Targeted therapies in advanced biliary tract cancers—a narrative review
Introduction
Intrahepatic cholangiocarcinoma (IC), distal extrahepatic cholangiocarcinoma (EC), hilar (Klatskin) cholangiocarcinoma, and gallbladder cancer, collectively known as biliary tract cancers (BTCs) arise from the epithelium of the biliary tree and are relatively rare cancers. The global incidence of BTCs is estimated to be between 0.3 to 6 per 100,000 people, with the highest incidence in southeast Asia (age-standardized rate as high as 3.00 in South Korea) and lowest in Western countries (age-standardized rate as low as 0.66 in the United Kingdom) (1,2). In the United States, the incidence of cholangiocarcinoma, particularly IC, is rising (3,4). Risk factors for BTCs include liver fluke infection, primary sclerosing cholangitis, hepatolithiasis, choledochal cysts, alcohol consumption, tobacco use, hepatitis B and hepatitis C infection, and metabolic conditions such as obesity, diabetes, and nonalcoholic fatty liver disease (5-9). Although some progress has been made with respect to improving the survival of patients with BTCs over the past decade, historically, these malignancies are associated with a poor prognosis, with 5-year overall survival (OS) rates of less than 15% (10-12).
Early detection of BTCs greatly impacts prognosis. Currently, no screening guidelines exist or are recommended for BTCs. When BTC is suspected, contrast-enhanced magnetic resonance cholangiopancreatography (MRCP) or computed tomography (CT) are the imaging modalities of choice to evaluate for vascular anomalies, satellite lesions, lymph node involvement, and metastatic disease (13,14). These characteristics are crucial in determining whether a biliary tract tumor is surgically resectable and thereby potentially curable. A tissue diagnosis is often obtained via endoscopic retrograde cholangiopancreatography (ERCP) and via biliary tract brushings. Resectable disease is defined by the absence of multifocal liver disease, lymph node metastasis beyond the porta hepatis, and distant metastasis. Resectability status is ultimately determined by a multidisciplinary team of experienced radiologists and surgeons. In patient’s with hilar cholangiocarcinoma, biopsy should not be performed until resectability status and transplant candidacy have been determined because transperitoneal biopsy may preclude transplantation depending on institutions’ protocols. In patients with unresectable disease, biopsies should be performed for tissue analysis and molecular profiling, including next-generation sequencing, to identify actionable mutations. In addition, positron emission tomography (PET) and diagnostic laparoscopy can help detect regional lymph node involvement and distant metastasis, although PET scans have a higher propensity for falsely detecting sites of disease that are not actually sites of metastasis (15-17).
While chemotherapy has historically been the mainstay of treatment for patients with advanced cholangiocarcinoma, targeted molecular therapies are increasingly utilized in clinical practice due to newly identified alterations and the desire to reduce adverse effects associated with cytotoxic therapy (Figure 1). One analysis postulated that approximately 68% of patients with BTCs have an actionable mutation (18). In this review, we examine the evidence supporting the utilization of targeted therapies for patients with advanced BTCs. We present the following article in accordance with the Narrative Review reporting checklist (available at https://cco.amegroups.com/article/view/10.21037/cco-22-93/rc).
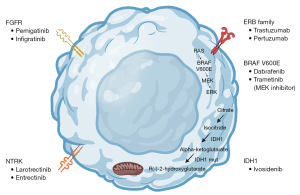
Methods
A literature search was performed to identify phase I, II, and III trials of targeted therapies in patients with advanced BTCs published between January 1, 2010 and October 1, 2022 (Table 1). MEDLINE (via PubMed) and ClinicalTrials.gov were searched for relevant studies and 415 trials were identified. The search strategy was performed using keywords including: biliary tract cancer, cholangiocarcinoma, gallbladder cancer, chemotherapy, targeted therapy, randomized trials, controlled trials, phase I, phase II, and phase III. Search results were imported into EndNote X 9.1.
Table 1
Items | Specification |
---|---|
Date of search | 06/01/2022 |
Databases and other sources searched | PubMed, ClinicalTrials.gov |
Search terms used | Biliary tract cancer, cholangiocarcinoma, gallbladder cancer, chemotherapy, targeted therapy, randomized trials, controlled trials, phase I, phase II, phase III |
Timeframe | January 1, 2010 and October 1, 2022 |
Inclusion and exclusion criteria | All clinical trials (phase I, phase II, phase III) for the treatment of biliary tract cancers were included, based on search criteria above. Trials were excluded if they were not completed, closed early, or did not report their outcome in the form of an abstract or manuscript |
Selection process | An independent search was conducted by the authors |
Chemotherapy
For patients with unresectable (hereto referred to as advanced) cholangiocarcinoma who are treatment naive, therapeutic options include clinical trial enrollment, gemcitabine-based chemotherapy, and chemotherapy combined with immunotherapy. Other options are also acceptable depending on a patient’s comorbidities and drug toxicity profiles, including fluoropyrimidine-based chemotherapy, chemoradiation, or radiation alone. The survival benefit conferred by gemcitabine or fluoropyrimidine-based chemotherapy for patients with advanced, BTCs has been demonstrated in several trials (Table 2). Based on the ABC-02 study, combination gemcitabine and cisplatin for patients with advanced, untreated BTC was the standard of care regimen for over a decade (19). Alternative first-line chemotherapeutic options that are suitable depending on a patient’s comorbidities include: gemcitabine plus oxaliplatin, gemcitabine plus capecitabine, gemcitabine plus albumin-bound paclitaxel, capecitabine plus oxaliplatin, 5-fluorouracil plus oxaliplatin, single-agent fluorouracil, single-agent capecitabine, or single-agent gemcitabine.
Table 2
Investigational arm | Comparison arm | N | Line | Phase | mPFS | mOS |
---|---|---|---|---|---|---|
Gemcitabine plus cisplatin, ABC-02 Study (19) | Gemcitabine | 410 | 1L | 3 | 8.0 vs. 5.0 months (P<0.001) | 11.7 vs. 8.1 months (HR 0.64; 95% CI: 0.52, 0.80; P<0.001) |
FOLFOX plus active symptom control, ABC-06 Study (20) | Active symptom control | 162 | 2L | 3 | 4.0 months (95% CI: 3.2–5.0) vs. not reported | 6.2 vs. 5.3 months (HR 0.69; 95% CI: 0.50, 0.97; P=0.031) |
Liposomal Irinotecan (nal-IRI) plus 5FU/LV, NIFTY Study (21) | 5FU/LV | 174 | 2L | 2B | 7.1 vs. 1.4 months (P=0.0019) | 8.6 vs. 5.3 months (HR 0.68; 95% CI: 0.48, 0.95; P=0.024) |
mPFS, median progression-free survival; mOS, median overall survival; CI, confidence interval; HR, hazard ratio; nal-IRI, nanoliposomal irinotecan; 5FU, fluorouracil; LV, leucovorin.
No consensus exists on which chemotherapy regimens should be utilized in the second-line (or further) setting, however the ABC-06 study demonstrated in patients with advanced, refractory BTCs, that 5-fluorouracil with leucovorin and oxaliplatin (FOLFOX) plus active symptom control improved median OS compared to active symptom control alone [6.2 vs. 5.3 months, hazard radio (HR) 0.69; 95% confidence interval (CI): 0.50–0.97; P=0.031] (20). Another option for patients with advanced BTCs who have progressed on gemcitabine and cisplatin is liposomal irinotecan in combination with fluorouracil, which was shown to be superior with regard to median OS compared to fluorouracil alone in the phase II NIFTY trial (8.6 vs. 5.3 months, HR 0.68; 95% CI: 0.48–0.95; P=0.024) (21).
Immunotherapy
Broadly, immunotherapy refers to the class of agents that block the ability of tumor cells to evade recognition and destruction by immune cells. Several prognostic biomarkers of response to immunotherapy for specific cancer types have been identified. However, their significance in predicting response to immunotherapy in BTCs is under investigation (22). A tumor’s mutational burden (TMB), or the quantity of a tumor’s genetic mutations, is associated with susceptibility to immunotherapy across many cancer types (23). High tumoral expression of PD-L1 is another marker that may be associated with susceptibility to immunotherapy (24). TMB status and PD-L1 expression do not typically correlate with one another, as each provides unique information about a tumor’s genome (25). A third prognostic biomarker for immunotherapy sensitivity involves mutations in genes that encode proteins responsible for the repair of single base pair insertions and deletions (mismatch repair, or MMR) at repetitive sequences of deoxyribonucleic acid (DNA), known as microsatellites. Tumoral genomes are classified as having either proficient (pMMR) or deficient MMR (dMMR) as well as microsatellite stability (MSS) or instability (MSI). In one analysis of mutational load by cancer type, 7% of all cholangiocarcinomas (n=1,327) had a TMB of greater than ten mutations per megabase, whereas only 1% of cholangiocarcinomas were classified as having microsatellite instability (26).
Recently, in the TOPAZ-1 study, the addition of durvalumab, an antibody against programmed death-ligand 1 (PD-L1), in combination with gemcitabine and cisplatin was shown to improve OS and progression-free survival (PFS) without an increase in grade 3 or 4 treatment-related adverse events in patients with advanced, BTCs (27). A median OS of 12.8 months was observed in patients who received durvalumab with gemcitabine and cisplatin compared to 11.5 months in patients who received gemcitabine and cisplatin (HR 0.80; 95% CI: 0.66–0.97; P=0.021) (Table 3).
