Efficacy of scalp-sparing volumetric-modulated arc therapy approach in reducing scalp radiation dose for patients with glioblastoma: a cross-sectional study
Highlight box
Key findings
• Radiation scalp-sparing volumetric-modulated arc therapy (SSV) for glioblastomas (GBMs) can minimize radiation doses to the scalp without compromising target coverage or exceeding critical normal structure doses.
What is known and what is new?
• Radiation therapy (RT) causes significant scalp toxicity that can impact patients’ quality of life.
• We demonstrate the feasibility of a scalp-sparing VMAT approach to minimizing undesirable radiation doses to the scalp.
What is the implication, and what should change now?
• Scalp-sparing VMAT can be safely and effectively used in the treatment of GBM to minimize RT-induced scalp toxicity.
Introduction
Background
Glioblastomas (GBMs) account for almost half of all primary brain tumor cases diagnosed each year in the United States (US). More than 14,490 people will receive this diagnosis in 2023 (1,2). GBMs are usually rapidly fatal, with a historical 5-year overall survival rate of only 9% (3). Yearly, they claim over 10,000 lives in the US. The aggressive nature of these tumor is matched by their intense treatment course. The standard of care (SoC) treatment for GBMs consists of surgical resection, and post-operative concurrent chemoradiotherapy, followed by tumor treating fields (TTFields) administered alongside maintenance chemotherapy (4).
Rationale
TTFields are the latest addition to the GBM treatment paradigm and have significantly prolonged patients’ lives (5). They deliver intermediate frequency (200 kHz) alternating electric fields transcranially, using cap-like transducer arrays affixed to the scalp. They wreak havoc on the cellular DNA repair and division machinery, stamping out cancer cell growth in the process (6,7).
TTFields only work while the transducer arrays are actively delivering the electric fields. They must hence be worn for more than 18 hours per day for optimal benefit (8). Unsurprisingly, this is a cumbersome undertaking made worse by the fact that these arrays can irritate the scalp that may have already been compromised by radiation. Erythema, dermatitis, ulceration, and open wounds have all been reported due to their use in up to 35% of patients (9). These dermatologic side effects can ultimately affect patient com/pliance with the requirement for more than 18 hours of wear time (10).
Radiation itself is a scalp irritant as well. It causes local endarteritis, fibrosis, myofibroblast injury, and epithelial atrophy, which eventually translate into a loss of scalp thickness, elasticity, and vascularization (11-14). These changes increase the risk of post-surgical wound dehiscence, wound infection, flap loss, and bone plate infection (15-18). Reducing such risks could become even more important as TTFields are incorporated into the concurrent chemoradiation phase of the treatment. EF-32 clinical trial is an ongoing trial testing just that (19). By reducing radiation injury to the skin, we may be able to avoid exacerbating this injury with the subsequent use of TTFields.
Both clinicians and patients, therefore, have a vested interest in preventing or mitigating dermatologic toxicity.
Dermatologic toxicity
Dermatologic toxicity is usually managed either prophylactically or with treatment interventions. Prophylactic measures include educating patients on proper scalp care, hygienic ways to apply and remove the transducer arrays, and to periodically shift the arrays to different parts of the scalp. Treatment interventions can consist of topical corticosteroids if patients develop scalp dermatitis or topical antibiotics if they show signs of scalp infection. For more severe skin side effects, small treatment interruptions can also be considered to give the skin a chance to heal (9,20).
A novel way to mitigate such toxicities may be to reduce scalp radiation by contouring the scalp as an organ at risk (OAR) and using scalp dose constraints during treatment planning. This technique is termed scalp-sparing volumetric-modulated arc therapy (SSV) (21).
Such scalp avoidance, however, should not trade decreased scalp toxicity for optimal clinical outcomes or expose critical brain structures to unjustifiable risk. These outcomes depend on many factors, including the delivery of a sufficiently lethal radiation dose to the tumor as evidenced by the planning treatment volume (PTV) dose distribution (22). Harm to critical brain structures, including the brainstem, optic nerves, and optic chiasm, is minimized by respecting their radiation tolerances (23).
