Role of radiotherapy and its contribution to immunotherapy in hepatocellular carcinoma
Introduction
Overview of hepatocellular carcinoma (HCC) incidence and risk factors
HCC is a major contributor to global cancer-related deaths (1,2). The incidence of HCC is increasing in many regions of the world, particularly in areas with a high incidence of hepatitis B and C (3). HCC has a male predominance with a two-fold higher incidence in males than females and a higher prevalence in East Asian countries relative to European countries (4). The incidence and mortality of HCC in the United States are undergoing a shift, with a transition from predominantly affecting Asians/Pacific Islanders to affecting African American and Hispanic communities (5). This change is likely attributed to the effective implementation of hepatitis B virus (HBV) control measures, including vaccination and antiviral therapy, as HBV is the primary cause of HCC in Asian populations (6,7). Other major risk factors for HCC, besides chronic viral hepatitis, include cirrhosis, nonalcoholic steatohepatitis associated with metabolic syndrome, alcohol abuse, tobacco consumption, and aflatoxin B1 (2,8,9).
Current treatments for advanced HCC
The Barcelona Clinic Liver Cancer (BCLC) staging system utilizes the patient’s liver function, tumor characteristics, and performance status to guide treatment decisions (10-12) (Table 1). The majority of patients present with BCLC B or later stages which are not amenable for curative intent therapies such as resection, ablation or transplantation. In fact, local treatments such as transarterial chemoembolization (TACE) only serve a minority of patients in the BCLC B stage with well-defined nodules, preserved portal flow without portal hypertension, and selective access to tumor-feeding arteries (13-15). TACE has often served as a bridge to transplantation, but many patients cannot ultimately receive transplantation due to either the progression of the disease or the determination of liver function (16-18).
Table 1
Classification component | BCLC stage | ||||
---|---|---|---|---|---|
0 | A | B | C | D | |
Tumor burden | Single ≤2 cm | Single or ≤3 nodules each ≤3 cm | Multinodular | Portal invasion and/or extrahepatic spread | Any tumor burden |
Liver function (CPC) | A | A or B | A or B | A or B | C |
PS (ECOG scale) | 0 | 0 | 0 | 1 or 2 | 3 or 4 |
The tumor burden encompasses the number, size, portal and extrahepatic spread. Liver function is based on the CPC, which measures the severity of liver disease according to ascites, serum concentration of bilirubin, serum concentration of albumin, prothrombin time, and degree of encephalopathy. CPC A is a well-compensated disease, CPC B indicates significant functional compromise, and CPC C represents a decompensated disease state. The PS is based on the ECOG scale. PS 0 is where one is fully active and can carry on all pre-disease performance without restrictions. PS 1 is where a patient is restricted in heavy physical work but can perform work of a light or sedentary nature. PS 2 is where the patient is ambulatory and capable of self-care but cannot carry out any work activities. This patient is confined to the bed less than 50% of the time. PS 3 is where the patient can only have limited self-care and is confined to bed or chair more than 50% of the time. PS 4 is where a patient is completely disabled and cannot carry out self-care. The patient is confined to the bed or chair 100% of the time. BCLC, Barcelona Clinic Liver Cancer; CPC, Child-Pugh Class; PS, Performance Status; ECOG, Eastern Cooperative Oncology Group.
The 2022 BCLC updated guidelines have been revised to offer guidance on first- and second-line systemic therapies for patients with advanced-stage HCC (BCLC C) (10,19). The atezolizumab/bevacizumab [programmed death ligand-1 (PD-L1)/anti-vascular endothelial growth factor (VEGF)] combination is currently regarded as the standard of care for HCC patients with BCLC C or any patients not amendable for or have progressed after curative or local therapies. A combination of tremelimumab and durvalumab has been shown to be more effective than sorafenib and is another first-line treatment option (20). Both regimens significantly improve overall survival (OS) in the first-line setting (20-23).
Limitations of current systemic therapies in HCC
It is now well-accepted that immunotherapy offers the best potential for optimal outcomes in advanced HCC. Such regimens were only studied in patients with Eastern Cooperative Oncology Group Performance Status (ECOG PS) status at least 1 or better and in patients with a preserved liver function such as Child-Pugh Class (CPC) A (20,21,23). Unfortunately, many patients with HCC are diagnosed with either ECOG PS status of 2 or CPC B (24-26), leaving many patients without adequate therapeutic options (24,27-29).
The rationale of radiotherapy (RT) utilization in HCC
Although the updated 2022 BCLC guidelines did not incorporate RT in the modified treatment algorithm for HCC, RT has recently emerged as a potential therapy (30-35). Liver tumors exhibit moderate sensitivity to radiation (36). The sensitivities of tissues to radiation are determined by their cellular states of differentiation, division, and potential to divide. The hematopoietic system, reproductive system, gastrointestinal lining, and skin are more radiosensitive than solid organs such as the kidney, lungs, and liver. Muscles and nerves are less radiosensitive than the liver (37).
RT is a localized treatment that utilizes ionizing radiation to induce direct and indirect DNA double-strand breaks (38). Ionizing radiation damages tumor cells by causing direct damage to their DNA, particularly double-strand DNA breaks (39,40). Irradiating water within the cytoplasm of tumor cells can lead to the formation of reactive oxygen species. This ionization results in indirect unrepairable double-strand DNA breaks that disrupt tumor function (41).
The abscopal effect in RT
An advantage of localized irradiation is the abscopal effect (42). The abscopal effect refers to the phenomenon where local RT leads to the regression of metastatic cancer at a distance outside the irradiated site, mediated by the activation of the immune system (43-46). In this phenomenon, RT can induce immunogenic cell death in irradiated tumor cells, which leads to the release of neoantigens into the tumor microenvironment (47,48). Neoantigens predominantly represent novel tumor-specific antigens that arise from mutations within neoplastic cells, resulting in their exclusive expression in the malignant cellular population (49,50).
These neoantigens are taken up by antigen-presenting cells and processed to become mature dendritic cells (DCs). These mature DCs then migrate to the tumor-draining lymph nodes. The neoantigens are presented to T cells, a process mediated by the major histocompatibility complex (MHC) pathway and other co-stimulatory signals, such as CD80 and CD28. The T cells differentiate into cytotoxic T lymphocytes, which can recognize and eliminate tumor cells as they recirculate within the body (42,51-53) (Figure 1). This is particularly relevant when combined with immunotherapy such as cytotoxic T-lymphocyte-associated protein 4 (CTLA 4) antibody that energizes cytotoxic T cells. The beneficial effects on cytotoxic T cells by the abscopal effect can be important when combined with programmed death-1 (PD-1) inhibitors, as cytotoxic T cells and the PD-1 pathway have been shown to be synergistic in HCC treatment. This has been illustrated by the significant OS benefits of combining CTLA 4 antibody and PD-1 inhibitor (54,55).
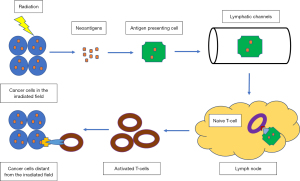
Despite a growing number of trials and cases reporting the abscopal effect of RT alone, the overall occurrence rate has been observed to be relatively low (56-58). Since the introduction of immune checkpoint inhibitors (ICIs) in oncology, interest in the abscopal effects of radiation has increased dramatically. It presents the idea of possible potentiation of abscopal effects in patients receiving RT and ICIs, particularly in cancers where RT has a limited role, whereas immunotherapy is beneficial. The low rate of abscopal effect can be attributed to the insufficient ability of RT alone to overcome the immunoresistance of malignant tumors (56-59). Combining immunotherapy with RT can enhance the systemic anti-tumor response of RT, possibly due to the reduction of immune tolerance toward tumors by immunotherapy (56,57). This combination has been a subject of intense interest as it is thought to amplify the anti-tumor immune response and increase the likelihood of an abscopal effect (47,60,61). Moreover, the release of neoantigens by RT presents increased areas for PD-1 inhibitors to target. It helps overcome immune exhaustion experienced by patients on long-term immunotherapy and resensitizes tumors to the effects of immunotherapy (47,56).
Translational research has explored the potentiation of immunotherapy by RT. In a study investigating the antitumor effect of a combination of immune checkpoint blockade and RT, the authors found that in vitro radiation application upregulated PD-L1 protein expression in HCC murine and human cell lines (62). In vivo radiation exposure also upregulated PD-L1 expression in a dose-dependent manner in murine HCC tumor tissue. Investigation into the signaling pathways responsible for radiation-induced upregulation of PD-L1 suggests that radiation initially induces the production of IFN-γ, which then signals downstream expression of STAT3, ultimately increasing PD-L1 expression in HCC tumor cells. Mice receiving a combination of anti-PD-L1 treatment and radiation demonstrated significantly suppressed tumor growth and increased survival rate compared to those receiving anti-PD-L1 or radiation alone. These findings support the reasoning that radiation increases the susceptibility of tumor cells to anti-PD-L1 treatment by increasing induction of PD-L1.
It is also important to note that enhanced PD-L1 expression can promote T cell exhaustion and subsequent dysfunction through the upregulation of inhibitory receptors such as PD-1 and CTLA-4. The authors found that combination treatment not only increased the percentage of CD8 T cells but also increased IFN-γ CD8 T cells, CD107a CD8 T cells, and Ki67 CD8 T cells, indicating an increased effector cell cytotoxicity and proliferation, when compared to those treated with monotherapy (62).