Table 3
Investigational arm | Comparison arm | N | Line | Phase | mPFS | mOS |
---|---|---|---|---|---|---|
Durvalumab plus gemcitabine plus cisplatin, TOPAZ-1 Study (27) | Gemcitabine plus cisplatin | 685 | 1L | 3 | 7.2 vs. 5.8 months (HR 0.75; 95% CI: 0.64–0.89; P=0.001) | 12.8 vs. 11.5 months (HR 0.80; 95% CI: 0.66–0.97; P=0.021) |
mPFS, median progression-free survival; mOS, median overall survival; CI, confidence interval; HR, hazard ratio.
Nivolumab, an antibody against programmed cell death 1 (PD-1) receptor, has been studied in patients with advanced, refractory BTCs. In a phase II trial of 54 patients (46 evaluable from the United States) who received nivolumab, an objective response rate (ORR) of 22% was observed by investigator review (11% by blind independent review) and a disease control rate (DCR) of 59% was observed by investigator review (50% by blind independent review). All patients who responded had tumors with proficient mismatch repair, and nine of the ten patients who responded had PD-L1 expressed by 1% or more of the tumor cells analyzed (28). In another phase II trial, patients (n=39) who received nivolumab in combination with ipilimumab, an antibody against cytotoxic lymphocyte-associated protein 4 (CTLA-4), resulted in an ORR of 23% and DCR of 44%. Of note, only patients with IC or gallbladder cancer responded (29). As a result of these studies, nivolumab can be considered as a subsequent-line treatment option for patients with unresectable or metastatic progressive BTC who have not previously been treated with a checkpoint inhibitor.
Pembrolizumab, an antibody against PD-1, has also been studied in patients with advanced, refractory BTCs. In a phase II trial of 233 patients (with 27 cancer types, including 22 patients with cholangiocarcinoma) with MSI-H/dMMR tumors who received pembrolizumab, an ORR of 34.3%, median PFS of 4.1 months, and median OS of 23.5 months were observed. In addition, 29% of patients with a high TMB responded to pembrolizumab, whereas only 6% of patients without a high TMB had a response (30,31). In another analysis of 86 patients (with 12 cancer types, including four patients with cholangiocarcinoma) with dMMR tumors who received pembrolizumab, all patients with cholangiocarcinoma demonstrated disease control, and one had a complete response (32). As a result of these studies, pembrolizumab is another option as a first or subsequent-line (when a checkpoint inhibitor was not used in the first-line) treatment option for patients with advanced MSI-H/dMMR and TMB-H BTCs. Pembrolizumab was also studied in combination with lenvatinib, a multikinase inhibitor, in a phase II trial of 31 patients with advanced, refractory BTC. An ORR of 10%, DCR of 68%, and median PFS of 6.1 months were observed. All patients who responded had tumors with PD-L1 expressed by 1% or more of the tumor cells analyzed (33).
Dostarlimab, another antibody against PD-1, was studied in a phase I study of 209 patients with MSI-H/dMMR or polymerase epsilon catalytic subunit (POLE) hypermutated tumors (34). One patient in this study had gallbladder cancer, and another had cholangiocarcinoma. Both had a complete response (35). As a result of this study, dostarlimab can be considered as a subsequent-line treatment option for patients with MSI-H/dMMR recurrent or advanced BTCs as a subsequent-line therapy who have no satisfactory alternative treatment option and have not been treated with a checkpoint inhibitor.
Lastly, besides the aforementioned benefit from adding durvalumab to gemcitabine and cisplatin in the first-line setting from the TOPAZ-1 study, durvalumab has also been studied as a single agent and in combination with tremelimumab, an antibody against CTLA-4, in a phase I study of 107 patients with advanced, refractory BTCs. In the 42 patients who received durvalumab alone, a DCR of 16.7% and median OS of 8.1 months were observed. In the 65 patients who received durvalumab and tremelimumab, a DCR of 32.2% and median OS of 10.2 months were observed (36). Several trials studying the efficacy and safety of immunotherapy in patients with BTCs are ongoing (Table 4).
Table 4
Investigational arm | Comparison arm | n | Line | Phase | NCT number |
---|---|---|---|---|---|
Pembrolizumab plus gemcitabine plus cisplatin (KEYNOTE-966) | Gemcitabine plus cisplatin | 1,048 | 1L | 3 | NCT04003636 |
Durvalumab plus SNDX-6532 after intra-arterial chemoembolization or radioembolization | None | 30 | Unspecified | 2 | NCT04301778 |
Sitravatinib plus tislelizumab | None | 43 | 2L and beyond | 2 | NCT04727996 |
Durvalumab plus tremelimumab plus radiation | None | 70 | 2L and beyond | 2 | NCT03482102 |
Nivolumab plus rucaparib | None | 35 | Maintenance following platinum therapy | 2 | NCT03639935 |
Nivolumab plus DKN-01 | None | 30 | 2L and beyond | 2 | NCT04057365 |
Durvalumab plus tremelimumab with or without paclitaxel | None | 106 | 2L | 2 | NCT03704480 |
Nivolumab plus nanoliposomal-irinotecan plus 5-FU | None | 34 | 2L | 1/2 | NCT03785873 |
XmAb22841 | XmAb22841 with pembrolizumab | 242* | 2L and beyond | 1 | NCT03849469 |
Toripalimab plus lenvatinib | None | 44 | 2L | 2 | NCT04211168 |
Toripalimab plus S1 plus albumin paclitaxel | None | 30 | 1L | 2 | NCT04027764 |
Toripalimab plus gemcitabine plus oxaliplatin | none | 20 | 1L | 2 | NCT04191343 |
Avelumab plus regorafenib | None | 482* | 2L and beyond | 1/2 | NCT03475953 |
Camrelizumab plus apatinib | Camrelizumab plus either GEMOX or FOLFOX | 157* | Arm A: 2L or beyond; arm B: 1L | 2 | NCT03092895 |
Durvalumab plus guadecitabine | None | 55* | 2L | 1 | NCT03257761 |
Envafolimab plus GEMOX | GEMOX | 480 | 1L | 3 | NCT03478488 |
*, includes several cancer types. NCT, National Clinical Trial; FOLFOX, leucovorin (folinic acid), fluorouracil, oxaliplatin; GEMOX, gemcitabine, oxaliplatin.
Targeted therapies
Fibroblast growth factor receptor (FGFR) inhibitors
FGFRs are transmembrane receptors that, when bound by FGF ligands, activate downstream signaling pathways that promote cell survival, proliferation, and differentiation, including the extracellular signal-regulated kinase (ERK) and mitogen-activated protein kinase (MAPK) pathway (37). FGFR2 gene mutations, fusions, and rearrangements are identified in 13–14% of ICs and are associated with a favorable prognosis (38-41).
The FGFR inhibitor futibatinib, in the phase II FOENIX-CCA2 trial, was recently shown to show activity in 103 patients with advanced, refractory IC harboring FGFR2 fusion/rearrangements. The median duration of response was 9.5 months, PFS was 8.9 months, and OS was 20.0 months (42). Pemigatinib, another FGFR inhibitor, was studied in the phase II FIGHT-202 trial of 146 patients with advanced, refractory cholangiocarcinoma containing FGFR fusions, rearrangements, or alterations. 35.5% of patients achieved an objective response (95% CI: 26.5–45.4%) (43). Pemigatinib was also studied in another phase II trial of 87 patients with advanced, refractory cholangiocarcinoma (47 with FGFR2 translocations, 22 with other FGFR alterations, and 18 without FGFR alterations). Responses were only observed in eight patients with FGFR2 translocations. A median PFS of 6.8 months (95% CI: 3.6–9.2 months) in patients with FGFR2 translocations was observed compared to 1.4 months in patients with other FGFR alterations and 1.5 months in patients without FGFR alterations (44). Infigratinib, a different FGFR inhibitor, was studied in a phase II trial of 108 patients with advanced, refractory cholangiocarcinoma containing FGFR fusions or rearrangements. In this study, a median PFS of 7.3 months (95% CI: 5.6–7.6 months) and ORR of 23.1% (95% CI: 15.6–32.2%) were demonstrated, with a median duration of response of 5.0 months (45). As a result of these studies, pemigatinib and infigratinib are considered to be subsequent-line treatment options for patients with unresectable or metastatic cholangiocarcinoma with FGFR2 fusions or rearrangements.
