The future is here—targeted therapies are coming of age in biliary tract cancers
Biliary tract cancer (BTC) is a rare human tumor entity comprising intrahepatic, extrahepatic and gallbladder malignancies. The overall prognosis of advanced and metastatic disease stages remains unsatisfactory with a median survival of about 1 year only. While conventional chemotherapy regimens (e.g., gemcitabine or 5-fluorouracil combinations) still remain important treatment options, the broader availability of mutational and genomic analyses via next-generation sequencing technologies provided an opportunity for novel targeted therapies in distinct sub-populations of BTC (Figure 1). As summarized by Banales et al., heterogeneous signaling pathways are involved in cholangiocarcinogenesis. These processes are further complicated by the interplay of extracellular ligands within the tumor microenvironment with increased expression and/or aberrant activation of cell surface receptors in combination with deregulated intracellular signaling pathways (such as KRAS, BRAF, ARID1, PBRM1, BAP1, IDH1, and IDH2) (4).
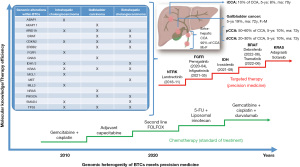
LaPelusa et al. have comprehensively summarized some of those novel therapeutic approaches and showed that especially FGFR2- and IDH1-targeting agents achieve clinically meaningful responses in the intrahepatic subtype of BTC (5). The small molecule fibroblast growth factor receptor (FGFR) inhibitor futibatinib recently received Food and Drug Administration (FDA) approval for the treatment of intrahepatic cholangiocarcinomas harboring FGFR2 gene rearrangements (6). Unfortunately, these compounds work only in a minor subset of patients due to the low prevalence of these alterations, which is highest in intrahepatic cholangiocarcinomas [FGFR2 fusions: up to 15% (7), IDH1 mutations: approximately 13% (8)]. Recent comprehensive sequencing analyses revealed that potentially actionable mutations could be found in approximately 27% of extrahepatic cholangiocarcinomas and 50% of intrahepatic cholangiocarcinomas and gallbladder cancers, respectively (9,10). This supports the need to look in more detail into the mode of gene alterations (gene fusions/arrangements, mutations or amplifications) for diagnostic and therapeutic purposes (11). Overall, these numbers underline the importance of identifying novel therapeutic targets and approaches to overcome resistance development (12) and improve the poor overall response rate and prognosis for patients with BTC. Especially, resistance to targeted therapy of BTC is an often observed clinical situation which could be related to different molecular mechanisms of chemoresistance (MOC) like changes of drug transporters (MOC 1a), changes in drug molecular targets (MOC 3a), activation of alternative signaling pathways through other growth receptors (MOC 3b), drug-induced selection of tumor cell subclones (MOC 3c) or changes in the balance between pro- and anti-apoptotic proteins (MOC 5a/b) indicating the complexity of such targeted therapies in BTCs (12).
Immune checkpoint inhibitors have become a mainstay of modern oncology. LaPelusa et al. also highlight recent studies using immune checkpoint inhibitor antibodies alone or in combination with chemotherapy or targeted agents in BTC. Similar to other solid tumors, overall response rates do not exceed 40% although some responses are durable (5). The combination of durvalumab, gemcitabine and cisplatin received FDA approval based on the phase 3 TOPAZ-1 study. Although objective response rate was 26.7% in the triple combination vs. 18.7% for chemotherapy only, addition of durvalumab to the chemotherapy backbone more than doubled overall survival at 24 months, reaching 24.9% vs. 10.4% without significantly increasing toxicity (13).
In BTC, too, there still is debate on predictivity of tumor mutational burden (TMB), programmed death ligand 1 (PD-L1) expression level and DNA mismatch repair/microsatellite stability, which overall show low expression or positivity in BTC (10,14). Novel targets regulating the tumor-immune microenvironment include LAG3, TIGIT, or CD47/SIRPα are currently explored in early clinical studies but no clinical data for BTC is available yet (15-17).
mRNA based cancer vaccines are considered the next step in immuno-oncology and several early clinical studies explore various tumor-antigens like carcinoembryonic antigen (CEA), modified KRAS, tyrosinase or novel antigens like CD247, FCGR1A, or TRAP in BTC (18,19). Other novel experimental approaches include the use of antibody-drug conjugates, e.g., targeting anti-glypican-1 or FGFR2 (20,21), or enhancing the efficacy of photodynamic therapy by coupling a photosensitizer to a TROP2-targeting antibody (22). Also, several approaches using chimeric antigen receptor (CAR)-T cells have been explored that included BTC patients (23) but data on their clinical efficacy are still pending.