Therapeutic benefits of RT in HCC
RT is an emerging option for treating HCC across all BCLC stages (31). Historically, the use of external beam radiotherapy (EBRT) has been restricted in managing HCC due to technical limitations and fear of hepatic toxicities (63). Recent technological advancements have ensured the precise delivery of high doses of radiation to the liver for HCC while minimizing damage to normal tissue (38,64,65). In addition to EBRT for the treatment of HCC, other forms of RT include stereotactic body radiotherapy (SBRT), including CyberKnife (66,67), selective internal radiation therapy (SIRT) (68), carbon iron radiation therapy (CIRT) (69), and proton beam therapy (PBT) (70-76) (Table 2).
Table 2
Treatment method | Description | Delivery method | Main advantages | Main disadvantages |
---|---|---|---|---|
EBRT (72) | A traditional form of radiation therapy that uses external beams of radiation to treat cancer | External beam directed at the tumor, with intensity modulated to spare healthy tissue | Can treat a larger area and is not limited by the size or shape of the tumor | Requires many treatments over several weeks. More exposure of healthy tissue to radiation, which can lead to side effects |
SBRT (73) | A radiation therapy that delivers very high doses of radiation to a small, precise area of the body | External beam targeted precisely to the tumor | Can treat smaller, well-defined tumors. Fewer treatments needed than traditional radiation. Minimizes exposure to healthy tissue | May not be suitable for larger or less well-defined tumors. Can have side effects such as fatigue, skin changes, and other symptoms depending on the location of the treatment |
CyberKnife (66,67) | A type of SBRT. It uses a robotic arm to deliver highly focused beams of radiation | External beam targeted precisely to the tumor, capable of adjusting to patient movement | Offers real-time tracking and adjustment for patient movement, making it highly precise. Can treat tumors in challenging or risky areas | Limited by the size of the tumor and can be more costly. Requires sophisticated equipment and experienced clinicians |
SIRT (68,74) | A form of internal radiation therapy where radioactive microspheres are injected into the blood vessels that feed the tumor | Internal, via catheter directly into blood vessels feeding the tumor | Delivers radiation directly to the tumor, sparing healthy liver tissue. Can be used in patients with larger or multifocal liver disease | Risk of radiation exposure to non-targeted tissues and can be more costly. More invasive procedure. Requires a specialized interventional radiology team |
CIRT (69,75) | A type of particle therapy that uses carbon ions to treat cancer | External beam directed at the tumor | Carbon ions are heavier and have a higher LET, leading to more precise targeting and potential for greater tumor kill | Limited availability as it requires specialized equipment and can be more costly. More research is needed to fully understand the long-term effects and comparative effectiveness |
PBT (70,76) | A type of radiation therapy that uses protons to treat cancer | External beam directed at the tumor | Protons stop at the tumor site, reducing exposure of healthy tissue beyond the tumor. Can be more precise than traditional radiation | Requires specialized equipment and can be more costly. More research is needed to fully understand the long-term effects and comparative effectiveness |
RT, radiotherapy; HCC, hepatocellular carcinoma; EBRT, external beam radiation therapy; SBRT, stereotactic body radiation therapy; SIRT, selective internal radiation therapy; CIRT, carbon ion radiation therapy; LET, linear energy transfer; PBT, proton beam therapy.
EBRT and SBRT are both viable radiation therapy options for the management of HCC. However, they possess distinct characteristics, benefits, and limitations. EBRT is a conventional therapy that delivers radiation to the tumor site over multiple sessions. It uses a broad radiation beam that passes through healthy tissue to reach the HCC tumor (77). In contrast, SBRT is a more advanced and targeted form of RT that delivers high doses of radiation to the tumor in fewer sessions (78). SBRT is more precise than EBRT, as it accurately targets the HCC tumor while sparing adjacent healthy tissue (78,79). This allows higher radiation doses to be administered over fewer treatment sessions, resulting in better tumor control and potentially fewer side effects (66,67,79). EBRT typically requires daily treatment sessions over several weeks, while SBRT involves fewer sessions (usually between 1 and 5) (77-79). The use of EBRT as a localized therapeutic approach for advanced HCCs has expanded, particularly in the form of three-dimensional conformal RT (77). The general description, advantages and disadvantages of each form of RT are covered in Table 2.
RT monotherapy in early-stage HCC (BCLC A)
EBRT is utilized for primary HCC with diverse objectives, including ablation of HCC, consolidation of other local treatments, linking to liver transplantation, and treating recalcitrant disease (80-82). SBRT administers targeted, high-dose external radiation to the tumor site while minimizing radiation-induced damage to the adjacent liver parenchyma (31).
A retrospective trial demonstrated that after propensity score matching, the 2-year freedom from local progression rates was 74.9% for the SBRT group and 64.9% for the radiofrequency ablation (RFA) group. This study indicated that SBRT seems to be a promising alternative treatment option for HCC, mainly when RFA is not feasible due to tumor location or size (83). A retrospective analysis and large-scale metanalysis suggested the superiority of SBRT over RFA in local control (LC) for HCC (84-86).
In a randomized phase III-controlled trial comparing PBT to RFA in the treatment of recurrent HCC (size <3 cm, number ≤2), PBT demonstrated non-inferiority to RFA in terms of 2-year local progression-free survival (LPFS) rates, with 94.8% for PBT and 83.9% for RFA (87). The intention-to-treat analysis also showed comparable LPFS rates between the two treatment arms. The progression-free survival (PFS) and OS rates were not significantly different between the two arms.
In a recent study, PBT was utilized as a primary treatment modality in HCC patients, who either could not undergo other localized therapies or opted against them (88). Notably, the use of PBT led to encouraging outcomes, presenting a 5-year freedom from local progression (FFLP) rate of 94.5% and a 5-year OS rate of 76.7% in patients classified as BCLC 0/A/B, where the majority were stage A (92.3%). A comparable study evaluated the same treatment modality, PBT, in a cohort of treatment-naive HCC patients. They documented a 5-year FFLP and OS rates of 94% and 69%, respectively, in BCLC 0/A patients (n=30) (89). The studies affirm the promising potential of PBT as a viable treatment option for HCC patients for BCLC 0/A.
A comparison study was conducted to evaluate the efficacy of CIRT versus TACE in the treatment of single HCC (90). The study included 31 patients who received CIRT and 23 patients who received TACE. After propensity score matching, 17 matched pairs of patients from each treatment group were analyzed. The median follow-up durations after CIRT and TACE were 43 and 32 months, respectively. The 3-year OS, local control, and PFS rates in the CIRT group were significantly higher than those in the TACE group, with rates of 88% vs. 58% (P<0.05), 80% vs. 26% (P<0.01), and 51% vs. 15% (P<0.05), respectively (90). These results suggest that CIRT may be a more effective treatment option for single HCC than TACE.
RT for intermediate stage HCC (BCLC B)
In a retrospective analysis, when compared to TACE, SBRT was linked to better tumor control for primary therapy (91% vs. 23% at 2 years) (91). Moreover, in a prospective study, SBRT was found to be on par with other treatments for bridging to transplantation (92).
In a study looking at treatment-naive HCC patients with PBT, patients categorized as BCLC B (n=34) had 5-year FFLP and OS rates, 87% and 66% respectively that were slightly lower than those seen with BCLC 0/A (89).
SIRT and transarterial radioembolization (TARE) for intermediate-stage HCC (BCLC B)
SIRT, also known as radioembolization, delivers tiny radioactive particles called microspheres directly to the tumor through a catheter, which are then lodged in the tumor’s blood vessels, where they deliver targeted radiation to the cancer cells (93). The microspheres used in SIRT are usually made of a radioactive isotope called yttrium-90 (90Y), which has a short half-life and therefore delivers high doses of radiation to the tumor while minimizing exposure to surrounding healthy tissues (94). This results in less damage to healthy liver tissue and fewer side effects compared to traditional RT (94).
The use of SIRT may have future benefits that are not entirely seen at the moment. In a multi-site, prospective trial comparing sorafenib alone and a combination of sorafenib and SIRT with 90Y microspheres in patients with advanced HCC (BCLC A/B/C, and CPC A to B7), it found median OS was 11.4 months with sorafenib and 12.1 months with sorafenib and SIRT. This study concluded that including SIRT alongside sorafenib did not lead to a notable enhancement in OS when compared to using sorafenib alone (94).
Evidence supporting the effectiveness of radiation therapy in the form of TARE is progressively accumulating. The fundamental concept underlying TARE infusion involves administering high-dose ionizing radiation directly into the tumor bed via the hepatic artery, leading to continuous radiation exposure as 90Y decays over time (31). TARE has gained traction as a frequently employed locoregional therapy for HCC (95,96). In the early and intermediate stages of HCC, TARE segmentectomy is a promising technique that exhibits a positive risk profile and presents favorable radiology-pathology outcomes for HCC that is ≤5 cm (97).