Other FGFR inhibitors, such as derazantinib and RLY-4008, have been evaluated in early phase trials with modest to comparable results. Derazantinib was studied in a phase I/II trial of 29 patients with advanced IC with FGFR fusions, of which 27 patients had refractory disease. Patients treated with derazantinib had an ORR of 20.7%, DCR of 82.8%, and median PFS of 5.7 months (95% CI: 4.0–9.2 months) (46). Derazantinib was also studied in a phase II trial of 28 patients with IC containing FGFR2 mutations or amplifications. Of the 23 patients who received derazantinib, the DCR was 73.9% (95% CI: 51.6–89.8%) (47). RLY-4008 was studied in a phase I trial of 45 patients with advanced, refractory tumors (35 had cholangiocarcinoma) containing FGFR2 alterations (26 with FGFR fusions, 13 with FGFR mutations, and 5 with FGFR amplifications). Radiographic tumor reduction of 10% or more was observed in 59% of patients treated with RLY-4008 (48). RLY-4008 was also studied in a phase II trial of 38 patients advanced solid tumors with FGFR fusions or rearrangements and showed an ORR of 88% (at the “recommended phase 2 dose”) (49). Several trials studying the efficacy and safety of FGFR inhibitors in patients with advanced BTCs are ongoing (Table 5).
Table 5
Investigational arm | Comparison arm | N | Line | Phase | NCT number |
---|---|---|---|---|---|
Infigratinib | Gemcitabine plus cisplatin | 300 | 1L | 3 | NCT03773302 |
Infigratinib | None | 143 | 2L and beyond | 2 | NCT02150967 |
Pemigatinib | Gemcitabine plus cisplatin | 434 | 1L | 3 | NCT03656536 |
Futibatinib | Gemcitabine plus cisplatin | 216 | 1L | 3 | NCT04093362 |
Erdafitinib | None | 35* | 2L and beyond | 2 | NCT02699606 |
Gunagratinib | None | 56* | 2L and beyond | 1/2 | NCT03758664 |
Pazopanib plus trametinib | None | 89* | 2L and beyond | 1 | NCT01438554 |
Derazantinib | None | 148 | 2L and beyond | 2 | NCT03230318 |
E7090 | None | 60 | 2L and beyond | 2 | NCT04238715 |
*, includes several cancer types. NCT, National Clinical Trial; FGFR, fibroblast growth factor receptor.
Isocitrate dehydrogenase (IDH) inhibitors
IDH1 and IDH2 are enzymes involved in cellular aerobic respiration, catalyzing the conversion of isocitrate to alpha-ketoglutarate. Mutations in genes encoding these proteins result in the abnormal production of R(-)-2-hydroxyglutarate and downstream, uncontrolled cellular differentiation (50). IDH mutations exist in 10–23% of ICs and less than 1% of patients with ECs (38,51-53). In patients with EC, IDH1 mutations are associated with a poor prognosis (54).
Ivosidenib, an IDH1 inhibitor, was studied and compared to placebo in the phase III ClarIDHy trial of 185 patients with advanced, refractory IDH1-mutant cholangiocarcinoma. In the 124 patients treated with ivosidenib, a median PFS of 2.7 months was observed compared to 1.4 months in the placebo arm (HR 0.37; 95% CI: 0.25–0.52; P<0.0001) (55). In the final survival analysis, a median OS of 10.3 months in the ivosidenib arm was observed compared to 7.5 months in the placebo arm (HR 0.79; 95% CI: 0.34–0.70; P<0.0001) (56). As a result of this study, ivosidenib is recommended as a subsequent-line treatment option for patients with unresectable or metastatic IDH1-mutated cholangiocarcinoma. Several early-phase trials studying the efficacy and safety of therapies in patients with IDH-mutated BTCs are ongoing (Table 6).
Table 6
Investigational arm | Comparison arm | N | Line | Phase | NCT number |
---|---|---|---|---|---|
Olaparib | None | 145* | 2L and beyond | 2 | NCT03212274 |
Olaparib plus durvalumab | None | 78* | 2L or 3L | 2 | NCT03991832 |
Olaparib plus ceralasertib | None | 50* | 2L and beyond | 2 | NCT03878095 |
LY3410738 | LY3410738 with gemcitabine plus cisplatin or with durvalumab | 200* | Dependent on cohort (e.g., dose expansion cohort limited to 1L) | 2 | NCT04521686 |
HMPL-306 | None | 90* | 2L and beyond | 1 | NCT04762602 |
Dasatinib | None | 8 | 2L and beyond | 2 | NCT02428855 |
FT-2102 plus gemcitabine plus cisplatin | None | 93* | 2L | 1/2 | NCT03684811 |
Ivosidenib plus nivolumab | None | 35* | 2L and beyond | 2 | NCT04056910 |
Ivosidenib or pemigatinib plus gemcitabine plus cisplatin | None | 40 | Maintenance | 1 | NCT04088188 |
*, includes several cancer types. NCT, National Clinical Trial; IDH, isocitrate dehydrogenase.
Human epidermal growth factor receptor 2 (HER2)-directed therapy
HER2 is a receptor encoded by ERBB2 and belongs to the epidermal growth factor receptor (EGFR) family. Overexpression of HER2 leads to abnormal cell survival and proliferation (57). Typically, HER2 status is graded by immunohistochemical (IHC) staining from 0 (negative, or less than 10% of cells displaying membranous reactivity) to 3+ (positive). In situ hybridization (ISH) is used for IHC equivocal (2+) HER2 status to determine the ratio of copies of the HER2 gene to chromosome 17 centromeres (CEP17) within the nucleus of tumor cells. HER2 gene amplification is identified in as high as 18% of EC and is associated with a poor prognosis (58).
One of the first indicators of efficacy of HER2-directed therapy in patients with BTC was a retrospective study of eight patients with advanced gallbladder cancer containing HER2/neu gene amplification who received HER2-directed treatment. Three patients experienced disease stability, four experienced a partial response, and one had a complete response (59).
Neratinib, an oral pan-HER inhibitor, was studied in the phase II basket trial SUMMIT and showed modest response in patients with advanced, refractory HER2-mutated BTC. Of 25 patients in the updated analysis (11 cholangiocarcinoma, 10 gallbladder, 4 ampullary cancers), the ORR was 16% (95% CI: 4.5–36.1%), median PFS was 2.8 months (95% CI: 1.1–3.7 months), and median OS was 5.4 months (95% CI: 3.7–11.7 months) (60,61).
Varlitinib, another oral pan-HER inhibitor, was studied in a phase II trial of 127 patients with advanced, refractory BTCs. Sixty-four patients received varlitinib plus capecitabine and 63 received placebo plus capecitabine. No differences among the two arms were detected with regard to median PFS (2.8 vs. 2.8 months; P=0.63) and median OS (7.8 vs. 7.5 months; P=0.66) (62).
Regarding HER2-directed antibodies, the combination of pertuzumab and trastuzumab, both monoclonal antibodies against HER2, was studied in a phase II trial of 39 patients with HER2-amplified or HER2-overexpressed (or both) advanced, refractory BTC. This study revealed that dual HER2-directed therapy resulted in an ORR of 23% (95% CI: 11–39%). Notably, patients with gallbladder cancer had an ORR of 31% (95% CI: 11–59%) and DCR of 63% (95% CI: 35–85%), which was higher than the ORR in patients with intrahepatic and EC (63).
Moreover, trastuzumab-deruxtecan, an antibody-drug conjugate, was studied in a phase II trial of 32 patients with advanced, refractory BTCs; 24 patients were HER2-expressing and 8 patients were classified as having HER2-low disease. HER2-expressing was defined as IHC/ISH status of 3+ or 2+/+ while HER2-low was defined as IHC/ISH status of 0/+, 1+/−, 1+/+, or 2+/−. In HER2-expressing patients, an ORR of 36.4% (90% CI: 19.6–56.1%), DCR of 81.8% (95% CI: 59.7–94.8%), median PFS of 4.4 months (95% CI: 2.8–8.3 months), and median OS of 7.1 months (95% CI: 4.7–14.6 months) were observed compared to an ORR of 12.5% (95% CI: 0.3–52.7%), DCR of 75.0% (95% CI: 34.9–96.8%), median PFS of 4.2 months (95% CI: 1.3–6.2 months), and median OS of 8.9 months (95% CI: 3.0–12.8 months) in HER2-low patients (64).
Lastly, zanidatamab, a bispecific HER2-targeted antibody, was studied in a phase I trial of 20 patients with advanced, refractory BTCs with HER2 overexpression. Of 17 evaluable patients, an ORR of 47% (95% CI: 23–72%), DCR of 65% (95% CI: 38–86%), and median duration of response of 6.6 months (95% CI: 3.2–not estimable) were observed (65). Several trials studying the efficacy and safety of HER2-directed therapies in patients with advanced BTCs are ongoing (Table 7).
Table 7
Investigational arm | Comparison arm | N | Line | Phase | NCT number |
---|---|---|---|---|---|
Trastuzumab plus tucatinib | None | 12 | 2L and beyond | 2 | NCT04579380 |
Multiple targeted therapies | None | 6,452* | 2L and beyond | 2 | NCT02465060 |
Multiple targeted therapies | None | 3,581* | 2L and beyond | 2 | NCT02693535 |
BDTX-189 | None | 91* | 2L and beyond | 1/2 | NCT04209465 |
*, includes several cancer types. NCT, National Clinical Trial; HER2, human epidermal growth factor receptor 2.
Neurotrophic tyrosine receptor kinase (NTRK) gene fusion inhibitors
NTRK genes encode transmembrane tyrosine kinase receptors that, when bound by neurotrophins, activate downstream signaling pathways promoting cell growth, differentiation, and survival such as MAPK, phosphatidylinositol 3-kinase (PI3K), and protein kinase C (PKC). Molecular aberrations that commonly affect NTRK, including gene fusions, mutations, and amplifications, result in constitutive activation of these signaling pathways (66). NTRK fusions exist in less than 1% of BTCs (67).