Knowledge gap and objective
A single arm pilot study (clinicaltrials.gov Identifier: NCT03477110) investigated the safety of delivering SSV in conjunction with TTFields and reported favorable findings (14). To our knowledge, a head-to-head dosimetric comparison between SSV and traditional non-SSV approaches has not been reported in the literature. Herein we compare dosimetric parameters between the two approaches, including scalp, PTV, and Organ at Risk (OAR) doses, to test the efficacy of an SSV approach in reducing scalp dose without compromising PTV coverage or putting OARs at risk.
Such an approach has the potential to prevent or reduce scalp toxicities for GBM patients and increase compliance. A prospective trial evaluating the clinical benefit of this technique is ongoing (Clinicaltrials. gov, identifier: NCT03251027).
We present this article in accordance with the STROBE reporting checklist (available at https://cco.amegroups.com/article/view/10.21037/cco-23-15/rc).
Methods
This retrospective cross-sectional study was conducted in accordance with the Declaration of Helsinki (as revised in 2013). The study was approved by Thomas Jefferson University Hospital’s (TJUH) institutional review board (No. FWA 00002109) and individual consent for this retrospective analysis was waived.
Patient selection
We retrospectively queried patient databases from TJUH and identified 19 patients with newly diagnosed GBMs, World Health Organization (WHO) Grade IV, who were treated at our institution between 2015 and 2018 with standard of care (SoC) treatment (24), including concurrent chemotherapy [temozolomide (TMZ): 75 mg/m2] and conventional VMAT without scalp-sparing optimization to a total dose of 60 Gy. This included patients who received RT in a single course, 60 Gy in 30 fractions, as well as a split course, consisting of 46 Gy in 23 fractions to an initial volume followed by 14 Gy in 7 fractions to a cone-down, smaller volume. We excluded patients who received hypofractionated radiation treatment.
Scalp, OAR, and target volume contours
We generated scalp contours on the CT simulation scans used for the patients’ original non-SSV treatment planning. The scalp was defined as a 5-mm thick, rind-like structure directly beneath the cranial skin surface. This scalp contour was extended caudally to the level of the foramen magnum (14). Figure 1 shows the scalp contour for a representative patient in axial, coronal, and sagittal planes as well as a 3D rendering.
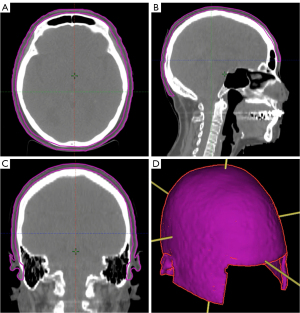
Radiation Therapy Oncology Group (RTOG/NRG) consensus guidelines were used to delineate OARs (25). European Organization for Research and Treatment (EORTC) and RTOG guidelines were used to generate the CTV, GTV, and PTV contours per the treating physicians (26). We used the original non-SSV contours without modifications for SSV treatment planning.
The EORTC guidelines define the gross tumor volume (GTV) as the enhancing lesion on T1 post-contrast MR imaging as well as the post-op surgical cavity. The GTV is expanded by 2 cm to generate the CTV, which is then edited to reflect the natural barriers to tumor growth. This includes the bones, tentorium, and falx. Finally, the PTV is generated by geometrically expanding the CTV by 3 mm. Ninety-five percent of the PTV receives 100% of the prescribed radiation dose (60 Gy in 30 fractions).
The RTOG/NRG guidelines, on the other hand, use a staggered approach to contouring the target volumes. They define GTV1 as the T1 post-contrast enhancing lesion and the post-op surgical cavity. In addition, they require a GTV2, which is defined as GTV1, and all areas of FLAIR abnormalities on T2 MR imaging. Both the GTVs are radially expanded to generate CTV1 and CTV2 respectively. These CTVs are then edited to respect the natural barriers to tumor growth mentioned before. PTV1 and PTV2 are generated by uniformly expanding CTV1 and CTV2 by 3 mm. PTV1 is prescribed 46 Gy in 23 fractions. PTV2 is prescribed an additional 14 Gy in 7 fractions for a total dose of 60 Gy in 30 fractions.
A prospective randomized trial revealed no difference in outcomes in patients treated with either the RTOG volumes or the smaller EORTC volumes. Hence, treating physicians were allowed to use the guideline of their choice.
