but not NK cells are associated with a favourable outcome for resected colorectal liver metastases. BMC Cancer 2014;14:180. [PubMed]
- Remark R, Alifano M, Cremer I, et al. Characteristics and clinical impacts of the immune environments in colorectal and renal cell carcinoma lung metastases: influence of tumor origin. Clin Cancer Res 2013;19:4079-91. [PubMed]
- Cimino-Mathews A, Ye X, Meeker A, et al. Metastatic triple-negative breast cancers at first relapse have fewer tumor-infiltrating lymphocytes than their matched primary breast tumors: a pilot study. Hum Pathol 2013;44:2055-63. [PubMed]
- DiLillo DJ, Yanaba K, Tedder TF. B cells are required for optimal CD4+ and CD8+ T cell tumor immunity: therapeutic B cell depletion enhances B16 melanoma growth in mice. J Immunol 2010;184:4006-16. [PubMed]
- Berghoff AS, Ricken G, Widhalm G, et al. Tumor infiltrating lymphocytes and expression of programmed death ligand 1 (PD-L1) in melanoma brain metastases. Histopathology 2015;66:289-99. [PubMed]
- Robert C, Long GV, Brady B, et al. Nivolumab in previously untreated melanoma without BRAF mutation. N Engl J Med 2015;372:320-30. [PubMed]
- Rizvi NA, Mazieres J, Planchard D, et al. Activity and safety of nivolumab, an anti-PD-1 immune checkpoint inhibitor, for patients with advanced, refractory squamous non-small-cell lung cancer (CheckMate 063): a phase 2, single-arm trial. Lancet Oncol 2015;16:257-65. [PubMed]
- Naidoo J, Page DB, Wolchok JD. Immune modulation for cancer therapy. Br J Cancer 2014;111:2214-9. [PubMed]
- Berghoff AS, Bartsch R, Wöhrer A, et al. Predictive molecular markers in metastases to the central nervous system: recent advances and future avenues. Acta Neuropathol 2014;128:879-91. [PubMed]
- Ji RR, Chasalow SD, Wang L, et al. An immune-active tumor microenvironment favors clinical response to ipilimumab. Cancer Immunol Immunother 2012;61:1019-31. [PubMed]
- Berghoff AS, Sax C, Klein M, et al. Alleviation of brain edema and restoration of functional independence by bevacizumab in brain-metastatic breast cancer: a case report. Breast Care (Basel) 2014;9:134-6. [PubMed]
- Topalian SL, Hodi FS, Brahmer JR, et al. Safety, activity, and immune correlates of anti-PD-1 antibody in cancer. N Engl J Med 2012;366:2443-54. [PubMed]
- Berghoff AS, Inan C, Ricken G, et al. Tumor-infiltrating lymphocytes (TILs) and PD-L1 expression in non-small cell lung cancer brain metastases (BM) and matched primary tumors (PT). Ann Oncol 2014;25:465-6.
- Margolin K, Ernstoff MS, Hamid O, et al. Ipilimumab in patients with melanoma and brain metastases: an open-label, phase 2 trial. Lancet Oncol 2012;13:459-65. [PubMed]
- Hodi FS, O'Day SJ, McDermott DF, et al. Improved survival with ipilimumab in patients with metastatic melanoma. N Engl J Med 2010;363:711-23. [PubMed]
- Harmankaya K, Erasim C, Koelblinger C, et al. Continuous systemic corticosteroids do not affect the ongoing regression of metastatic melanoma for more than two years following ipilimumab therapy. Med Oncol 2011;28:1140-4. [PubMed]
- Chasset F, Pages C, Biard L, et al. Single-center study under a French Temporary Authorization for Use (TAU) protocol for ipilimumab in metastatic melanoma: negative impact of baseline corticosteroids. Eur J Dermatol 2015;25:36-44. [PubMed]
- Wolchok JD, Hoos A, O'Day S, et al. Guidelines for the evaluation of immune therapy activity in solid tumors: immune-related response criteria. Clin Cancer Res 2009;15:7412-20. [PubMed]
- Kiess AP, Wolchok JD, Barker CA, et al. Stereotactic Radiosurgery for Melanoma Brain Metastases in Patients Receiving Ipilimumab: Safety Profile and Efficacy of Combined Treatment. Int J Radiat Oncol Biol Phys 2015;92:368-75. [PubMed]
- Grimaldi AM, Simeone E, Giannarelli D, et al. Abscopal effects of radiotherapy on advanced melanoma patients who progressed after ipilimumab immunotherapy. Oncoimmunology 2014;3:e28780. [PubMed]