Both entrectinib and larotrectinib have shown activity in the very rare setting of BTCs with NTRK fusions. Entrectinib, a multikinase inhibitor that targets oncogenic rearrangements in genes such as NTRK, c-ros oncogene 1 (ROS1), and anaplastic lymphoma kinase (ALK), was studied in three early-phase trials of patients with advanced or metastatic NTRK fusion-positive tumors. In a pooled efficacy analysis of 54 patients enrolled in these trials, 57% had an objective response; however, only one patient in this analysis had cholangiocarcinoma (68). Larotrectinib, a tropomyosin receptor kinase (TRK) inhibitor, was studied in an early-phase trial of 55 patients with TRK fusion-positive tumors, in which two patients had cholangiocarcinoma. 75% achieved an objective response (95% CI: 61–85%). At one year, 71% of the responses were ongoing, and 55% of patients did not have disease progression (69). Entrectinib and larotrectinib are therefore viable first or subsequent-line treatment options for patients with unresectable or metastatic cholangiocarcinoma with NTRK gene fusions.
B-Raf proto-oncogene (BRAF) inhibitors
BRAF kinase is a protein that regulates signaling networks, primarily the MAPK/ERK cascade, responsible for regulating cell growth, development, and division (70). BRAF V600E mutations are identified in less than 1% of BTCs and are more common in IC (71).
Dabrafenib, a BRAF inhibitor, combined with trametinib, a mitogen-activated extracellular signal regulated kinase (MEK) 1/2 inhibitor, was studied in a phase II trial of 43 patients with BRAF V600E-mutated advanced, refractory BTC. 51% of patients treated with dabrafenib and trametinib achieved a response (95% CI: 36–37%) (72). In an open-label, single-arm study of 35 patients with advanced, refractory BRAF V600E-mutated tumors, four of which had cholangiocarcinoma, the ORR was 38% (90% CI: 22.9–54.9%) in patients treated with dabrafenib and trametinib. Three of the four patients with cholangiocarcinoma demonstrated a partial response, with individual PFS of 29.4, 12.8, and 9.1 months (73). Accordingly, combination therapy with dabrafenib and trametinib is a subsequent-line treatment option in unresectable or metastatic cholangiocarcinoma with BRAF V600E mutations.
EGFR inhibitors
EGFRs are transmembrane receptors that transduce signals through the MAPK, PI3K-Protein kinase B (PBK, or Akt), and phospholipase C (PLC)/protein kinase C (PKC) signaling pathways upon ligand binding that stimulate cell proliferation, differentiation, growth, and migration (74). Overexpression of EGFR is thought to be present in 8–27% of BTCs and is more common in IC (75,76); however, randomized studies have shown no benefit when adding EGFR inhibitors to a chemotherapy backbone.
Cetuximab, an EGFR inhibitor, was studied in combination with gemcitabine plus oxaliplatin in a phase II trial of 30 patients with advanced, untreated BTC. An objective response occurred in 63% of patients (95% CI: 56.2–69.8%) (77). This study led to further evaluation of cetuximab in the randomized phase II BINGO trial of 150 patients with advanced BTC, in which 76 patients received cetuximab in combination with gemcitabine plus oxaliplatin and 74 received gemcitabine and oxaliplatin alone. Overall, this study demonstrated no significant survival differences between study arms (78).
Panitumumab, another EGFR inhibitor, was studied in a phase II trial of 28 patients with advanced, untreated cholangiocarcinoma. This combination of therapies led to a DCR of 74%, median PFS of 9.7 months (95% CI: 5.1–12.9 months), and median OS of 12.9 months (95% CI: 9.5–27.8 months) (79). The addition of panitumumab to chemotherapy was subsequently evaluated in the randomized, phase II Vecti-BIL trial of 89 patients with untreated, KRAS wild-type, advanced BTCs. Forty-five patients received panitumumab in combination with gemcitabine and oxaliplatin, and 44 received gemcitabine and oxaliplatin alone. Similar to the results from the BINGO study, no survival benefit was observed with the addition of panitumumab to chemotherapy. A subgroup analysis by disease site demonstrated that panitumumab with chemotherapy may have resulted in a marginal OS benefit compared to chemotherapy alone in patients with IC, although there was a higher incidence of EGFR-related toxicity in the combination arm (80).
Finally, erlotinib, an oral EGFR inhibitor, was studied in a phase II trial of 42 patients with advanced, refractory BTC. 81% of patients included for analysis had EGFR overexpression. Seventeen percent of patients treated with erlotinib monotherapy were progression-free at 6 months (95% CI: 7–31%) and 8% of patients achieved a response (95% CI: 2–20%) (81). Erlotinib was later studied in a phase III trial of 268 patients with advanced, untreated BTC in which 135 patients received erlotinib in combination with gemcitabine and oxaliplatin and 133 received gemcitabine and oxaliplatin alone. Again, there were no significant PFS or OS differences when adding erlotinib to chemotherapy, although a subgroup analysis by disease site demonstrated a possible PFS benefit in patients with cholangiocarcinoma (82).
Other novel therapies
A number of other therapies, including praseltinib, regorafenib, bintrafusp alfa, INCB001158, sotorasib, and chimeric antigen receptor (CAR) T cells have been studied, largely in the context of early phase trials, in patients with advanced BTCs.
Rearranged during transfection (RET) fusions and mutations occur in approximately 5.7% of cholangiocarcinoma (83). Pralsetinib, a RET inhibitor, was studied in an early-phase trial of 27 patients with advanced, refractory RET-altered solid tumors. Of the two patients with cholangiocarcinoma, both had an objective response (84). Regorafenib, a multikinase inhibitor, was studied in three phase II trials in patients with advanced, refractory BTCs. In these studies, median PFS was found to be 3.6 months (90% CI: 3.0–5.7 months), 3.7 months (95% CI: 1.8–5.4 months), and, 1.5 months (95% CI: 1.2–2.0 months) while median OS was found to be 3.6 months (90% CI: 3.1–17.1 months), 5.4 months (95% CI: 3.4–12.8 months), and 5.3 months (95% CI: 2.7–10.5 months), respectively (85). A bifunctional fusion protein against transforming growth factor-beta and PD-L1, called bintrafusp alfa, was studied in a phase I trial of 30 patients with advanced, refractory BTC. Treatment with bintrafusp alfa in this cohort resulted in an ORR of 20% (95% CI: 8.0–39.0%), median PFS of 2.5 months (95% CI: 1.3–5.6 months), and median OS of 12.7 months (95% CI: 6.1–15.7 months) (86). The arginase inhibitor INCB001158, in combination with gemcitabine plus cisplatin, was administered to 33 patients with advanced, untreated BTC in an early phase trial. Patients experienced an ORR of 24% (95% CI: 11.1–42.3%), median duration of response of 5.8 months (4.1 months–not yet reached), and median PFS of 8.5 months (5.7–10.1 months) (87). Sotorasib, an inhibitor of Kirsten rat sarcoma viral oncogene homolog (KRAS), was studied in a phase I trial of 25 patients with advanced, refractory solid tumors harboring KRAS p.G12C mutations, which included one patient who had BTC and who achieved disease control (88).
Finally, one patient achieved a complete response and ten patients experiences stable disease in a phase I study of 17 patients with advanced, refractory EGFR-positive BTCs treated with anti-EGFR CAR T cells. In this study, a median PFS of 4 months (range, 2.2–22 months) was observed (89). In another phase I trial of 11 patients with advanced, refractory HER2-positive BTC and pancreatic cancer treated with anti-HER2 CAR T cells, one patient achieved a partial response, and five patients experienced stable disease. Combinations of previously studied therapies and a number of new agents are being studied in patients with advanced BTCs (Table 8).