Radiation simulation, treatment planning, and dose constraints
Patients were simulated in a supine position on an intensity-modulated radiation therapy (IMRT) board. Aquaplast masks were used to immobilize patients’ head and neck areas in a neutral neck position.
Volumetric modulated arc therapy (VMAT) technique was utilized for treatment planning. All plans were generated in Eclipse treatment planning system (Varian, Palo Alto, CA) using 6 MV photons. Initial treatment plans for all patients from 2015 through 2018 were planned in Eclipse version 11.0. Replanning with scalp sparing constraints for this study were done in Eclipse version 15.6.
Each plan utilized 2 clockwise and 2 counterclockwise (4 total) non-coplanar arcs. The arcs were primarily set ipsilateral to the PTV. A 0° couch angle was used for one set of arcs and a 90° couch angle was used for the other. If feasible, radiation beam entrance through the eyes was minimized by tilling the gantry angle away from the eyes. The same arc positions and range were used for the initial plans and replans.
Plans and replans were optimized to ensure that 95% of the PTV was covered by 100% of the dose. If the treatment consisted of initial and sequential boosts, each plan was normalized separately to cover 95% of the PTV with 100% of the dose, and then combined into a composite plan.
TJUH institutional dose constraints, derived from various protocols (27), were used for the initial plans and replans: Optic nerves Dmax <58 Gy, Optic Chiasm Dmax <56 Gy, Brainstem Dmax <60 Gy, bilateral retina Dmax <50 Gy, bilateral lens Dmax <10 Gy Cochlea Dmax <40 Gy, Scalp Dmean <20 Gy, Scalp D20cc <50 Gy, Scalp V30cc <40 Gy (25,28,29). Adequate PTV coverage was prioritized over scalp dose constraints.
Dosimetric evaluation
We compared various dosimetric parameters between non-SSV and SSV plans using dose-volume histograms (DVHs). To comprehensively quantify radiation doses to the scalp, we measured scalp Dmin, Dmax, Dmean, D20cc, D30cc, V30Gy, V40Gy, and V50Gy for all plans. PTV Dmin, Dmax, and Dmean were measured to evaluate target volume coverage. Data on Dmax to select critical structures including optic nerves and brainstem were also collected.
Statistical analysis
Two-sided student’s t-test was used to compare the difference in doses to the parameters listed above between non-SSV and SSV plans. Statistical significance was defined at the 5% level (P<0.05). The analysis was performed using Prism version 10.0.40 for Mac (GraphPad Software Inc., La Jolla, California, USA).
Results
Nineteen patients treated at TJUH between 2015 and 2018 with SoC including non-SSV radiation plans were selected for our study. Table 1 summarizes baseline patient characteristics. Eighty-four percent were male while 16% were female. Median age was 65 years (range, 33–78). Thirty-seven percent underwent a gross total resection while 63% received a subtotal resection. Fifty-four percent were O6-Methylguanine-DNA Methyltransferase (MGMT) methylated and 46% were unmethylated. Isocitrate dehydrogenase (IDH) was mutated in 21% of the patients while 79% were IDH wild type. Forty-seven percent of the patients received 60 Gy in 30 fractions in a single course, while 53% received split course radiation, with 46 Gy in 23 fractions followed by 14 Gy in 7 fractions delivered to initial and cone-down volumes respectively.
Table 1
Baseline characteristics | Value |
---|---|
Age | |
Median [range] | 65 years [33–78] |
Gender | |
Male | 84% |
Female | 16% |
Resection | |
GTR | 37% |
STR | 63% |
MGMT status | |
Methylated | 54% |
Unmethylated | 46% |
IDH status | |
Mutated | 21% |
Wild type | 79% |
Scalp volume, cm3 | |
Median [range] | 423 [379–468] |
Treatment protocol | |
EORTC | 47% |
RTOG/NRG | 53% |
MGMT, O6-Methylguanine-DNA Methyltransferase; IDH, isocitrate dehydrogenase; EORTC, European Organization for Research and Treatment; RTOG/NRG, Radiation Therapy Oncology Group.
After adding the scalp as an OAR, new SSV plants were generated for all patients. Table 2 compares median doses and doses delivered to different volumes for the scalp, PTV, and select OARs between non-SSV and SSV plans. SSV significantly reduced the minimum, maximum, and mean scalp doses, and doses received by 20 and 30 cc of the scalp.