RT for late-stage HCC
LC and OS with RT (Table 3)
Table 3
Trial | Type of study | Prospective vs. retrospective | Radiotherapy | Local control rate at 2 years | Overall survival rate at 2 years |
---|---|---|---|---|---|
Kang et al. (98) | Single-site | Prospective | SBRT | 94.6% | 68.7% |
Andolino et al. (99) | Single site | Prospective | SBRT | 90% | 48% |
Kwon et al. (71) | Single-site | Prospective | SBRT | 67.5% | 77.3% |
Huang et al. (100) | Single-site | Prospective, matched-pair | SBRT | 75% | 72.6% |
Bujold et al. (101) | Single-site | Prospective | SBRT | 87% at 1 year | 55% at 1 year |
Sanuke et al. (102) | Single-site | Retrospective | SBRT | 91% at 3 years | 70% at 3 years |
Jang et al. (103) | Single-site | Prospective | SBRT | 87% | 63% |
Yoon et al. (104) | Single-site | Prospective | EBRT + TACE | None reported | 55.4% at 48 weeks or roughly 28% at 2 years |
HCC, hepatocellular carcinoma; SBRT, stereotactic body radiotherapy; EBRT, external beam radiotherapy; TACE, transarterial chemoembolization.
RT has been shown to achieve moderate LC in patients with unresectable HCC (105-107). LC rates following SBRT for HCC range from 68% to 95% at 2 to 3 years after treatment (71,98-103). In a phase II, single-site, prospective trial of 50 patients who progressed after TACE for inoperable HCC (41 with CPC A, 6 with CPC B7, and 5 with portal vein tumor thrombosis), SBRT achieved an LC rate of 94.6% and OS rate of 68.7% after 2 years (98).
In another single-institution, prospective trial of 60 HCC patients that included 36 CPC A and 24 CPC B, all of the tumors (1 to 3 for each patient) had a maximal diameter of ≤6 cm, SBRT achieved 2-year LC rate of 90% and a 2-year OS rate of 48% (99). A single-site, prospective study using CyberKnife SBRT in 42 HCC patients. Three patients were eligible for local ablation or surgical resection, who had CPC A (38) and B (4) all had a tumor volume ≤100 cc. This study demonstrated LC rates of 72.0% and 67.5% at 1 and 2 years, respectively. OS rates were 92.9% and 77.3% at 1 and 2 years, respectively (71).
A study examined 36 unresectable HCC patients treated with CyberKnife SBRT matched for ECOG PS and CPC with 138 historical control who received other systemic therapies such as thalidomide, sorafenib, TACE, and liver transplantation or no treatments. This analysis showed that patients undergoing CyberKnife had better 2-year OS rates of 72.6% as compared to that of 42.1% in the historical group. Moreover, the SBRT-treated group had an in-field control rate of 75% after 2 years (100).
In a prospective sequential phase I/II trial, 102 HCC patients unsuitable for RFA, TACE, surgery, or alcohol ablation were treated with SBRT (24–55 Gy) in 6 fractions. Patients had ECOG performance score ≤2, CPC A, and at least 700 mL of non-HCC liver. These patients achieved an LC rate of 87% and an OS rate of 55% at 1 year (101).
In a separate retrospective study, where patients with HCC (CPC A and B) were treated with SBRT (40 Gy for CPC A and 35 Gy for PC in 5 fractions), the overall 3-year LC rate and OS rate were 91% and 70%, respectively. No statistical differences in LC or OS were seen between the SBRT dose levels (102). In contrast, a prospective study selected 108 HCC patients with incomplete responses to TACE who were unsuitable for local ablation or surgery. SBRT in these patients showed 2-year LC and OS rates of 87% and 63%, respectively. This investigation also revealed a positive correlation between RT dose and LC, with a similar dose response in OS when utilizing SBRT for HCC treatment. This study also suggested that enhanced LC outcomes may potentially lead to survival advantages for individuals diagnosed with HCC (103).
An open-label randomized trial demonstrated that EBRT with TACE for advanced HCC (with portal vein invasion) was well-tolerated and improved PFS and OS compared to sorafenib treatment (104). The trial explored the combination of EBRT with TACE vs. sorafenib in treatment naive HCC patients with macrovascular invasion. Ninety patients were randomized in a 1:1 fashion. The primary endpoint of the trial was 12-week PFS rate. In this head-to-head comparison, the PFS rate was significantly higher in the TACE-EBRT group (86.7% vs. 34.4%, P<0.001). Higher rates of radiologic response were also seen in the TACE-EBRT group when compared to sorafenib (33.3% vs. 2.2%) at the 24-week mark. Modified progression free survival (mPFS) and OS were 31 and 55 weeks for TACE-EBRT group and 11.7 and 43 weeks for sorafenib. Notably, patients with macrovascular invasion, a prognostic predictor of poor OS, were evaluated in this trial (104). The trial allowed for treatment crossover and were evaluated with intention-to-treat analysis. The superior mPFS of the TACE-EBRT group can make a case for treating patients initially with this combination prior to starting treatment with sorafenib. One limitation of the study is that the majority of the patients (85%) had hepatitis B associated HCC, and the results cannot be extrapolated to the general HCC population.
The recent results from the National Surgical Adjuvant Breast and Bowel Project (NSABP), the Radiation Therapy Oncology Group (RTOG), and the Gynecologic Oncology Group (GOG)/Radiation Therapy Oncology Group 1112 further indicate the role of combination strategies for treating HCC (108). The combination of sorafenib with SBRT vs. then standard-of-care sorafenib was evaluated in treatment naive advanced HCC patients. Trial accrual was closed early with new therapies approved for first line use in advanced HCC. Patients treated with SBRT + sorafenib had improved OS of 15.8 months (90% CI, 11.4–19.2) vs. 12.3 months (90% CI, 10.6–14.3) with sorafenib alone [hazard ratio (HR), 0.77; 90% CI, 0.59–1.01; 1-sided P=0.55]. Notably, 75% and 73% of patients in the SBRT/sorafenib and sorafenib monotherapy arms had macrovascular invasions, respectively. Also, the SBRT with sorafenib combination achieved a higher mPFS of 9.2 months (95% CI, 7.5–11.9) vs. 5.5 months (95% CI, 3.4–6.3) in those treated with sorafenib alone (HR, 0.55; 95% CI, 0.40–0.75; P=0.0001) (108). The improved PFS and OS might indicate future role of combination strategies involving RT with newly approved IO strategies in management of HCC (Table 3).
Symptom palliation with RT
The requirement for palliative care has been raised in cases of metastatic HCC, and advancements in technology have resulted in higher utilization of palliative RT (109). This approach is commonly pursued when no more effective systemic therapy exists. Initial studies on whole-liver RT at doses between 20–30 Gy for patients with mainly liver metastases demonstrated symptom palliation benefits for 49–95% of patients with minimal adverse effects (110-118). Most of these studies employed outdated radiation techniques, extended fractionation schedules, or concurrent radiosensitizers that are no longer commonly used in clinical practice (110). In one of the largest prospective studies of pain amelioration, patients with liver metastases were administered 21 Gy in 7 fractions to the whole liver and were randomized to misonidazole or placebo. This study found that patients with radiation and misonidazole had a response rate of improved abdominal pain of 87% compared to that of 74% in patients with radiation alone. The median response time was 13 weeks (117).
A prospective trial that studied 41 patients with liver metastases using 8 Gy in a single fraction to the whole liver showed improved average index symptom intensity in 48% of patients 1 month following RT. Still, benefits were observed only in patients with pain and abdominal discomfort. Treatment was well-tolerated, with only one patient developing grade 3 toxicity (119).
A similar result was seen in another single-site prospective study of 52 patients who received a single fraction (8 Gy) of palliative RT for abdominal discomfort due to unresectable HCC. More than 50% (51.9%) of patients experienced an improvement in their index symptoms after 1 month, with a median response duration of 89 days. The treatment was well tolerated, with only 3.8% of patients developing grade 3 gastrointestinal toxicities (110).
Not surprisingly, this is in line with another multi-center, phase III prospective study that shows that for patients with end-stage HCC or liver metastases, single-fraction radiation therapy (8 Gy) results in pain relief (worst pain) for the majority of patients and a potential improvement in survival (120).
RT and immunotherapy for intermediate stage in HCC (BCLC B)
With the emergence of ICIs, treatment options for advanced HCC have expanded beyond tyrosine kinase inhibitors (TKIs), such as sorafenib and lenvatinib, to include atezolizumab and bevacizumab based on the IMBRAVE150 study, and the STRIDE regimen based on HIMALAYA study (108,121-123).
There is growing interest in combining RT with immunotherapy in managing HCC. The immunomodulatory effects of RT have been well studied (124). RT enhances the infiltration of cytotoxic T cells within the tumor microenvironment. A robust and durable immune response can be obtained in combination with ICIs, which can potentially reverse the RT-mediated exhaustion pathways. Innate and adaptive immunity can also be activated when immuno-oncology (IO) is combined with low-dose RT, and a robust anti-tumor response can be obtained (125).
As mentioned before, RT can induce immunogenic cell death, release tumor antigens, and modulate the tumor microenvironment, while immunotherapy can enhance immune responses against cancer cells in HCC. The optimal dose, fractionation, and sequence of these treatment modalities are still being explored. The selection of dose and fractionation depends on various factors, including tumor size, location, and liver function. In general, lower doses of radiation (hypofractionation) are thought to be more immunogenic, potentially enhancing the immune response (126,127). However, higher doses (conventional fractionation) may also have immunomodulatory effects (127,128). The optimal approach may involve a balance between tumor control and immune activation, which requires further investigation in clinical trials specific to HCC.