Table 8
Investigational arm (mechanism) | Comparison arm | n | Line | Phase | NCT number |
---|---|---|---|---|---|
Olaparib (PARP inhibitor) | None | 36 | Maintenance following platinum therapy | 2 | NCT04042831 |
Ramucirumab (monoclonal antibody against VEGFR2 | None | 61 | 2L and beyond | 2 | NCT02520141 |
Niraparib (PARP inhibitor) | None | 35* | 2L and beyond | 2 | NCT03207347 |
Anlotinib (multikinase inhibitor) and levamisole (T cell activation and proliferation) | Anlotinib | 152 | 2L and beyond | 3 | NCT03940378 |
Bortezomib (proteasome inhibitor) | None | 50 | 2L and beyond | 3 | NCT03345303 |
CB-103 (diaryl ether against Notch receptors) | None | 200* | 2L and beyond | 1/2 | NCT03422679 |
Opaganib (SK2 inhibitor) with or without hydroxychloroquine | Opaganib | 65 | 1L or 2L | 2 | NCT03377179 |
Apatinib (VEGFR2 inhibitor) | None | 55 | 2L and beyond | 2 | NCT03427242 |
Apatinib (VEGFR2 inhibitor) | None | 30 | 2L and beyond | 2 | NCT03521219 |
Surufatinib (pan-VEGFR and CSF-1R inhibitor) | Capecitabine | 298 | 2L | 2/3 | NCT03873532 |
Copanlisib (PI3K inhibitor) plus gemcitabine plus cisplatin | None | 24 | 1L (although patients who received adjuvant therapy more than 6 months prior are also eligible) | 2 | NCT02631590 |
RXC004 (porcupine inhibitor) plus denosumab | None | 15 | 2L | 2 | NCT04907851 |
Crizotinib (multikinase inhibitor) | None | 246* | 2L and beyond | 2 | NCT02034981 |
Natural killer cells plus pembrolizumab | None | 40 | 2L and beyond | NCT03937895 | |
Tumor infiltrating lymphocytes plus aldesleukin (recombinant interleukin-2) | None | 59 | 2L and beyond | 2 | NCT03801083 |
Memory T cells plus chemotherapy | Chemotherapy | 20 | Resection without recurrence | 2 | NCT03820310 |
MUC-1 CAR-T cells plus fludarabine plus cyclophosphamide | None | 9 | 1L | 1/2 | NCT03633773 |
Anti-HER2 CAR-macrophages | None | 18* | 2L and beyond | 1 | NCT04660929 |
Gavocabtagene autoleucel (cell therapy) plus fludarabine plus cyclophosphamide with or without nivolumab and ipilimumab | None | 175* | 2L and beyond (but not more than 5 lines) | 1/2 | NCT03907852 |
Oncolytic adenovirus encoding TMZ-CD40L and 4-1BBL plus gemcitabine plus cisplatin | None | 50* | 1L and beyond | 1/2 | NCT03225989 |
*, includes several cancer types. NCT, National Clinical Trial; VEGFR, vascular endothelial growth factor receptor; CSF-1, colony stimulating factor 1; PI3K, phosphoinositide 3-kinase; CAR, chimeric antigen receptor; HER2, human epidermal growth factor receptor 2; PARP, poly adenosine diphosphate-ribose polymerase.
Future directions
Molecular profiles of biliary tract tumors vary by anatomic location, with IC displaying a higher rate of actionable mutations than other BTCs (90-92). For example, FGFR2 fusions and mutations in genes such as breast cancer gene-associated protein 1 (BAP1), IDH1, and polybromo 1 (PBRM1) are more common in IC while HER2 and tumor protein p53 (TP53) mutations are more common in EC and gallbladder cancer (91,92). An emerging understanding of the importance of biliary tract tumor transcriptomics and proteomics has helped identify “clusters” of biliary tract tumors based on genetic aberrations and immunophenotypes, supporting the hypothesis that molecular alterations may be used in conjunction with RNA and protein expression when searching for new targets and potentially choosing a therapeutic regimen. In an analysis of genomic and transcriptomic records of over 400 patients in North America with matched DNA, ribonucleic acid (RNA), and clinical data, four distinct genomic clusters (grouped based on driver mutations in similar genes) were identified (Table 9) (93). This analysis showed actionable biomarkers (defined by the authors as TMB > ten mutations per megabase, MSI-H, dMMR, NTRK gene fusions, FGFR2 fusions or rearrangements, IDH1 mutations, HER2 alterations, or BRAF V600E mutations) were present in 30.5% of all BTCs—39.1% of IC, 29.6% of EC, and 15% of gallbladder cancer. Another analysis, using a combination of genomic and proteomic data from 110 patients with IC, identified three molecular subtypes (chromatin remodeling, metabolism, and chronic inflammation) and showed that clinical outcomes varied by subtype. Others have also identified similar clusters and subtypes of BTCs based on shared tumor microenvironmental profiles (94-98). These data support the use of tumor molecular profiling and potentially incorporating multi-omic information to determine first and subsequent-line therapies in all patients with BTCs.
Table 9
Cluster 1 | Cluster 2 | Cluster 3 | Cluster 4 |
---|---|---|---|
TP53, KRAS, HER2, and ATM mutations | CDKN2A/B mutations | IDH1 and chromatin remodeling gene (ARID1A and PBRM1) mutations | FGFR2 fusions and BAP1 mutations |
BAP1, BRCA1 associated protein-1; FGFR, fibroblast growth factor receptor; IDH1, isocitrate dehydrogenase 1; CDKN2A/B, cyclin-dependent kinase inhibitor 2A/B; HER2, human epidermal growth factor receptor 2; ARID1A, AT-rich interactive domain-containing protein 1A; PBRM1, polybromo 1; ATM, ataxia telangiectasia mutated.
To date, most targeted therapies developed for BTCs take advantage of DNA mutations identified in tumor tissue. Efforts to detect targets in blood, bile, and cytologic specimens (i.e., from brushings obtained on endoscopic procedures or small tissue samples obtained on fine-needle aspiration) are underway. Circulating tumor DNA (ctDNA) and circulating tumor cells (CTCs), collectively referred to as cell-free DNA (cfDNA), are released into peripheral blood via necrosis and apoptosis of tumor cells (99). cfDNA is sometimes utilized to identify targetable mutations when tissue cannot be obtained for technical or logistical reasons and is being studied as a mechanism to detect early disease, acquired resistance to therapy, and response to treatment (100). In BTCs, mutational concordance between tissue and cfDNA is between 50–100% and is higher for IC (compared to other types of BTCs) and tissue obtained from metastatic sites of disease (compared to the primary tumor) (101-104). In a large analysis of 2,068 samples of cfDNA from 1,671 patients with advanced BTCs, targetable mutations were detected in 44% of patients. In this analysis, concordance between cfDNA was highest for IDH1 mutations (87%) and BRAF V600E mutations (87%) but low for FGFR2 fusions (18%) (105). Biliary tract tumors also shed ctDNA into bile. The mutational concordance between bile ctDNA and tissue DNA is as high as 88% (106). Alterations in non-DNA markers, particularly non-coding RNA (including micro RNA) shed from tumors into blood and bile, have also been studied to detect early disease, prognosticate, and monitor response to therapy for patients with BTCs (107-110).
Conclusions
BTCs are a heterogeneous group of malignancies, both in terms of anatomic location and mutational profile, with a poor prognosis. The development and refinement of next-generation sequencing has led to the identification of numerous actionable targets over the past decade. The integration of multi-omic information will likely lead to the development of new therapies targeting genomic alterations. Targeted agents can improve survival in patients with advanced disease and have substantially increased the number of potential therapeutic options in patients with refractory or progressive disease. Moreover, with ongoing and rapid drug development, these drugs may be studied in the first-line setting for patients with advanced BTCs who have actionable mutations. Data should also be gathered on patients who progress on targeted therapies to better understand the development of acquired resistance and define optimal treatment sequencing. In addition, non-invasive techniques to detect early disease and monitor response to therapy, which represent a promising alternative to tissue-based target identification, will likely be studied in trials with potential to change clinical practice. In conclusion, the therapeutic landscape of BTCs continues to evolve, based on improvements in detection of genomic alterations and effective utilization of molecularly-driven therapies. Discovering novel agents for genomic alterations in BTCs will be key in the coming years in order to improve patient outcomes.
Acknowledgments
Funding: None.
Footnote
Reporting Checklist: The authors have completed the Narrative Review reporting checklist. Available at https://cco.amegroups.com/article/view/10.21037/cco-22-93/rc
Conflicts of Interest: All authors have completed the ICMJE uniform disclosure form (available at https://cco.amegroups.com/article/view/10.21037/cco-22-93/coif). RA serves as an unpaid editorial board member of Chinese Clinical Oncology from May 2022 to April 2024. TH received grant support for Young Investigator Award from Conquer Cancer, the ASCO Foundation, and National Institute of Health (Bethesda, MD, USA) (No. K12-CA090625-17). LG received research funding from BMS, Agios, ASLAN, BeiGene, Basilea and Merck; consulting fees from Athenum; and is on the board of QED, Genentech, Merck, AstraZeneca, Exelixis, Boehringer Ingelheim and Cardinal Health. RA received Career Development Grant (NIH/NCI K12CA090625); honoraria for speaking at 2021 ASCO Advantage Course, 2022 NCCN Annual Conference and 2022 Great Debates and Updates in GI Malignancies; support (food and beverage) from Ipsen Pharmaceuticals, Inc at the 2020 ASCO GI Symposium Meeting. ML has no conflicts of interest to declare.
Ethical Statement: The authors are accountable for all aspects of the work in ensuring that questions related to the accuracy or integrity of any part of the work are appropriately investigated and resolved.
Open Access Statement: This is an Open Access article distributed in accordance with the Creative Commons Attribution-NonCommercial-NoDerivs 4.0 International License (CC BY-NC-ND 4.0), which permits the non-commercial replication and distribution of the article with the strict proviso that no changes or edits are made and the original work is properly cited (including links to both the formal publication through the relevant DOI and the license). See: https://creativecommons.org/licenses/by-nc-nd/4.0/.
References
- Wang H, Sun P, Baria K. The world-wide incidence of biliary tract cancer (BTC). American Society of Clinical Oncology; 2020.