Table 2
Structures | Dose/Volume | Non-SSV median1 | SSV median2 | Median % change3 | P value4 |
---|---|---|---|---|---|
Scalp | Dmin (Gy) | 0.3 | 0.1 | −43.5 | <0.01 |
Dmax (Gy) | 61.1 | 57.4 | −2.8 | 0.04 | |
Dmean (Gy) | 13.3 | 11.1 | −15.7 | <0.01 | |
D20cc (Gy) | 38.7 | 31.5 | −19.5 | <0.01 | |
D30cc (Gy) | 34.5 | 27.4 | −19.0 | <0.01 | |
V30Gy (cm3) | 40.2 | 25.8 | −42.3 | <0.01 | |
V40Gy (cm3) | 18.6 | 6.2 | −72.0 | <0.01 | |
V50Gy (cm3) | 7.0 | 0.2 | −92.4 | <0.01 | |
PTV | Dmin (Gy) | 47.9 | 51.3 | 2.0 | 0.50 |
Dmax (Gy) | 64.9 | 65.8 | 1.4 | 0.57 | |
Dmean (Gy) | 61.7 | 61.9 | 0.2 | 0.64 | |
OAR | |||||
Right optic nerve | Dmax (Gy) | 45.9 | 45.2 | −0.8 | 0.68 |
Left optic nerve | Dmax (Gy) | 39.2 | 35.8 | 0 | 0.61 |
Optic chiasm | Dmax (Gy) | 51.6 | 52.1 | 0.8 | 0.27 |
Brainstem | Dmax (Gy) | 54.5 | 54.8 | 0.8 | 0.68 |
1, non-SSV plan; 2, SSV plan; 3, median % change in dose between non-SSV and SSV plans; 4, P values with statistical significance defined at the 5% level (P<0.05). PTV, planning target volume; OAR, organ at risk; SSV, scalp-sparing volumetric-modulated arc therapy.
The median scalp volume was 423 cm3 (range, 363 to 468 cm3). The median reduction in scalp Dmin was 43.5% (range, −100% to 0%) from 0.3 to 0.1 Gy. The median reduction in scalp Dmax was 2.8% (range, +13.4% to −24.9%) from 61.1 to 57.4 Gy. The median scalp Dmean reduction was 15.7% (range, +2.1% to −39.9%) from 13.3 to 11.1 Gy. Median reductions in scalp D20cc and D30cc were 19.5% (range, −2.7% to −54.5%), from 38.7 to 31.5 Gy, and 19.0% (range, −5.3% to −39.5%), from 34.5 to 27.4 Gy, respectively. Additionally, the median volumes of the scalp receiving 30 Gy, 40 Gy, and 50 Gy were reduced by 42.3% (range, −70.6% to −12.5%), 72% (range, −100% to −2.3%), and 92.4% (range, −100% to +5.4%) respectively.
Figure 2 is a visual depiction of scalp dose reduction in a representative patient achieved via an SSV plan. Figure 3 shows non-SSV scalp DVHs (thin solid red lines) overlaid with SSV scalp DVHs (thin solid green lines) for individual patients as well as the mean non-SSV (thicker dashed red line) and mean SSV (thicker dashed green line) scalp DVHs for the entire cohort.
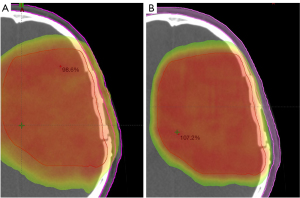
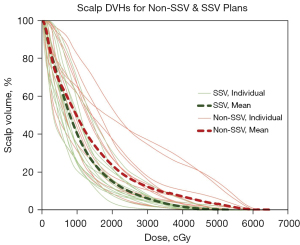
The 9 patients treated in accordance with the EORTC protocol were analyzed separately. The median scalp volume was 410 cm3 (range, 379 to 468 cm3). The median reduction in scalp Dmin was 90.6% (range, −100% to 0%) from 0.4 to 0 Gy. The median scalp Dmax increased by 2.2% (range, −24.9% to +13.4%) from 62.6 to 63.1 Gy. The median scalp Dmean reduction was 23.5% (range, −39.9% to −1.4%) from 11.4 to 9.9 Gy. Median reductions in scalp D20cc and D30cc were 22.6% (range, −36.9% to −2.7%; P=0.92), from 37.4 to 33.5 Gy, and 25.5% (range, −39.5% to 5.3%; P=0.10), from 34.5 to 26.6 Gy, respectively. Additionally, the median volumes of the scalp receiving 30 Gy, 40 Gy, and 50 Gy were reduced by 58.4% (range, −70.6% to −15.1%), 76.4% (range, −100% to −2.3%), and 66.0% (range, −100% to +5.4%) respectively.