LaPelusa et al. also highlight the importance of non-invasive detection and monitoring of alterations in BTC (5). This is crucial for early detection but also to understand the mode of action and potential mechanisms of resistance against these novel therapies. Driver alterations like IDH1 mutation or FGFR2 fusions can already be reliably detected from circulating DNA samples (24,25). Further technical refinements and broader availability of such technologies will increase the number of patients who can actually benefit from these novel therapies.
Finally, an important aspect of all targeted therapies, especially in BTC, is a tissue-based problem: With the exception of most intrahepatic BTCs, all other forms of BTC are anatomically difficult for radiologists, endoscopists or surgeons to obtain diagnostically relevant and sufficient tumor tissue. As a result, the time between diagnosis and the start of any targeted therapy is too long, and the amount of BTC tissue available is usually minimal. The small amount of tumor tissue raises the question which next step of biomarker analysis (immunohistochemistry, in situ hybridization, panel or whole genome sequencing) should be performed. Therefore, an intensive interaction of all clinical and molecular stakeholders in the care process of patients with BTCs is necessary to get deep and detailed molecular information as soon as possible.
In summary, several promising novel targets and therapeutic modalities are currently investigated in the various subtypes of BTCs. The increasing use of molecular diagnostics like next-generation sequencing panels or the development of circulating DNA technologies increases the probability to find an actionable alteration that could lead to improved overall survival for patients with BTC. The field is quickly moving away from unselected chemotherapy combinations into the era of advanced precision medicine in a real-world scenario.
Acknowledgments
Funding: None.
Footnote
Provenance and Peer Review: This article was commissioned by the editorial office, Chinese Clinical Oncology. The article did not undergo external peer review.
Conflicts of Interest: Both authors have completed the ICMJE uniform disclosure form (available at https://cco.amegroups.com/article/view/10.21037/cco-23-20/coif). DN reports honoraria for advisory function of Boehringer Ingelheim and Lilly. MO has no conflicts of interest to declare.
Ethical Statement: The authors are accountable for all aspects of the work in ensuring that questions related to the accuracy or integrity of any part of the work are appropriately investigated and resolved.
Open Access Statement: This is an Open Access article distributed in accordance with the Creative Commons Attribution-NonCommercial-NoDerivs 4.0 International License (CC BY-NC-ND 4.0), which permits the non-commercial replication and distribution of the article with the strict proviso that no changes or edits are made and the original work is properly cited (including links to both the formal publication through the relevant DOI and the license). See: https://creativecommons.org/licenses/by-nc-nd/4.0/.
References
- Cowzer D, Harding JJ. Advanced Bile Duct Cancers: A Focused Review on Current and Emerging Systemic Treatments. Cancers (Basel) 2022;14:1800. [Crossref] [PubMed]
- Personeni N, Lleo A, Pressiani T, et al. Biliary Tract Cancers: Molecular Heterogeneity and New Treatment Options. Cancers (Basel) 2020;12:3370. [Crossref] [PubMed]
- Valle JW, Lamarca A, Goyal L, et al. New Horizons for Precision Medicine in Biliary Tract Cancers. Cancer Discov 2017;7:943-62. [Crossref] [PubMed]
- Banales JM, Marin JJG, Lamarca A, et al. Cholangiocarcinoma 2020: the next horizon in mechanisms and management. Nat Rev Gastroenterol Hepatol 2020;17:557-88. [Crossref] [PubMed]
- LaPelusa M, Heumann T, Goff L, et al. Targeted therapies in advanced biliary tract cancers-a narrative review. Chin Clin Oncol 2023;12:14. [Crossref] [PubMed]
- Goyal L, Meric-Bernstam F, Hollebecque A, et al. Futibatinib for FGFR2-Rearranged Intrahepatic Cholangiocarcinoma. N Engl J Med 2023;388:228-39. [Crossref] [PubMed]
- Goyal L, Kongpetch S, Crolley VE, et al. Targeting FGFR inhibition in cholangiocarcinoma. Cancer Treat Rev 2021;95:102170. [Crossref] [PubMed]
- Boscoe AN, Rolland C, Kelley RK. Frequency and prognostic significance of isocitrate dehydrogenase 1 mutations in cholangiocarcinoma: a systematic literature review. J Gastrointest Oncol 2019;10:751-65. [Crossref] [PubMed]
- Okawa Y, Ebata N, Kim NKD, et al. Actionability evaluation of biliary tract cancer by genome transcriptome analysis and Asian cancer knowledgebase. Oncotarget 2021;12:1540-52. [Crossref] [PubMed]
- de Bitter TJJ, de Reuver PR, de Savornin Lohman EAJ, et al. Comprehensive clinicopathological and genomic profiling of gallbladder cancer reveals actionable targets in half of patients. NPJ Precis Oncol 2022;6:83. [Crossref] [PubMed]
- Ellinghaus P, Neureiter D, Nogai H, et al. Patient Selection Approaches in FGFR Inhibitor Trials-Many Paths to the Same End? Cells 2022;11:3180. [Crossref] [PubMed]
- Mayr C, Kiesslich T, Modest DP, et al. Chemoresistance and resistance to targeted therapies in biliary tract cancer: what have we learned? Expert Opin Investig Drugs 2022;31:221-33. [Crossref] [PubMed]
- Oh D Y, Ruth He A, Qin S, et al. Durvalumab plus gemcitabine and cisplatin in advanced biliary tract cancer. NEJM Evidence 2022;1:EVIDoa2200015.