- Bertuccio P, Malvezzi M, Carioli G, et al. Global trends in mortality from intrahepatic and extrahepatic cholangiocarcinoma. J Hepatol 2019;71:104-14. [Crossref] [PubMed]
- Saha SK, Zhu AX, Fuchs CS, et al. Forty-Year Trends in Cholangiocarcinoma Incidence in the U.S.: Intrahepatic Disease on the Rise. Oncologist 2016;21:594-9. [Crossref] [PubMed]
- Mukkamalla SKR, Naseri HM, Kim BM, et al. Trends in Incidence and Factors Affecting Survival of Patients With Cholangiocarcinoma in the United States. J Natl Compr Canc Netw 2018;16:370-6. [Crossref] [PubMed]
- Prueksapanich P, Piyachaturawat P, Aumpansub P, et al. Liver Fluke-Associated Biliary Tract Cancer. Gut Liver 2018;12:236-45. [Crossref] [PubMed]
- Petrick JL, Yang B, Altekruse SF, et al. Risk factors for intrahepatic and extrahepatic cholangiocarcinoma in the United States: A population-based study in SEER-Medicare. PLoS One 2017;12:e0186643. [Crossref] [PubMed]
- Welzel TM, Graubard BI, El-Serag HB, et al. Risk factors for intrahepatic and extrahepatic cholangiocarcinoma in the United States: a population-based case-control study. Clin Gastroenterol Hepatol 2007;5:1221-8. [Crossref] [PubMed]
- Donato F, Gelatti U, Tagger A, et al. Intrahepatic cholangiocarcinoma and hepatitis C and B virus infection, alcohol intake, and hepatolithiasis: a case-control study in Italy. Cancer Causes Control 2001;12:959-64. [Crossref] [PubMed]
- Tyson GL, El-Serag HB. Risk factors for cholangiocarcinoma. Hepatology 2011;54:173-84. [Crossref] [PubMed]
- Anderson CD, Pinson CW, Berlin J, et al. Diagnosis and treatment of cholangiocarcinoma. Oncologist 2004;9:43-57. [Crossref] [PubMed]
- Howlader N. Seer cancer statistics review, 1975-2008, national cancer institute, bethesda, md. Available online: http://seer cancer gov/csr/1975_2008/. based on November 2010 SEER data submission, posted to the SEER web site. 2011.
- Park J, Kim MH, Kim KP, et al. Natural History and Prognostic Factors of Advanced Cholangiocarcinoma without Surgery, Chemotherapy, or Radiotherapy: A Large-Scale Observational Study. Gut Liver 2009;3:298-305. [Crossref] [PubMed]
- Vogl TJ, Schwarz WO, Heller M, et al. Staging of Klatskin tumours (hilar cholangiocarcinomas): comparison of MR cholangiography, MR imaging, and endoscopic retrograde cholangiography. Eur Radiol 2006;16:2317-25. [Crossref] [PubMed]
- Hyodo T, Kumano S, Kushihata F, et al. CT and MR cholangiography: advantages and pitfalls in perioperative evaluation of biliary tree. Br J Radiol 2012;85:887-96. [Crossref] [PubMed]
- Petrowsky H, Wildbrett P, Husarik DB, et al. Impact of integrated positron emission tomography and computed tomography on staging and management of gallbladder cancer and cholangiocarcinoma. J Hepatol 2006;45:43-50. [Crossref] [PubMed]
- Corvera CU, Blumgart LH, Akhurst T, et al. 18F-fluorodeoxyglucose positron emission tomography influences management decisions in patients with biliary cancer. J Am Coll Surg 2008;206:57-65. [Crossref] [PubMed]
- Lee SW, Kim HJ, Park JH, et al. Clinical usefulness of 18F-FDG PET-CT for patients with gallbladder cancer and cholangiocarcinoma. J Gastroenterol 2010;45:560-6. [Crossref] [PubMed]
- Simbolo M, Fassan M, Ruzzenente A, et al. Multigene mutational profiling of cholangiocarcinomas identifies actionable molecular subgroups. Oncotarget 2014;5:2839-52. [Crossref] [PubMed]
- Valle J, Wasan H, Palmer DH, et al. Cisplatin plus gemcitabine versus gemcitabine for biliary tract cancer. N Engl J Med 2010;362:1273-81. [Crossref] [PubMed]
- Lamarca A, Palmer DH, Wasan HS, et al. Second-line FOLFOX chemotherapy versus active symptom control for advanced biliary tract cancer (ABC-06): a phase 3, open-label, randomised, controlled trial. Lancet Oncol 2021;22:690-701. [Crossref] [PubMed]
- Yoo C, Kim KP, Jeong JH, et al. Liposomal irinotecan plus fluorouracil and leucovorin versus fluorouracil and leucovorin for metastatic biliary tract cancer after progression on gemcitabine plus cisplatin (NIFTY): a multicentre, open-label, randomised, phase 2b study. Lancet Oncol 2021;22:1560-72. [Crossref] [PubMed]
- Rizzo A, Ricci AD, Brandi G. PD-L1, TMB, MSI, and Other Predictors of Response to Immune Checkpoint Inhibitors in Biliary Tract Cancer. Cancers (Basel) 2021;13:558. [Crossref] [PubMed]
- Yarchoan M, Hopkins A, Jaffee EM. Tumor Mutational Burden and Response Rate to PD-1 Inhibition. N Engl J Med 2017;377:2500-1. [Crossref] [PubMed]
- Patel SP, Kurzrock R. PD-L1 Expression as a Predictive Biomarker in Cancer Immunotherapy. Mol Cancer Ther 2015;14:847-56. [Crossref] [PubMed]
- Yarchoan M, Albacker LA, Hopkins AC, et al. PD-L1 expression and tumor mutational burden are independent biomarkers in most cancers. JCI Insight 2019;4:e126908. [Crossref] [PubMed]
- Alexandrov LB, Nik-Zainal S, Wedge DC, et al. Signatures of mutational processes in human cancer. Nature 2013;500:415-21. [Crossref] [PubMed]
- Oh DY, Ruth He A, Qin S, et al. Durvalumab plus Gemcitabine and Cisplatin in Advanced Biliary Tract Cancer. NEJM Evidence 2022:EVIDoa2200015.
- Kim RD, Chung V, Alese OB, et al. A Phase 2 Multi-institutional Study of Nivolumab for Patients With Advanced Refractory Biliary Tract Cancer. JAMA Oncol 2020;6:888-94. [Crossref] [PubMed]
- Klein O, Kee D, Nagrial A, et al. Evaluation of Combination Nivolumab and Ipilimumab Immunotherapy in Patients With Advanced Biliary Tract Cancers: Subgroup Analysis of a Phase 2 Nonrandomized Clinical Trial. JAMA Oncol 2020;6:1405-9. [Crossref] [PubMed]
- Marabelle A, Le DT, Ascierto PA, et al. Efficacy of Pembrolizumab in Patients With Noncolorectal High Microsatellite Instability/Mismatch Repair-Deficient Cancer: Results From the Phase II KEYNOTE-158 Study. J Clin Oncol 2020;38:1-10. [Crossref] [PubMed]
- Marabelle A, Fakih M, Lopez J, et al. Association of tumour mutational burden with outcomes in patients with advanced solid tumours treated with pembrolizumab: prospective biomarker analysis of the multicohort, open-label, phase 2 KEYNOTE-158 study. Lancet Oncol 2020;21:1353-65. [Crossref] [PubMed]
- Le DT, Durham JN, Smith KN, et al. Mismatch repair deficiency predicts response of solid tumors to PD-1 blockade. Science 2017;357:409-13. [Crossref] [PubMed]
- Villanueva L, Lwin Z, Chung HC, et al. Lenvatinib plus pembrolizumab for patients with previously treated biliary tract cancers in the multicohort phase II LEAP-005 study. J Clin Oncol 2021;39:4080. [Crossref]
- Berton D, Banerjee SN, Curigliano G, et al. Antitumor activity of dostarlimab in patients with mismatch repair-deficient/microsatellite instability–high tumors: A combined analysis of two cohorts in the GARNET study. J Clin Oncol 2021;39:2564. [Crossref]
- Andre T, Berton D, Curigliano G, et al. Safety and efficacy of anti–PD-1 antibody dostarlimab in patients (pts) with mismatch repair-deficient (dMMR) solid cancers: Results from GARNET study. J Clin Oncol 2021;39: [Crossref]
- Ioka T, Ueno M, Oh DY, et al. Evaluation of safety and tolerability of durvalumab (D) with or without tremelimumab (T) in patients (pts) with biliary tract cancer (BTC). J Clin Oncol 2019;37: [Crossref]
- Krook MA, Reeser JW, Ernst G, et al. Fibroblast growth factor receptors in cancer: genetic alterations, diagnostics, therapeutic targets and mechanisms of resistance. Br J Cancer 2021;124:880-92. [Crossref] [PubMed]
- Churi CR, Shroff R, Wang Y, et al. Mutation profiling in cholangiocarcinoma: prognostic and therapeutic implications. PLoS One 2014;9:e115383. [Crossref] [PubMed]
- Ross JS, Wang K, Gay L, et al. New routes to targeted therapy of intrahepatic cholangiocarcinomas revealed by next-generation sequencing. Oncologist 2014;19:235-42. [Crossref] [PubMed]
- Graham RP, Barr Fritcher EG, Pestova E, et al. Fibroblast growth factor receptor 2 translocations in intrahepatic cholangiocarcinoma. Hum Pathol 2014;45:1630-8. [Crossref] [PubMed]
- Arai Y, Totoki Y, Hosoda F, et al. Fibroblast growth factor receptor 2 tyrosine kinase fusions define a unique molecular subtype of cholangiocarcinoma. Hepatology 2014;59:1427-34. [Crossref] [PubMed]
- Goyal L, Meric-Bernstam F, Hollebecque A, et al. Updated results of the FOENIX-CCA2 trial: Efficacy and safety of futibatinib in intrahepatic cholangiocarcinoma (iCCA) harboring FGFR2 fusions/rearrangements. J Clin Oncol 2022;40: [Crossref]
- Abou-Alfa GK, Sahai V, Hollebecque A, et al. Pemigatinib for previously treated, locally advanced or metastatic cholangiocarcinoma: a multicentre, open-label, phase 2 study. Lancet Oncol 2020;21:671-84. [Crossref] [PubMed]
- Hollebecque A, Borad M, Sahai V, et al. Interim results of fight-202, a phase II, open-label, multicenter study of INCB054828 in patients (pts) with previously treated advanced/metastatic or surgically unresectable cholangiocarcinoma (CCA) with/without fibroblast growth factor (FGF)/FGF receptor (FGFR) genetic alterations. Ann Oncol 2018;29:viii258. [Crossref]
- Javle MM, Roychowdhury S, Kelley RK, et al. Final results from a phase II study of infigratinib (BGJ398), an FGFR-selective tyrosine kinase inhibitor, in patients with previously treated advanced cholangiocarcinoma harboring an FGFR2 gene fusion or rearrangement. J Clin Oncol 2021;39: [Crossref]
- Mazzaferro V, El-Rayes BF, Droz Dit Busset M, et al. Derazantinib (ARQ 087) in advanced or inoperable FGFR2 gene fusion-positive intrahepatic cholangiocarcinoma. Br J Cancer 2019;120:165-71. [Crossref] [PubMed]
- Javle MM, Abou-Alfa GK, Macarulla T, et al. Efficacy of derazantinib in intrahepatic cholangiocarcinoma patients with FGFR2 mutations or amplifications: Interim results from the phase 2 study FIDES-01. J Clin Oncol 2022;40: [Crossref]
- Goyal L, Borad M, Subbiah V, et al. Abstract P02-02: First results of RLY-4008, a potent and highly selective FGFR2 inhibitor in a first-in-human study in patients with FGFR2-altered cholangiocarcinoma and multiple solid tumors. Molecular Cancer Therapeutics 2021;20:P02.