The 10 patients treated in accordance with the RTOG/NRG guidelines were similarly analyzed separately and then compared to the EORTC patients (P values noted in parenthesis). The median scalp volume was 417 cm3 (range, 363 to 449 cm3; P=0.46). The median reduction in scalp Dmin was 41.0% (range, −83.6% to 0%; P=0.12) from 0.2 to 0.1 Gy. The median scalp Dmax decreased by 8.0% (range, −23.1% to +6.7%; P=0.23) from 59.9 to 55.5 Gy. The median scalp Dmean reduction was 13.3% (range, −25.4% to +2.1%; P=0.16) from 13.9 to 12.0 Gy. Median reductions in scalp D20cc and D30cc were 18.6% (range, −54.5% to −13.2%; P=0.92), from 39.5 to 31.5 Gy, and 16.7% (range, −30.1% to −12.9%; P=0.10), from 35.3 to 28.2 Gy, respectively. Additionally, the median volumes of the scalp receiving 30 Gy, 40 Gy, and 50 Gy were reduced by 40.9% (range, −55.9% to −12.5%; P=0.26), 71.8% (range, −86.4% to −34.1%, P=0.68), and 96.1% (range, −100% to −14.9%, P=0.14) respectively. There was no statistically significant difference in these parameters between the EORTC and RTOG/NRG patients.
There is no significant dosimetric difference in the PTV between the non-SSV and SSV plans. The median Dmin was 47.9 Gy and 51.3 Gy (+2.0% change, P=0.50), the median Dmax was 64.9 Gy and 65.8 Gy (+1.4% change, P=0.57), and the median Dmean was 61.7 Gy and 61.9 Gy (0.2% difference, P=0.64) for the non-SSV and SSV plans respectively. Similarly, Dmax to the brainstem, optic nerves, and optic chiasm did not change significantly between non-SSV and SSV plans (as shown in Table 2).
Figure 4 depicts non-SSV PTV DVHs (thin solid red lines) overlaid with SSV PTV DVHs (thin solid green lines) for individual patients as well as the mean non-SSV (thicker dashed red line) and mean SSV (thicker solid green line) PTV DVHs for the entire cohort.
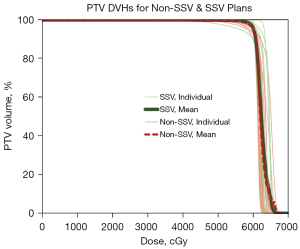
Discussion
The toils of undergoing GBM treatment cannot be overstated. In addition to the logistical challenges that upend patients’ lives, they must contend with treatment toxicity that takes a toll on their quality of life (QoL). This includes fatigue, dizziness, blood cytopenias, alopecia, and non-healing scalp wounds (30), among others (4), Scalp toxicity has become especially concerning due to the incorporation of TTFields in the GBM treatment package. TTFields use transducer arrays, which are secured to the same areas of the scalp with adhesives for long periods of time, leading to continuous thermal, mechanical, chemical, and moisture-related irritation to the scalp (9). Irradiated skin is especially vulnerable to these insults, as radiation compromises the highly ordered sequence of cellular events required for wound healing (31). Thus, reducing radiation doses to the scalp to minimize toxicity is a veritable clinical goal. Our study demonstrates that this goal is achievable through an SSV treatment approach applied to both the EORTC and RTOG guidelines for GBM treatment.