- Rizzo A, Ricci AD, Brandi G. PD-L1, TMB, MSI, and Other Predictors of Response to Immune Checkpoint Inhibitors in Biliary Tract Cancer. Cancers (Basel) 2021;13:558. [Crossref] [PubMed]
- Lee SJ, Byeon SJ, Lee J, et al. LAG3 in Solid Tumors as a Potential Novel Immunotherapy Target. J Immunother 2019;42:279-83. [Crossref] [PubMed]
- Ostroumov D, Duong S, Wingerath J, et al. Transcriptome Profiling Identifies TIGIT as a Marker of T-Cell Exhaustion in Liver Cancer. Hepatology 2021;73:1399-418. [Crossref] [PubMed]
- Yang H, Yan M, Li W, et al. SIRPα and PD1 expression on tumor-associated macrophage predict prognosis of intrahepatic cholangiocarcinoma. J Transl Med 2022;20:140. [Crossref] [PubMed]
- Huang X, Tang T, Zhang G, et al. Identification of tumor antigens and immune subtypes of cholangiocarcinoma for mRNA vaccine development. Mol Cancer 2021;20:50. [Crossref] [PubMed]
- Tang TY, Huang X, Zhang G, et al. mRNA vaccine development for cholangiocarcinoma: a precise pipeline. Mil Med Res 2022;9:40. [Crossref] [PubMed]
- Yokota K, Serada S, Tsujii S, et al. Anti-Glypican-1 Antibody-drug Conjugate as Potential Therapy Against Tumor Cells and Tumor Vasculature for Glypican-1-Positive Cholangiocarcinoma. Mol Cancer Ther 2021;20:1713-22. [Crossref] [PubMed]
- Kim SB, Meric-Bernstam F, Kalyan A, et al. First-in-Human Phase I Study of Aprutumab Ixadotin, a Fibroblast Growth Factor Receptor 2 Antibody-Drug Conjugate (BAY 1187982) in Patients with Advanced Cancer. Target Oncol 2019;14:591-601. [Crossref] [PubMed]
- Nishimura T, Mitsunaga M, Sawada R, et al. Photoimmunotherapy targeting biliary-pancreatic cancer with humanized anti-TROP2 antibody. Cancer Med 2019;8:7781-92. [Crossref] [PubMed]
- Anwar MY, Williams GR, Paluri RK. CAR T Cell Therapy in Pancreaticobiliary Cancers: a Focused Review of Clinical Data. J Gastrointest Cancer 2021;52:1-10. [Crossref] [PubMed]
- Varghese AM, Patel J, Janjigian YY, et al. Noninvasive Detection of Polyclonal Acquired Resistance to FGFR Inhibition in Patients With Cholangiocarcinoma Harboring FGFR2 Alterations. JCO Precis Oncol 2021;5:PO.20.00178.
- Lapin M, Huang HJ, Chagani S, et al. Monitoring of Dynamic Changes and Clonal Evolution in Circulating Tumor DNA From Patients With IDH-Mutated Cholangiocarcinoma Treated With Isocitrate Dehydrogenase Inhibitors. JCO Precis Oncol 2022;6:e2100197. [Crossref] [PubMed]