- Hollebecque A BM, Goyal L, Schram A, et al. Efficacy of RLY-4008, a highly selective FGFR2 inhibitor in patients (pts) with an FGFR2-fusion or rearrangement (f/r), FGFR inhibitor (FGFRi)-naïve cholangiocarcinoma (CCA): ReFocus trial. Ann Oncol 2022;33:S808-69. [Crossref]
- Clark O, Yen K, Mellinghoff IK. Molecular Pathways: Isocitrate Dehydrogenase Mutations in Cancer. Clin Cancer Res 2016;22:1837-42. [Crossref] [PubMed]
- Mondesir J, Willekens C, Touat M, et al. IDH1 and IDH2 mutations as novel therapeutic targets: current perspectives. J Blood Med 2016;7:171-80. [Crossref] [PubMed]
- Borger DR, Tanabe KK, Fan KC, et al. Frequent mutation of isocitrate dehydrogenase (IDH)1 and IDH2 in cholangiocarcinoma identified through broad-based tumor genotyping. Oncologist 2012;17:72-9. [Crossref] [PubMed]
- Kipp BR, Voss JS, Kerr SE, et al. Isocitrate dehydrogenase 1 and 2 mutations in cholangiocarcinoma. Hum Pathol 2012;43:1552-8. [Crossref] [PubMed]
- Ruzzenente A, Fassan M, Conci S, et al. Cholangiocarcinoma Heterogeneity Revealed by Multigene Mutational Profiling: Clinical and Prognostic Relevance in Surgically Resected Patients. Ann Surg Oncol 2016;23:1699-707. [Crossref] [PubMed]
- Abou-Alfa GK, Macarulla T, Javle MM, et al. Ivosidenib in IDH1-mutant, chemotherapy-refractory cholangiocarcinoma (ClarIDHy): a multicentre, randomised, double-blind, placebo-controlled, phase 3 study. Lancet Oncol 2020;21:796-807. [Crossref] [PubMed]
- Zhu AX, Macarulla T, Javle MM, et al. Final results from ClarIDHy, a global, phase III, randomized, double-blind study of ivosidenib (IVO) versus placebo (PBO) in patients (pts) with previously treated cholangiocarcinoma (CCA) and an isocitrate dehydrogenase 1 (IDH1) mutation. J Clin Oncol 2021;39:266. [Crossref]
- Moasser MM. The oncogene HER2: its signaling and transforming functions and its role in human cancer pathogenesis. Oncogene 2007;26:6469-87. [Crossref] [PubMed]
- Kim HJ, Yoo TW, Park DI, et al. Gene amplification and protein overexpression of HER-2/neu in human extrahepatic cholangiocarcinoma as detected by chromogenic in situ hybridization and immunohistochemistry: its prognostic implication in node-positive patients. Ann Oncol 2007;18:892-7. [Crossref] [PubMed]
- Javle M, Churi C, Kang HC, et al. HER2/neu-directed therapy for biliary tract cancer. J Hematol Oncol 2015;8:58. [Crossref] [PubMed]
- Harding J, Cleary J, Shapiro G, et al. Treating HER2-mutant advanced biliary tract cancer with neratinib: benefits of HER2-directed targeted therapy in the phase 2 SUMMIT ‘basket’trial. Ann Oncol 2019;30:iv127. [Crossref]
- Harding JJ, Piha-Paul S, Shah RH, et al. Targeting HER2 mutation-positive advanced biliary tract cancers with neratinib: Final results from the phase 2 SUMMIT ‘basket’trial. Change 2022;40:200.
- Javle MM, Oh DY, Ikeda M, et al. Results from TreeTopp: A randomized phase II study of the efficacy and safety of varlitinib plus capecitabine versus placebo in second-line (2L) advanced or metastatic biliary tract cancer (BTC). J Clin Oncol 2020;38:4597. [Crossref]
- Javle M, Borad MJ, Azad NS, et al. Pertuzumab and trastuzumab for HER2-positive, metastatic biliary tract cancer (MyPathway): a multicentre, open-label, phase 2a, multiple basket study. Lancet Oncol 2021;22:1290-300. [Crossref] [PubMed]
- Ohba A, Morizane C, Kawamoto Y, et al. Trastuzumab deruxtecan (T-DXd; DS-8201) in patients (pts) with HER2-expressing unresectable or recurrent biliary tract cancer (BTC): An investigator-initiated multicenter phase 2 study (HERB trial). J Clin Oncol 2022;40:4006. [Crossref]
- Meric-Bernstam F, Hanna DL, El-Khoueiry AB, et al. Zanidatamab (ZW25) in HER2-positive biliary tract cancers (BTCs): Results from a phase I study. J Clin Oncol 2021;39:299. [Crossref]
- Hechtman JF. NTRK insights: best practices for pathologists. Mod Pathol 2022;35:298-305. [Crossref] [PubMed]
- Demols A, Rocq L, Charry M, et al. NTRK gene fusions in biliary tract cancers. J Clin Oncol 2020;38: [Crossref]
- Doebele RC, Drilon A, Paz-Ares L, et al. Entrectinib in patients with advanced or metastatic NTRK fusion-positive solid tumours: integrated analysis of three phase 1-2 trials. Lancet Oncol 2020;21:271-82. [Crossref] [PubMed]
- Drilon A, Laetsch TW, Kummar S, et al. Efficacy of Larotrectinib in TRK Fusion-Positive Cancers in Adults and Children. N Engl J Med 2018;378:731-9. [Crossref] [PubMed]
- Holderfield M, Deuker MM, McCormick F, et al. Targeting RAF kinases for cancer therapy: BRAF-mutated melanoma and beyond. Nat Rev Cancer 2014;14:455-67. [Crossref] [PubMed]
- Goeppert B, Frauenschuh L, Renner M, et al. BRAF V600E-specific immunohistochemistry reveals low mutation rates in biliary tract cancer and restriction to intrahepatic cholangiocarcinoma. Mod Pathol 2014;27:1028-34. [Crossref] [PubMed]
- Wainberg ZA, Lassen UN, Elez E, et al. Efficacy and safety of dabrafenib (D) and trametinib (T) in patients (pts) with BRAF V600E–mutated biliary tract cancer (BTC): A cohort of the ROAR basket trial. J Clin Oncol 2019;37: [Crossref]
- Salama AKS, Li S, Macrae ER, et al. Dabrafenib and Trametinib in Patients With Tumors With BRAFV600E Mutations: Results of the NCI-MATCH Trial Subprotocol H. J Clin Oncol 2020;38:3895-904. [Crossref] [PubMed]
- Wee P, Wang Z. Epidermal Growth Factor Receptor Cell Proliferation Signaling Pathways. Cancers (Basel) 2017;9:52. [Crossref] [PubMed]
- Nakazawa K, Dobashi Y, Suzuki S, et al. Amplification and overexpression of c-erbB-2, epidermal growth factor receptor, and c-met in biliary tract cancers. J Pathol 2005;206:356-65. [Crossref] [PubMed]
- Yoshikawa D, Ojima H, Iwasaki M, et al. Clinicopathological and prognostic significance of EGFR, VEGF, and HER2 expression in cholangiocarcinoma. Br J Cancer 2008;98:418-25. [Crossref] [PubMed]
- Gruenberger B, Schueller J, Heubrandtner U, et al. Cetuximab, gemcitabine, and oxaliplatin in patients with unresectable advanced or metastatic biliary tract cancer: a phase 2 study. Lancet Oncol 2010;11:1142-8. [Crossref] [PubMed]
- Malka D, Cervera P, Foulon S, et al. Gemcitabine and oxaliplatin with or without cetuximab in advanced biliary-tract cancer (BINGO): a randomised, open-label, non-comparative phase 2 trial. Lancet Oncol 2014;15:819-28. [Crossref] [PubMed]
- Sohal DP, Mykulowycz K, Uehara T, et al. A phase II trial of gemcitabine, irinotecan and panitumumab in advanced cholangiocarcinoma. Ann Oncol 2013;24:3061-5. [Crossref] [PubMed]
- Leone F, Marino D, Cereda S, et al. Panitumumab in combination with gemcitabine and oxaliplatin does not prolong survival in wild-type KRAS advanced biliary tract cancer: A randomized phase 2 trial (Vecti-BIL study). Cancer 2016;122:574-81. [Crossref] [PubMed]
- Philip PA, Mahoney MR, Allmer C, et al. Phase II study of erlotinib in patients with advanced biliary cancer. J Clin Oncol 2006;24:3069-74. [Crossref] [PubMed]
- Lee J, Park SH, Chang HM, et al. Gemcitabine and oxaliplatin with or without erlotinib in advanced biliary-tract cancer: a multicentre, open-label, randomised, phase 3 study. Lancet Oncol 2012;13:181-8. [Crossref] [PubMed]
- Kato S, Subbiah V, Marchlik E, et al. RET Aberrations in Diverse Cancers: Next-Generation Sequencing of 4,871 Patients. Clin Cancer Res 2017;23:1988-97. [Crossref] [PubMed]
- Subbiah V, Hu MIN, Gainor JF, et al. Clinical activity of the RET inhibitor pralsetinib (BLU-667) in patients with RET fusion–positive solid tumors. American Society of Clinical Oncology; 2021.