Of course, minimizing scalp toxicity should not take precedence over objectives critical to treatment success. Ensuring adequate target or PTV coverage and not dosing highly critical brain structures beyond their tolerances are goals of the highest priorities. Unsurprisingly, PTV underdosing is predictive of treatment failures (22). Ideal PTV coverage requires V100 ≥95% PTV, but this is not always possible, especially if the tumor is in the vicinity of critical structures (21). Therefore, V95% ≥95% PTV is an acceptable and likely more practical alternative. Our study shows that an SSV approach does not lead to inadequate PTV doses, and thus, does not cause an unjustifiable increase in the risk of treatment failure.
PTV volumes, especially in the case of peripheral tumors, can also encroach on the scalp (32). This can necessitate violating the scalp dose constraints, and therefore, strict sparing may not always be practical. Even in such cases, minimizing scalp dose to reasonably achievable levels may avoid the degree of scalp toxicity that may otherwise result from total scalp non-avoidance.
Another treatment planning consideration is the abundance of critical structures (OARs) in the vicinity of GBM tumors. Firstly, GBM severely curtails patients’ lifespan, making optimizing the QoL a prime consideration for many. Any damage to critical brain structures and the consequent sequalae naturally run counter to this consideration (33). Brainstem injury, for example, can lead to gait instability, debilitating muscle weakness, and facial paralysis (23,34). Injury to the optic nerves may result in vision loss (35). Secondly, GBM has an undesirable knack for recurrence, which can require additional radiation for management (36). The feasibility of any retreatment hinges on the doses delivered to the OARs during prior courses of radiotherapy. All in all, radiation oncologists are doubly incentivized to respect dose constraints to critical structures. Our analysis shows that sparing the scalp did not violate these constraints, establishing the safety of the SSV approach.
Our study has limitations inherent to all retrospective analyses, such as selection biases. We included a small cohort of 19 patients. It is possible that this represents a patient population with tumors unusually amenable to an SSV approach. There could be GBM tumors unlike the ones in our study where the scalp cannot be meaningfully spared unless PTV dose or OAR safety is compromised. Additionally, we did not treat patients with the SSV plans. Therefore, we cannot conclude whether this approach would translate into an effective decrease in scalp toxicity. Preliminary results from an ongoing prospective phase II trial evaluating this benefit (clinicaltrials.gov Identifier: NCT03251027) were encouraging. Patients treated with an SSV approach had a median 13% decrease in scalp thickness 9 months post-radiation as compared to a 30% decrease reported for the non-SSV cohort (2). A decrease in scalp thickness correlates with scalp toxicity.
SSV is a feasible technique to significantly reduce scalp radiation doses without sacrificing tumor coverage or overdosing critical brain structures. This could translate into reduced short and long-term toxicity to the scalp. This is especially important as TTFields, now a staple in the GBM treatment paradigm, come with their own scalp side effects that can compound radiation-induced dermatologic injuries. By circumventing such injuries, we can greatly improve patients’ QoL. This may lead to reduced acute and late radiation toxicity to the scalp. A prospective trial evaluating the clinical benefit of this technique is ongoing (clinicaltrials.gov Identifier: NCT03251027).
Conclusions
Scalp-sparing VMAT is a feasible technique that can significantly reduce scalp radiation dose without compromising target coverage or critical normal structure doses. This may lead to reduced acute and late radiation toxicity to the scalp. A prospective trial evaluating the clinical benefit of this technique is ongoing (clinicaltrials.gov Identifier: NCT03251027).
Acknowledgments
Funding: None.
Footnote
Provenance and Peer Review: This article was commissioned by the editorial office, Chinese Clinical Oncology for the series “Recent Advances in Neuro-Oncology”. The article has undergone external peer review.
Reporting Checklist: The authors have completed the STROBE reporting checklist. Available at https://cco.amegroups.com/article/view/10.21037/cco-23-15/rc
Data Sharing Statement: Available at https://cco.amegroups.com/article/view/10.21037/cco-23-15/dss
Peer Review File: Available at https://cco.amegroups.com/article/view/10.21037/cco-23-15/prf
Conflicts of Interest: All authors have completed the ICMJE uniform disclosure form (available at https://cco.amegroups.com/article/view/10.21037/cco-23-15/coif). The series “Recent Advances in Neuro-Oncology” was commissioned by the editorial office without any funding or sponsorship. WS served as the unpaid Guest Editor of the series and serves as an unpaid Associate Editor-in-Chief of Chinese Clinical Oncology from October 2019 to September 2023. WS also received consulting fees from Brainlab, Novocure, Zai Lab, and Varian, and participated in the advisory board for Novocure. HL received funding from Brainlab and participated in BrainLAB Novalis circle educational webinar. The other authors have no other conflicts of interest to declare.