Early results from several prospective trials evaluating RT and IO are discussed here. A phase II single-arm prospective study, sequential transarterial chemoembolization (TACE) and SBRT followed by IO as conversion therapy for patients with locally advanced HCC unsuitable for curative therapy (START-FIT), was conducted (129,130). The trial’s purpose was to evaluate the role of a combined modality approach for unresectable local HCC and make it amenable to curative treatment. Critical eligibility criteria included patients with HCC ≥5 cm, tumor nodules ≤3, and CPC A5-B7 liver function. Tumors with distant metastasis, main portal vein (VP4) invasion, or inferior vena cava (VV3) invasion were excluded. All enrolled patients underwent TACE on day 1, followed by 5 fractions of SBRT (27.5–40 Gy) on day 28. This treatment was followed by Avelumab (10 mg/kg) over 2 weeks. The study would be stopped if patients were deemed evaluable for surgery or had grade 3 or higher adverse events that required withdrawal or progression of the disease.
Thirty-three patients were enrolled in the study, with 32/33 male patients enrolled. The median sum of the diameter of the lesion(s) was 15.1 cm (range, 5.3–31.1 cm), 21 (63.6%) had macrovascular invasion (13 hepatic vein, 3 branched portal vein, 5 both) and 24 (73%) had hepatitis B. Most patients (21/33) patients were also BCLC C. After a median follow-up of 17.2 months (range, 3.5–31.6 months), 3 (9.1%) patients had tumor downstage with curative surgery done. Of the 33 patients, 18 were deemed amenable to curative treatment. Fourteen had a complete response (CR), and four had a partial response. Eight of the fifteen patients considered not amenable to curative therapy had a progression of the disease, and there was one grade 5 adverse event. The objective response rate (ORR) was 62.5% (95% CI, 45.3–77.1%), of whom 15 had a CR (43.8%), and 6 had a partial response (18.7%). The median OS and PFS were 30.3 months (95% CI: 22.7– not reached) and 20.7 months (95% CI: 14.6–26.8), respectively. The median time to progression was 21.4 months (95% CI: 16.4–26.4).
Eleven patients (33%) experienced ≥ grade 3 treatment-related adverse events, commonly transient increases in alanine transaminase (ALT)/aspartate aminotransferase (AST) (n=5, 15%) and bilirubin (n=2, 6%) levels after TACE. All patients recovered with conservative management (129). Five patients (15.2%) developed ≥ grade 3 immune-related adverse events (irAEs), primarily hepatitis and dermatitis. Two patients had irAEs which led to discontinuation of avelumab. However, 55% (18/33) of patients were amenable to curative treatment, with 12% undergoing curative treatment and 42% having radiographic CR without surgery, with an OS rate of 92% (129). These results warrant further exploration of a multimodality approach for successfully treating locally advanced unresectable HCC. The effectiveness of LC achieved through TACE and SBRT is further compounded by the addition of immunotherapy.
RT and immunotherapy for late-stage HCC (BCLC C)
First line therapy
A single-site retrospective case series reported impressive results on five patients (ECPG PS 0–2) with unresectable CPC A and B HCC with SBRT followed by anti-PD1 antibodies (nivolumab or pembrolizumab). All five patients responded to treatment, with two complete and three partial responses. Three patients had underlying hepatitis B, and 4 of them had BCLC C disease. The median tumor size was 9.8 cm (8.5–16.1 cm), with two patients having tumor vascular thrombosis and 1 having extrahepatic disease. No patients progressed on treatment during a median follow-up of 14.9 months (8.6–19 months). The combination also helped downstage the tumor, making it amendable to RFA, as reported in 1 partial responder. One patient had grade ≥3 toxicities (pneumonitis and skin reaction). No patient had grade >3 hepatotoxicity (131). The effective local tumor control obtained through a combination of SBRT with PD-1 inhibitors warranted further exploration of this combination in future, more extensive prospective studies.
Another phase I trial was conducted, which evaluated SBRT (40 Gy in 5 fractions) followed by either ipilimumab/nivolumab or nivolumab alone. This trial is the first prospective study assessing the role of immunotherapy with SBRT in advanced or unresectable HCC. The trial was closed early due to slow accrual. Fourteen patients were enrolled, with thirteen evaluable. Six patients were allocated to the nivolumab arm, whereas the remaining eight were assigned to the ipilimumab/nivolumab arm. Most patients were male (11/13), and the median age was 67 years (63-71). All patients had CPC A classification, and the most common etiology of cirrhosis was hepatitis C (50%), followed by alcohol use (36%). Two patients received prior systemic therapy with TKIs. Four enrolled patients had extrahepatic disease, and four patients had tumor thrombus. All patients underwent liver SBRT, delivering 40 Gy in 5 fractions (132). The primary endpoint of the study was dose-limiting toxicity (DLT). Two patients had DLT, with one each observed in the ipilimumab/nivolumab arm and nivolumab alone arm within 6 months of SBRT. There were no grade 4 or 5 toxicities. Grade 3 adverse events occurred in 8 patients, 3 in the nivolumab alone arm and 5 in the ipilimumab/nivolumab arm. These included AST/ALT elevations, lipase elevations, thrombocytopenia, hyponatremia, fatigue, and generalized muscle weakness. Grade 3 hepatotoxicity events were AST/ALT elevations and occurred in 4 (30.8%) of 13 patients, 1 (16.7%) of 6 patients, and 3 (42.9%) of 7 patients in the respective cohorts (132).
Additionally, after a median follow-up of 42.1 months, the ORR was 57% (4 of 7 patients; 90% CI, 23–87%) in the ipilimumab/nivolumab arm compared to 0% (0 of 6 patients; 90% CI, 0–39%) in the nivolumab alone arm. All four patients had a partial response to treatment. The study reported the following: median PFS of 11.6 months (90% CI, 4.5 to not reached) versus 2.7 months (90% CI, 1.3–4.7), and median OS of 41.6 months (90% CI, 4.5 to not reached) versus 4.7 months (90% CI, 2.0–16.2). In addition, the ipilimumab/nivolumab arm showed more grade 3 hepatotoxicity (4 vs. 1 patient in the nivolumab arm) (132). The small sample size of 14 patients split into two arms is a key limitation of this study. Still, it offers safety and efficacy data on the potential efficacy of this combination of RT and ipilimumab/nivolumab. Further studies should be done to explore the role of this combination.
Second line therapy
Camrelizumab, in combination with SBRT, in a phase IIA trial, was also studied to evaluate the potential efficacy and toxicity of the combination in patients with advanced HCC who had progressed on prior systemic therapy. SBRT with administered with 30–50 Gy over 10 fractions (133,134). Camrelizumab 200 mg was given intravenously every 3 weeks since the first day of RT until disease progression or intolerable toxicity. Seventeen patients were enrolled in the study (median age 54 years; range, 32–69 years). Fifteen (88%) patients were male. Fourteen (82%) were ECOG 0. All patients had CPC A and mainly had BCLC C (16/17). Thirteen patients had a history of hepatitis B. Extrahepatic metastases were present in 11 patients (65%). Fifteen patients previously had >2 lines of prior therapy. The ORR was 47%. The best response assessed by RECIST 1.1 was a partial response (8 patients). Four patients had grade 3 irAEs, including elevated LFTs (n=1), decreased hemoglobin (n=1), reduced platelet count (n=1), and decreased neutrophil count (n=1). All grade 3 irAEs were mitigated with proper treatment. No treatment-related deaths occurred during the study (133). This study’s high ORR of 47% in the 2nd line setting of HCC highlighted the vital role of combined SBRT and PD-1 blockade approach in managing HCC.
Ongoing clinical studies for RT with PD-1 and PD-L1 inhibitors in HCCs (Table 4)
Table 4
Trial phase | NCT number | HCC patient population | Treatment arms | SBRT dose | Primary endpoint | Secondary endpoints |
---|---|---|---|---|---|---|
IB | NCT05096715 (135) | Unresectable | Atezolizumab + bevacizumab + SBRT (1–3 days of C1), followed by atezolizumab + bevacizumab | 20 Gy | DLT | PFS, OS, in-field RR, out of field RR, and change in CP score |
I | NCT05488522 (136) | Unresectable | Atezolizumab + bevacizumab + SBRT | 17 Gy (max 3 fractions) of SBRT at 4-week intervals | DLT | ORR, OS, PFS |
I | NCT03203304 (completed; terminated early because of poor enrollment) (132) | Unresectable | SBRT + nivolumab +/− ipilimumab | 40 Gy in 5 fractions | DLT | ORR, OS, PFS, AE rate, LC |
II | NCT04913480 (137) | Unresectable | SBRT + durvalumab | 27.5–50 Gy/5 fractions | PFS | OS, ORR, LC, LFR, AE rate, change in PD-L1 before and after RT |
II | NCT03316872 (138) | Advanced disease after progression on sorafenib | SBRT + pembrolizumab | 5 fractions | ORR | PFS, OS, RR in non-irradiated tumors |
II | NCT03817736 (129) unresectable hepatocellular carcinoma (START-FIT) | Locally advanced disease for downstaging | SBRT + TACE + Avelumab | 27.5–40.0 Gy/5 fractions | No patients downstaged for curative resection | OS, PFS, TTP |
II | NCT03857815 (139) | Recurrent or oligometastatic disease | SBRT + sintilimab | 54 Gy (48–60 Gy)/6 fractions | PFS | ORR, OS, AE |
RT, radiotherapy; HCC, hepatocellular carcinoma; SBRT, stereotactic body radiotherapy; DLT, dose-limiting toxicity; PFS, progression-free survival; OS, overall survival; RR, response rate; CP, Child-Pugh; ORR, overall response rate; AE, adverse event; LC, local control, LFR, liver failure-free, PD-L1, programmed death-ligand 1; TACE, transarterial chemoembolization; TTP, time to progression.