- Demols A, Borbath I, Van den Eynde M, et al. Regorafenib after failure of gemcitabine and platinum-based chemotherapy for locally advanced/metastatic biliary tumors: REACHIN, a randomized, double-blind, phase II trial. Ann Oncol 2020;31:1169-77. [Crossref] [PubMed]
- Yoo C, Oh DY, Choi HJ, et al. Phase I study of bintrafusp alfa, a bifunctional fusion protein targeting TGF-β and PD-L1, in patients with pretreated biliary tract cancer. J Immunother Cancer 2020;8:e000564. [Crossref] [PubMed]
- Javle MM, Bridgewater JA, Gbolahan OB, et al. A phase I/II study of safety and efficacy of the arginase inhibitor INCB001158 plus chemotherapy in patients (Pts) with advanced biliary tract cancers. J Clin Oncol 2021;39:311. [Crossref]
- Hong DS, Kuo J, Sacher AG, et al. CodeBreak 100: Phase I study of AMG 510, a novel KRASG12C inhibitor, in patients (pts) with advanced solid tumors other than non-small cell lung cancer (NSCLC) and colorectal cancer (CRC). J Clin Oncol 2020;38:3511. [Crossref]
- Guo Y, Feng K, Liu Y, et al. Phase I Study of Chimeric Antigen Receptor-Modified T Cells in Patients with EGFR-Positive Advanced Biliary Tract Cancers. Clin Cancer Res 2018;24:1277-86. [Crossref] [PubMed]
- Valle JW, Lamarca A, Goyal L, et al. New Horizons for Precision Medicine in Biliary Tract Cancers. Cancer Discov 2017;7:943-62. [Crossref] [PubMed]
- Weinberg BA, Xiu J, Lindberg MR, et al. Molecular profiling of biliary cancers reveals distinct molecular alterations and potential therapeutic targets. J Gastrointest Oncol 2019;10:652-62. [Crossref] [PubMed]
- Lowery MA, Ptashkin R, Jordan E, et al. Comprehensive Molecular Profiling of Intrahepatic and Extrahepatic Cholangiocarcinomas: Potential Targets for Intervention. Clin Cancer Res 2018;24:4154-61. [Crossref] [PubMed]
- Mody K, Jain P, El-Refai SM, et al. Clinical, Genomic, and Transcriptomic Data Profiling of Biliary Tract Cancer Reveals Subtype-Specific Immune Signatures. JCO Precis Oncol 2022;6:e2100510. [Crossref] [PubMed]
- Sia D, Hoshida Y, Villanueva A, et al. Integrative molecular analysis of intrahepatic cholangiocarcinoma reveals 2 classes that have different outcomes. Gastroenterology 2013;144:829-40. [Crossref] [PubMed]
- Qiu Z, Ji J, Xu Y, et al. Epigenomic analysis of biliary tract cancer identifies subtypes with different immune characteristics and clinical outcomes. J Clin Oncol 2021;39:e16153. [Crossref]
- Jusakul A, Cutcutache I, Yong CH, et al. Whole-Genome and Epigenomic Landscapes of Etiologically Distinct Subtypes of Cholangiocarcinoma. Cancer Discov 2017;7:1116-35. [Crossref] [PubMed]
- Dong L, Lu D, Chen R, et al. Proteogenomic characterization identifies clinically relevant subgroups of intrahepatic cholangiocarcinoma. Cancer Cell 2022;40:70-87.e15. [Crossref] [PubMed]
- Job S, Rapoud D, Dos Santos A, et al. Identification of Four Immune Subtypes Characterized by Distinct Composition and Functions of Tumor Microenvironment in Intrahepatic Cholangiocarcinoma. Hepatology 2020;72:965-81. [Crossref] [PubMed]
- Jahr S, Hentze H, Englisch S, et al. DNA fragments in the blood plasma of cancer patients: quantitations and evidence for their origin from apoptotic and necrotic cells. Cancer Res 2001;61:1659-65. [PubMed]
- Winter H, Kaisaki PJ, Harvey J, et al. Identification of Circulating Genomic and Metabolic Biomarkers in Intrahepatic Cholangiocarcinoma. Cancers (Basel) 2019;11:1895. [Crossref] [PubMed]
- Ettrich TJ, Schwerdel D, Dolnik A, et al. Genotyping of circulating tumor DNA in cholangiocarcinoma reveals diagnostic and prognostic information. Sci Rep 2019;9:13261. [Crossref] [PubMed]
- Okamura R, Kurzrock R, Mallory RJ, et al. Comprehensive genomic landscape and precision therapeutic approach in biliary tract cancers. Int J Cancer 2021;148:702-12. [Crossref] [PubMed]
- Lamarca A, Kapacee Z, Breeze M, et al. Molecular Profiling in Daily Clinical Practice: Practicalities in Advanced Cholangiocarcinoma and Other Biliary Tract Cancers. J Clin Med 2020;9:2854. [Crossref] [PubMed]
- Zill OA, Greene C, Sebisanovic D, et al. Cell-Free DNA Next-Generation Sequencing in Pancreatobiliary Carcinomas. Cancer Discov 2015;5:1040-8. [Crossref] [PubMed]
- Berchuck JE, Facchinetti F, DiToro DF, et al. The clinical landscape of cell-free DNA alterations in 1671 patients with advanced biliary tract cancer. Ann Oncol 2022;33:1269-83. [Crossref] [PubMed]
- Kinugasa H, Nouso K, Ako S, et al. Liquid biopsy of bile for the molecular diagnosis of gallbladder cancer. Cancer Biol Ther 2018;19:934-8. [Crossref] [PubMed]
- Voigtländer T, Gupta SK, Thum S, et al. MicroRNAs in Serum and Bile of Patients with Primary Sclerosing Cholangitis and/or Cholangiocarcinoma. PLoS One 2015;10:e0139305. [Crossref] [PubMed]
- Li L, Masica D, Ishida M, et al. Human bile contains microRNA-laden extracellular vesicles that can be used for cholangiocarcinoma diagnosis. Hepatology 2014;60:896-907. [Crossref] [PubMed]
- Chen L, Yan HX, Yang W, et al. The role of microRNA expression pattern in human intrahepatic cholangiocarcinoma. J Hepatol 2009;50:358-69. [Crossref] [PubMed]
- Xue XY, Liu YX, Wang C, et al. Identification of exosomal miRNAs as diagnostic biomarkers for cholangiocarcinoma and gallbladder carcinoma. Signal Transduct Target Ther 2020;5:77. [Crossref] [PubMed]