An open-label, multi-center study currently aims to assess the efficacy and safety of combining pembrolizumab and 90Y radioembolization in patients with high-risk HCC who are not eligible for liver transplantation or surgical resection and have a well-compensated liver function. The treatment protocol involves administering pembrolizumab 200 mg intravenously every 3 weeks, along with 90Y radioembolization performed 1 week after the initial pembrolizumab dose. In cases of bilobar disease, a second 90Y radioembolization will be conducted no later than 4 weeks following the first procedure, targeting the contralateral hepatic lobe (Table 4) (140).
Two phase I trials (NCT05488522 and NCT05096715) are currently evaluating the role of concurrent SBRT with atezolizumab, a PD-L1 inhibitor, and bevacizumab with SBRT for patients with advanced HCC deemed not amenable to curative treatment (135,141). The primary endpoint of the study is to establish dose-limiting toxicity. Secondary endpoints are OS, PFS, ORR, and toxicity rates. Trials evaluating other ICIs such as durvalumab (NCT04913480) and pembrolizumab (NCT03316872) with SBRT are also currently underway (138,142) (Table 4).
Conclusions
The management of HCC has seen a remarkable evolution over the years. A combined modality approach has become increasingly common, with several treatment strategies available to treat the disease medically and surgically. RT has shown a role in downstaging unresectable HCC and potentially achieving disease control. Based on recent technological advances, the combination of immunotherapy and RT has emerged as a promising approach for the treatment of unresectable HCC. The combination approach of RT and immunotherapy has shown promising results, with several trials evaluating the optimal timing, dose, and sequence of these therapies. In addition, combination therapies involving ICIs have emerged as potential treatment options for advanced HCC, with several immunotherapeutic agents also in development for their potential role in treating HCC. Future studies would include well-designed clinical trials using RT and IO combinations such as PD-1 inhibitor + CTLA 4 antibody, PD-1 inhibitor combined with lymphocyte activation gene 3 (LAG 3) inhibitor, or PD-1 inhibitor + VEGF inhibitor.
Acknowledgments
Funding: None.
Footnote
Peer Review File: Available at https://cco.amegroups.com/article/view/10.21037/cco-23-50/prf
Conflicts of Interest: All authors have completed the ICMJE uniform disclosure form (available at https://cco.amegroups.com/article/view/10.21037/cco-23-50/coif). The authors have no conflicts of interest to declare.
Ethical Statement: The authors are accountable for all aspects of the work in ensuring that questions related to the accuracy or integrity of any part of the work are appropriately investigated and resolved.
Open Access Statement: This is an Open Access article distributed in accordance with the Creative Commons Attribution-NonCommercial-NoDerivs 4.0 International License (CC BY-NC-ND 4.0), which permits the non-commercial replication and distribution of the article with the strict proviso that no changes or edits are made and the original work is properly cited (including links to both the formal publication through the relevant DOI and the license). See: https://creativecommons.org/licenses/by-nc-nd/4.0/.
References
- Bray F, Ferlay J, Soerjomataram I, et al. Global cancer statistics 2018: GLOBOCAN estimates of incidence and mortality worldwide for 36 cancers in 185 countries. CA Cancer J Clin 2018;68:394-424. [Crossref] [PubMed]
- Llovet JM, Zucman-Rossi J, Pikarsky E, et al. Hepatocellular carcinoma. Nat Rev Dis Primers 2016;2:16018. [Crossref] [PubMed]
- El-Serag HB. Epidemiology of viral hepatitis and hepatocellular carcinoma. Gastroenterology 2012;142:1264-1273.e1. [Crossref] [PubMed]
- Sung H, Ferlay J, Siegel RL, et al. Global Cancer Statistics 2020: GLOBOCAN Estimates of Incidence and Mortality Worldwide for 36 Cancers in 185 Countries. CA Cancer J Clin 2021;71:209-49. [Crossref] [PubMed]
- Altekruse SF, Henley SJ, Cucinelli JE, et al. Changing hepatocellular carcinoma incidence and liver cancer mortality rates in the United States. Am J Gastroenterol 2014;109:542-53. [Crossref] [PubMed]
- Ashtari S, Pourhoseingholi MA, Sharifian A, et al. Hepatocellular carcinoma in Asia: Prevention strategy and planning. World J Hepatol 2015;7:1708-17. [Crossref] [PubMed]
- Arbuthnot P, Kew M. Hepatitis B virus and hepatocellular carcinoma. Int J Exp Pathol 2001;82:77-100. [Crossref] [PubMed]
- Estes C, Razavi H, Loomba R, et al. Modeling the epidemic of nonalcoholic fatty liver disease demonstrates an exponential increase in burden of disease. Hepatology 2018;67:123-33. [Crossref] [PubMed]
- Wang Z, Budhu AS, Shen Y, et al. Genetic susceptibility to hepatocellular carcinoma in chromosome 22q13.31, findings of a genome-wide association study. JGH Open 2021;5:1363-72. [Crossref] [PubMed]
- Tsilimigras DI, Aziz H, Pawlik TM. Critical Analysis of the Updated Barcelona Clinic Liver Cancer (BCLC) Group Guidelines. Ann Surg Oncol 2022;29:7231-4. [Crossref] [PubMed]
- Raza A, Sood GK. Hepatocellular carcinoma review: current treatment, and evidence-based medicine. World J Gastroenterol 2014;20:4115-27. [Crossref] [PubMed]
- Karademir S. Staging of hepatocellular carcinoma. Hepatoma Res 2018;4:58.
- Zhong BY, Jin ZC, Chen JJ, et al. Role of Transarterial Chemoembolization in the Treatment of Hepatocellular Carcinoma. J Clin Transl Hepatol 2023;11:480-9. [Crossref] [PubMed]
- Wu FX, Chen J, Bai T, et al. The safety and efficacy of transarterial chemoembolization combined with sorafenib and sorafenib mono-therapy in patients with BCLC stage B/C hepatocellular carcinoma. BMC Cancer 2017;17:645. [Crossref] [PubMed]
- Golfieri R, Bargellini I, Spreafico C, et al. Patients with Barcelona Clinic Liver Cancer Stages B and C Hepatocellular Carcinoma: Time for a Subclassification. Liver Cancer 2019;8:78-91. [Crossref] [PubMed]
- Sur BW, Sharma A. Transarterial chemoembolization for hepatocellular carcinoma. J Radiol Nurs 2018;37:107-11.
- Han K, Kim JH. Transarterial chemoembolization in hepatocellular carcinoma treatment: Barcelona clinic liver cancer staging system. World J Gastroenterol 2015;21:10327-35. [Crossref] [PubMed]
- Bucalau AM, Tancredi I, Verset G. In the Era of Systemic Therapy for Hepatocellular Carcinoma Is Transarterial Chemoembolization Still a Card to Play? Cancers (Basel) 2021;13:5129. [Crossref] [PubMed]
- Reig M, Forner A, Rimola J, et al. BCLC strategy for prognosis prediction and treatment recommendation: The 2022 update. J Hepatol 2022;76:681-93. [Crossref] [PubMed]
- Abou-Alfa GK, Lau G, Kudo M, et al. Tremelimumab plus durvalumab in unresectable hepatocellular carcinoma. NEJM Evidence 2022;1:EVIDoa2100070.
- Jain A, Chitturi S, Peters G, et al. Atezolizumab and bevacizumab as first line therapy in advanced hepatocellular carcinoma: Practical considerations in routine clinical practice. World J Hepatol 2021;13:1132-42. [Crossref] [PubMed]
- Kudo M. Durvalumab plus tremelimumab in unresectable hepatocellular carcinoma. Hepatobiliary Surg Nutr 2022;11:592-6. [Crossref] [PubMed]
- Fong KY, Zhao JJ, Sultana R, et al. First-Line Systemic Therapies for Advanced Hepatocellular Carcinoma: A Systematic Review and Patient-Level Network Meta-Analysis. Liver Cancer 2023;12:7-18. [Crossref] [PubMed]
- Choi WM, Lee D, Shim JH, et al. Effectiveness and Safety of Nivolumab in Child-Pugh B Patients with Hepatocellular Carcinoma: A Real-World Cohort Study. Cancers (Basel) 2020;12:1968. [Crossref] [PubMed]
- Marrero JA, Kudo M, Venook AP, et al. Observational registry of sorafenib use in clinical practice across Child-Pugh subgroups: The GIDEON study. J Hepatol 2016;65:1140-7. [Crossref] [PubMed]
- Pressiani T, Boni C, Rimassa L, et al. Sorafenib in patients with Child-Pugh class A and B advanced hepatocellular carcinoma: a prospective feasibility analysis. Ann Oncol 2013;24:406-11. [Crossref] [PubMed]
- Khalaf N, Ying J, Mittal S, et al. Natural History of Untreated Hepatocellular Carcinoma in a US Cohort and the Role of Cancer Surveillance. Clin Gastroenterol Hepatol 2017;15:273-281.e1. [Crossref] [PubMed]
- Zhuo Y, Chen Q, Chhatwal J. Changing Epidemiology of Hepatocellular Carcinoma and Role of Surveillance. In: Hoshida Y. editor. Hepatocellular carcinoma: translational precision medicine approaches. Cham (CH): Humana Cham, 2019:53-67.
- Singal AG, Parikh ND, Rich NE, et al. Hepatocellular carcinoma surveillance and staging. In: Hoshida Y. editor. Hepatocellular carcinoma: translational precision medicine approaches. Cham (CH): Humana Cham, 2019:27-51.
- Ohri N, Dawson LA, Krishnan S, et al. Radiotherapy for Hepatocellular Carcinoma: New Indications and Directions for Future Study. J Natl Cancer Inst 2016;108:djw133. [Crossref] [PubMed]
- Parikh ND, Cuneo K, Mendiratta-Lala M. Radiation Therapies for the Treatment of Hepatocellular Carcinoma. Clin Liver Dis (Hoboken) 2021;17:341-6. [Crossref] [PubMed]
- Manzar GS, De BS, Abana CO, et al. Outcomes and Toxicities of Modern Combined Modality Therapy with Atezolizumab Plus Bevacizumab and Radiation Therapy for Hepatocellular Carcinoma. Cancers (Basel) 2022;14:1901. [Crossref] [PubMed]
- Wei L, Simeth J, Aryal MP, et al. The Effect of Stereotactic Body Radiation Therapy for Hepatocellular Cancer on Regional Hepatic Liver Function. Int J Radiat Oncol Biol Phys 2023;115:794-802. [Crossref] [PubMed]
- Rim CH, Lee JS, Kim SY, et al. Comparison of radiofrequency ablation and ablative external radiotherapy for the treatment of intrahepatic malignancies: A hybrid meta-analysis. JHEP Rep 2023;5:100594. [Crossref] [PubMed]
- Sharma R, Pillai A, Marron TU, et al. Patterns and outcomes of subsequent therapy after immune checkpoint inhibitor discontinuation in HCC. Hepatol Commun 2022;6:1776-85. [Crossref] [PubMed]
- Emami B. Tolerance of normal tissue to therapeutic radiation. Rep Radiother Oncol 2013;1:123-7.
- Chang DS, Lasley FD, Das IJ, et al. Normal tissue radiation responses. In: Basic Radiotherapy Physics and Biology. Cham: Springer, 2014:265-275.
- Kalogeridi MA, Zygogianni A, Kyrgias G, et al. Role of radiotherapy in the management of hepatocellular carcinoma: A systematic review. World J Hepatol 2015;7:101-12. [Crossref] [PubMed]
- Meyer J, Singal AG. Stereotactic ablative radiotherapy for hepatocellular carcinoma: History, current status, and opportunities. Liver Transpl 2018;24:420-7. [Crossref] [PubMed]
- Eriksson D, Stigbrand T. Radiation-induced cell death mechanisms. Tumour Biol 2010;31:363-72. [Crossref] [PubMed]
- Folkert MR, Timmerman RD. Stereotactic ablative body radiosurgery (SABR) or Stereotactic body radiation therapy (SBRT). Adv Drug Deliv Rev 2017;109:3-14. [Crossref] [PubMed]
- Siva S, MacManus MP, Martin RF, et al. Abscopal effects of radiation therapy: a clinical review for the radiobiologist. Cancer Lett 2015;356:82-90. [Crossref] [PubMed]
- Demaria S, Ng B, Devitt ML, et al. Ionizing radiation inhibition of distant untreated tumors (abscopal effect) is immune mediated. Int J Radiat Oncol Biol Phys 2004;58:862-70. [Crossref] [PubMed]
- Postow MA, Callahan MK, Barker CA, et al. Immunologic correlates of the abscopal effect in a patient with melanoma. N Engl J Med 2012;366:925-31. [Crossref] [PubMed]
- Demaria S, Formenti SC. The abscopal effect 67 years later: from a side story to center stage. Br J Radiol 2020;93:20200042. [Crossref] [PubMed]
- Demaria S, Golden EB, Formenti SC. Role of Local Radiation Therapy in Cancer Immunotherapy. JAMA Oncol 2015;1:1325-32. [Crossref] [PubMed]
- Liu Y, Dong Y, Kong L, et al. Abscopal effect of radiotherapy combined with immune checkpoint inhibitors. J Hematol Oncol 2018;11:104. [Crossref] [PubMed]
- Boone BA, Lotze MT. Targeting damage-associated molecular pattern molecules (DAMPs) and DAMP receptors in melanoma. Methods Mol Biol 2014;1102:537-52. [Crossref] [PubMed]
- Zamora AE, Crawford JC, Thomas PG. Hitting the Target: How T Cells Detect and Eliminate Tumors. J Immunol 2018;200:392-9. [Crossref] [PubMed]
- Zhang Z, Lu M, Qin Y, et al. Neoantigen: A New Breakthrough in Tumor Immunotherapy. Front Immunol 2021;12:672356. [Crossref] [PubMed]
- Bian Z, Shi L, Kidder K, et al. Intratumoral SIRPα-deficient macrophages activate tumor antigen-specific cytotoxic T cells under radiotherapy. Nat Commun 2021;12:3229. [Crossref] [PubMed]
- Vaes RDW, Hendriks LEL, Vooijs M, et al. Biomarkers of Radiotherapy-Induced Immunogenic Cell Death. Cells 2021;10:930. [Crossref] [PubMed]
- Bao X, Xie L. Targeting purinergic pathway to enhance radiotherapy-induced immunogenic cancer cell death. J Exp Clin Cancer Res 2022;41:222. [Crossref] [PubMed]
- Yau T, Kang YK, Kim TY, et al. Efficacy and Safety of Nivolumab Plus Ipilimumab in Patients With Advanced Hepatocellular Carcinoma Previously Treated With Sorafenib: The CheckMate 040 Randomized Clinical Trial. JAMA Oncol 2020;6:e204564. [Crossref] [PubMed]
- Guo J, Li L, Guo B, et al. Mechanisms of resistance to chemotherapy and radiotherapy in hepatocellular carcinoma. Transl Cancer Res 2018;7:765-81.
- Ngwa W, Irabor OC, Schoenfeld JD, et al. Using immunotherapy to boost the abscopal effect. Nat Rev Cancer 2018;18:313-22. [Crossref] [PubMed]
- Rodríguez-Ruiz ME, Vanpouille-Box C, Melero I, et al. Immunological Mechanisms Responsible for Radiation-Induced Abscopal Effect. Trends Immunol 2018;39:644-55. [Crossref] [PubMed]
- Abuodeh Y, Venkat P, Kim S. Systematic review of case reports on the abscopal effect. Curr Probl Cancer 2016;40:25-37. [Crossref] [PubMed]
- Twyman-Saint Victor C, Rech AJ, Maity A, et al. Radiation and dual checkpoint blockade activate non-redundant immune mechanisms in cancer. Nature 2015;520:373-7. [Crossref] [PubMed]
- Hodge JW, Sharp HJ, Gameiro SR. Abscopal regression of antigen disparate tumors by antigen cascade after systemic tumor vaccination in combination with local tumor radiation. Cancer Biother Radiopharm 2012;27:12-22. [Crossref] [PubMed]
- Vatner RE, Cooper BT, Vanpouille-Box C, et al. Combinations of immunotherapy and radiation in cancer therapy. Front Oncol 2014;4:325. [Crossref] [PubMed]
- Kim KJ, Kim JH, Lee SJ, et al. Radiation improves antitumor effect of immune checkpoint inhibitor in murine hepatocellular carcinoma model. Oncotarget 2017;8:41242-55. [Crossref] [PubMed]
- Jung J, Kim H, Yoon SM, et al. Targeting Accuracy of Image-Guided Stereotactic Body Radiation Therapy for Hepatocellular Carcinoma in Real-Life Clinical Practice: In Vivo Assessment Using Hepatic Parenchymal Changes on Gd-EOB-DTPA-Enhanced Magnetic Resonance Images. Int J Radiat Oncol Biol Phys 2018;102:867-74. [Crossref] [PubMed]
- Chen W, Chiang CL, Dawson LA. Efficacy and safety of radiotherapy for primary liver cancer. Chin Clin Oncol 2021;10:9. [Crossref] [PubMed]
- Yu JI, Park HC. Radiotherapy as valid modality for hepatocellular carcinoma with portal vein tumor thrombosis. World J Gastroenterol 2016;22:6851-63. [Crossref] [PubMed]
- Kurup G. CyberKnife: A new paradigm in radiotherapy. J Med Phys 2010;35:63-4. [Crossref] [PubMed]
- Fuller DB. CyberKnife Radiosurgery: Quality of Life and Cost Considerations. In: Urschel HC, Kresl JJ, Luketich JD, et al. editors. Treating Tumors that Move with Respiration. Heidelberg: Springer Berlin Heidelberg; 2007:283-293.
- Marqueen KE, Kim E, Ang C, et al. Cost-Effectiveness Analysis of Selective Internal Radiotherapy With Yttrium-90 Versus Sorafenib in Locally Advanced Hepatocellular Carcinoma. JCO Oncol Pract 2021;17:e266-77. [Crossref] [PubMed]
- Lazar AA, Schulte R, Faddegon B, et al. Clinical trials involving carbon-ion radiation therapy and the path forward. Cancer 2018;124:4467-76. [Crossref] [PubMed]
- Paganetti H. Proton beam therapy. Bristol, UK: IOP Publishing Bristol; 2017.
- Kwon JH, Bae SH, Kim JY, et al. Long-term effect of stereotactic body radiation therapy for primary hepatocellular carcinoma ineligible for local ablation therapy or surgical resection. Stereotactic radiotherapy for liver cancer. BMC Cancer 2010;10:475. [Crossref] [PubMed]
- Koka K, Verma A, Dwarakanath BS, et al. Technological Advancements in External Beam Radiation Therapy (EBRT): An Indispensable Tool for Cancer Treatment. Cancer Manag Res 2022;14:1421-9. [Crossref] [PubMed]
- Curley SA, Stuart KE, Schwartz JM, et al. Localized hepatocellular carcinoma: Liver-directed therapies for nonsurgical candidates not eligible for local thermal ablation. In: Tanabe KK, Willett CG, eds. UpToDate, Post TW (Ed). 2022. Accessed 7/2/2023. Available online: https://www.uptodate.com/contents/localized-hepatocellular-carcinoma-liver-directed-therapies-for-nonsurgical-candidates-not-eligible-for-local-thermal-ablation?search=sbrt&source=search_result&selectedTitle=8~64&usage_type=default&display_rank=8
- Garin E, Rolland Y, Edeline J. (90)Y-Loaded Microsphere SIRT of HCC Patients With Portal Vein Thrombosis: High Clinical Impact of 99mTc-MAA SPECT/CT-Based Dosimetry. Semin Nucl Med 2019;49:218-26. [Crossref] [PubMed]
- Ebner DK, Kamada T. The Emerging Role of Carbon-Ion Radiotherapy. Front Oncol 2016;6:140. [Crossref] [PubMed]
- Halpern JA, Sedrakyan A, Hsu WC, et al. Use, complications, and costs of stereotactic body radiotherapy for localized prostate cancer. Cancer 2016;122:2496-504. [Crossref] [PubMed]
- Rim CH, Yoon WS. Leaflet manual of external beam radiation therapy for hepatocellular carcinoma: a review of the indications, evidences, and clinical trials. Onco Targets Ther 2018;11:2865-74. [Crossref] [PubMed]
- Gerum S, Jensen AD, Roeder F. Stereotactic body radiation therapy in patients with hepatocellular carcinoma: A mini-review. World J Gastrointest Oncol 2019;11:367-76. [Crossref] [PubMed]
- Zeng M, Han LF. Stereotactic radiosurgery: a “targeted” therapy for cancer. Chin J Cancer 2012;31:471-5. [Crossref] [PubMed]
- Klein J, Dawson LA. Hepatocellular carcinoma radiation therapy: review of evidence and future opportunities. Int J Radiat Oncol Biol Phys 2013;87:22-32. [Crossref] [PubMed]
- Yu JI, Park JW, Park HC, et al. Clinical impact of combined transarterial chemoembolization and radiotherapy for advanced hepatocellular carcinoma with portal vein tumor thrombosis: An external validation study. Radiother Oncol 2016;118:408-15. [Crossref] [PubMed]
- Cha H, Park HC, Yu JI, et al. Clinical Practice Patterns of Radiotherapy in Patients with Hepatocellular Carcinoma: A Korean Radiation Oncology Group Study (KROG 14-07). Cancer Res Treat 2017;49:61-9. [Crossref] [PubMed]
- Kim N, Kim HJ, Won JY, et al. Retrospective analysis of stereotactic body radiation therapy efficacy over radiofrequency ablation for hepatocellular carcinoma. Radiother Oncol 2019;131:81-7. [Crossref] [PubMed]
- Wahl DR, Stenmark MH, Tao Y, et al. Outcomes After Stereotactic Body Radiotherapy or Radiofrequency Ablation for Hepatocellular Carcinoma. J Clin Oncol 2016;34:452-9. [Crossref] [PubMed]
- Rompianesi G. Editorial: Radiofrequency ablation in liver cancers: Investigations of efficacy as monotherapy and polytherapy. Front Oncol 2022;12:1088012. [Crossref] [PubMed]
- Pan YX, Fu YZ, Hu DD, et al. Stereotactic Body Radiotherapy vs. Radiofrequency Ablation in the Treatment of Hepatocellular Carcinoma: A Meta-Analysis. Front Oncol 2020;10:1639. [Crossref] [PubMed]
- Kim TH, Koh YH, Kim BH, et al. Proton beam radiotherapy vs. radiofrequency ablation for recurrent hepatocellular carcinoma: A randomized phase III trial. J Hepatol 2021;74:603-12. [Crossref] [PubMed]
- Kim TH, Kim BH, Park JW, et al. Proton Beam Therapy for Treatment-Naïve Hepatocellular Carcinoma and Prognostic Significance of Albumin-Bilirubin (ALBI) Grade. Cancers (Basel) 2022;14:4445. [Crossref] [PubMed]
- Fukuda K, Okumura T, Abei M, et al. Long-term outcomes of proton beam therapy in patients with previously untreated hepatocellular carcinoma. Cancer Sci 2017;108:497-503. [Crossref] [PubMed]
- Shiba S, Shibuya K, Katoh H, et al. A comparison of carbon ion radiotherapy and transarterial chemoembolization treatment outcomes for single hepatocellular carcinoma: a propensity score matching study. Radiat Oncol 2019;14:137. [Crossref] [PubMed]
- Sapir E, Tao Y, Schipper MJ, et al. Stereotactic Body Radiation Therapy as an Alternative to Transarterial Chemoembolization for Hepatocellular Carcinoma. Int J Radiat Oncol Biol Phys 2018;100:122-30. [Crossref] [PubMed]
- Sapisochin G, Barry A, Doherty M, et al. Stereotactic body radiotherapy vs. TACE or RFA as a bridge to transplant in patients with hepatocellular carcinoma. An intention-to-treat analysis. J Hepatol 2017;67:92-9. [Crossref] [PubMed]
- Jensen RT. Gastrointestinal Neuroendocrine Tumor Syndromes (GI NETS). In: Huhtaniemi I, Martini L. editors. Encyclopedia of Endocrine Diseases (Second Edition). Cambridge, USA: Academic Press; 2018;1:597-616.
- Ricke J, Klümpen HJ, Amthauer H, et al. Impact of combined selective internal radiation therapy and sorafenib on survival in advanced hepatocellular carcinoma. J Hepatol 2019;71:1164-74. [Crossref] [PubMed]
- Houle S, Yip TK, Shepherd FA, et al. Hepatocellular carcinoma: pilot trial of treatment with Y-90 microspheres. Radiology 1989;172:857-60. [Crossref] [PubMed]
- Ahmadzadehfar H, Biersack HJ, Ezziddin S. Radioembolization of liver tumors with yttrium-90 microspheres. Semin Nucl Med 2010;40:105-21. [Crossref] [PubMed]
- Vouche M, Habib A, Ward TJ, et al. Unresectable solitary hepatocellular carcinoma not amenable to radiofrequency ablation: multicenter radiology-pathology correlation and survival of radiation segmentectomy. Hepatology 2014;60:192-201. [Crossref] [PubMed]
- Kang JK, Kim MS, Cho CK, et al. Stereotactic body radiation therapy for inoperable hepatocellular carcinoma as a local salvage treatment after incomplete transarterial chemoembolization. Cancer 2012;118:5424-31. [Crossref] [PubMed]
- Andolino DL, Johnson CS, Maluccio M, et al. Stereotactic body radiotherapy for primary hepatocellular carcinoma. Int J Radiat Oncol Biol Phys 2011;81:e447-53. [Crossref] [PubMed]
- Huang WY, Jen YM, Lee MS, et al. Stereotactic body radiation therapy in recurrent hepatocellular carcinoma. Int J Radiat Oncol Biol Phys 2012;84:355-61. [Crossref] [PubMed]
- Bujold A, Massey CA, Kim JJ, et al. Sequential phase I and II trials of stereotactic body radiotherapy for locally advanced hepatocellular carcinoma. J Clin Oncol 2013;31:1631-9. [Crossref] [PubMed]
- Sanuki N, Takeda A, Oku Y, et al. Stereotactic body radiotherapy for small hepatocellular carcinoma: a retrospective outcome analysis in 185 patients. Acta Oncol 2014;53:399-404. [Crossref] [PubMed]
- Jang WI, Kim MS, Bae SH, et al. High-dose stereotactic body radiotherapy correlates increased local control and overall survival in patients with inoperable hepatocellular carcinoma. Radiat Oncol 2013;8:250. [Crossref] [PubMed]
- Yoon SM, Ryoo BY, Lee SJ, et al. Efficacy and Safety of Transarterial Chemoembolization Plus External Beam Radiotherapy vs Sorafenib in Hepatocellular Carcinoma With Macroscopic Vascular Invasion: A Randomized Clinical Trial. JAMA Oncol 2018;4:661-9. [Crossref] [PubMed]
- Chen CP. Role of Radiotherapy in the Treatment of Hepatocellular Carcinoma. J Clin Transl Hepatol 2019;7:183-90. [Crossref] [PubMed]
- Daher S, Massarwa M, Benson AA, et al. Current and Future Treatment of Hepatocellular Carcinoma: An Updated Comprehensive Review. J Clin Transl Hepatol 2018;6:69-78. [Crossref] [PubMed]
- European Association for Study of Liver. EASL-EORTC clinical practice guidelines: management of hepatocellular carcinoma. Eur J Cancer 2012;48:599-641. Erratum in: Eur J Cancer 2012;48:1255-6. [Crossref] [PubMed]
- Dawson LA, Winter K, Knox J, et al. NRG/RTOG 1112: Randomized Phase III Study of Sorafenib vs. Stereotactic Body Radiation Therapy (SBRT) Followed by Sorafenib in Hepatocellular Carcinoma (HCC) (NCT01730937). Int J Radiat Oncol Biol Phys 2022;114:1057.
- Yoo GS, Park HC, Yu JI, et al. Stereotactic ablative body radiotherapy for spinal metastasis from hepatocellular carcinoma: its oncologic outcomes and risk of vertebral compression fracture. Oncotarget 2017;8:72860-71. [Crossref] [PubMed]
- Yeung CSY, Chiang CL, Wong NSM, et al. Palliative Liver Radiotherapy (RT) for Symptomatic Hepatocellular Carcinoma (HCC). Sci Rep 2020;10:1254. [Crossref] [PubMed]
- Ingold JA, Reed GB, Kaplan HS, et al. Radiation hepatitis. Am J Roentgenol Radium Ther Nucl Med 1965;93:200-8.
- Wharton JT, Delclos L, Gallager S, et al. Radiation hepatitis induced by abdominal irradiation with the cobalt 60 moving strip technique. Am J Roentgenol Radium Ther Nucl Med 1973;117:73-80. [Crossref] [PubMed]
- Sherman DM, Weichselbaum R, Order SE, et al. Palliation of hepatic metastasis. Cancer 1978;41:2013-7. [Crossref]
- Borgelt BB, Gelber R, Brady LW, et al. The palliation of hepatic metastases: results of the Radiation Therapy Oncology Group pilot study. Int J Radiat Oncol Biol Phys 1981;7:587-91. [Crossref] [PubMed]
- Prasad B, Lee MS, Hendrickson FR. Irradiation of hepatic metastases. Int J Radiat Oncol Biol Phys 1977;2:129-32. [Crossref]
- Turek-Maischeider M, Kazem I. Palliative irradiation for liver metastases. JAMA 1975;232:625-8.
- Leibel SA, Pajak TF, Massullo V, et al. A comparison of misonidazole sensitized radiation therapy to radiation therapy alone for the palliation of hepatic metastases: results of a Radiation Therapy Oncology Group randomized prospective trial. Int J Radiat Oncol Biol Phys 1987;13:1057-64. [Crossref] [PubMed]
- Bydder S, Spry NA, Christie DR, et al. A prospective trial of short-fractionation radiotherapy for the palliation of liver metastases. Australas Radiol 2003;47:284-8. [Crossref] [PubMed]
- Soliman H, Ringash J, Jiang H, et al. Phase II trial of palliative radiotherapy for hepatocellular carcinoma and liver metastases. J Clin Oncol 2013;31:3980-6. [Crossref] [PubMed]
- Dawson LA, Fairchild AM, Dennis K, et al. Canadian Cancer Trials Group HE. 1: A phase III study of palliative radiotherapy for symptomatic hepatocellular carcinoma and liver metastases. J Clin Oncol 2023;41:abstr LBA492.
- Abou-Alfa GK, Chan SL, Kudo M, et al. Phase 3 randomized, open-label, multicenter study of tremelimumab (T) and durvalumab (D) as first-line therapy in patients (pts) with unresectable hepatocellular carcinoma (uHCC): HIMALAYA. J Clin Oncol 2022;40:abstr 379.
- Finn RS, Qin S, Ikeda M, et al. Atezolizumab plus Bevacizumab in Unresectable Hepatocellular Carcinoma. N Engl J Med 2020;382:1894-905. [Crossref] [PubMed]
- Kudo M, Finn RS, Qin S, et al. Lenvatinib versus sorafenib in first-line treatment of patients with unresectable hepatocellular carcinoma: a randomised phase 3 non-inferiority trial. Lancet 2018;391:1163-73. [Crossref] [PubMed]
- Zhang Z, Liu X, Chen D, et al. Radiotherapy combined with immunotherapy: the dawn of cancer treatment. Signal Transduct Target Ther 2022;7:258. [Crossref] [PubMed]
- Herrera FG, Romero P, Coukos G. Lighting up the tumor fire with low-dose irradiation. Trends Immunol 2022;43:173-9. [Crossref] [PubMed]
- Wang Y. Advances in Hypofractionated Irradiation-Induced Immunosuppression of Tumor Microenvironment. Front Immunol 2020;11:612072. [Crossref] [PubMed]
- Demaria S, Guha C, Schoenfeld J, et al. Radiation dose and fraction in immunotherapy: one-size regimen does not fit all settings, so how does one choose? J Immunother Cancer 2021;9:e002038. [Crossref] [PubMed]
- Formenti SC, Demaria S. Combining radiotherapy and cancer immunotherapy: a paradigm shift. J Natl Cancer Inst 2013;105:256-65. [Crossref] [PubMed]
- Chiang CL, Chiu KWH, Chan KSK, et al. Sequential transarterial chemoembolisation and stereotactic body radiotherapy followed by immunotherapy as conversion therapy for patients with locally advanced, unresectable hepatocellular carcinoma (START-FIT): a single-arm, phase 2 trial. Lancet Gastroenterol Hepatol 2023;8:169-78. [Crossref] [PubMed]
- Chiang CL, Chiu WHK, Lau WHV, et al. Sequential trans-arterial chemoembolization and stereotactic body radiotherapy followed by immunotherapy (START-FIT) for locally advanced hepatocellular carcinoma: A single-arm, phase II trial. J Clin Oncol 2022;8:169-78. [Crossref] [PubMed]
- Chiang CL, Chan ACY, Chiu KWH, et al. Combined Stereotactic Body Radiotherapy and Checkpoint Inhibition in Unresectable Hepatocellular Carcinoma: A Potential Synergistic Treatment Strategy. Front Oncol 2019;9:1157. [Crossref] [PubMed]
- Juloori A, Katipally RR, Lemons JM, et al. Phase 1 Randomized Trial of Stereotactic Body Radiation Therapy Followed by Nivolumab plus Ipilimumab or Nivolumab Alone in Advanced/Unresectable Hepatocellular Carcinoma. Int J Radiat Oncol Biol Phys 2023;115:202-13. [Crossref] [PubMed]
- Li JX, Su TS, Gong WF, et al. Combining stereotactic body radiotherapy with camrelizumab for unresectable hepatocellular carcinoma: a single-arm trial. Hepatol Int 2022;16:1179-87. [Crossref] [PubMed]
- Li JX, Su TS, Lin XF, et al. Combining radiation therapy with anti-PD-1 for patients with advanced hepatocellular carcinoma: An open-label, single-center, single-arm clinical study. J Clin Oncol 2021;39:abstr e16117.
- Massachusetts General H, Genentech I. Atezolizumab + Bevacizumab + SBRT in Unresectable HCC. 2024. NCT05096715.
- Abramson Cancer Center at Penn M. SBRT With Atezo/Bev for HCC. 2024. NCT05488522.
- SBRT and Durvalumab for Inoperable/Unresectable Hepatocellular Carcinoma. NCT04913480.
- University Health Network T. Study of Pembrolizumab and Radiotherapy in Liver Cancer. 2022. NCT03316872.
- Chen Y, Yang P, Du S, et al. A phase II study of stereotactic body radiotherapy (SBRT) combined with sintilimab in patients with recurrent or oligometastatic hepatocellular carcinoma (HCC). J Clin Oncol 2022;40:abstr 4071.
- Ashwin S, Merck S, Dohme LLC, Hoosier Cancer Research N. Pembrolizumab Plus Y90 Radioembolization in HCC Subjects. 2021. NCT03099564.
- Brown TJ, Minn AJ, Carpenter EL, et al. A phase I clinical trial of stereotactic body radiotherapy with atezolizumab and bevacizumab in advanced hepatocellular carcinoma. J Clin Oncol 2023;41:abstr TPS626.
- The University of Hong K. SBRT and Durvalumab for Inoperable/Unresectable Hepatocellular Carcinoma. 2023. NCT04913480.