Ethical Statement: The authors are accountable for all aspects of the work in ensuring that questions related to the accuracy or integrity of any part of the work are appropriately investigated and resolved. The study was conducted in accordance with the Declaration of Helsinki (as revised in 2013). The study was approved by Thomas Jefferson University Hospital’s (TJUH) institutional review board (No. FWA 00002109) and individual consent for this retrospective analysis was waived.
Open Access Statement: This is an Open Access article distributed in accordance with the Creative Commons Attribution-NonCommercial-NoDerivs 4.0 International License (CC BY-NC-ND 4.0), which permits the non-commercial replication and distribution of the article with the strict proviso that no changes or edits are made and the original work is properly cited (including links to both the formal publication through the relevant DOI and the license). See: https://creativecommons.org/licenses/by-nc-nd/4.0/.
References
- Glioblastoma Multiforme – Symptoms, Diagnosis and Treatment Options n.d. Available online: https://www.aans.org/en/Patients/Neurosurgical-Conditions-and-Treatments/Glioblastoma-Multiforme (accessed December 15, 2022).
- Ali A, Niazi MZK, Andrews DW, et al. Scalp-Sparing Volume Modulated Radiation Therapy (VMAT) for Newly Diagnosed Gliomas: A Phase 2 Trial. International Journal of Radiation Oncology*Biology*Physics 2021;111:e585.
- Survival Rates for Selected Adult Brain and Spinal Cord Tumors n.d. Available online: https://www.cancer.org/cancer/brain-spinal-cord-tumors-adults/detection-diagnosis-staging/survival-rates.html (accessed December 15, 2022).
- De Vleeschouwer SFernandes C, Costa A, et al. Current Standards of Care in Glioblastoma Therapy. 2017.
- Guzauskas GF, Salzberg M, Wang BC. Estimated lifetime survival benefit of tumor treating fields and temozolomide for newly diagnosed glioblastoma patients. CNS Oncol 2018;7:CNS23. [Crossref] [PubMed]
- Miller R, Niazi M, Russial O, et al. Tumor treating fields with radiation for glioblastoma: a narrative review. Chin Clin Oncol 2022;11:40. [Crossref] [PubMed]
- Rominiyi O, Vanderlinden A, Clenton SJ, et al. Tumour treating fields therapy for glioblastoma: current advances and future directions. Br J Cancer 2021;124:697-709. [Crossref] [PubMed]
- Stupp R, Taillibert S, Kanner A, et al. Effect of Tumor-Treating Fields Plus Maintenance Temozolomide vs Maintenance Temozolomide Alone on Survival in Patients With Glioblastoma: A Randomized Clinical Trial. JAMA 2017;318:2306-16. [Crossref] [PubMed]
- Lacouture ME, Anadkat MJ, Ballo MT, et al. Prevention and Management of Dermatologic Adverse Events Associated With Tumor Treating Fields in Patients With Glioblastoma. Front Oncol 2020;10:1045. [Crossref] [PubMed]
- Mehta M, Wen P, Nishikawa R, et al. Critical review of the addition of tumor treating fields (TTFields) to the existing standard of care for newly diagnosed glioblastoma patients. Crit Rev Oncol Hematol 2017;111:60-5. [Crossref] [PubMed]
- Weintraub NL, Jones WK, Manka D. Understanding radiation-induced vascular disease. J Am Coll Cardiol 2010;55:1237-9. [Crossref] [PubMed]
- Straub JM, New J, Hamilton CD, et al. Radiation-induced fibrosis: mechanisms and implications for therapy. J Cancer Res Clin Oncol 2015;141:1985-94. [Crossref] [PubMed]
- Toshkov IA, Gleiberman AS, Mett VL, et al. Mitigation of Radiation-Induced Epithelial Damage by the TLR5 Agonist Entolimod in a Mouse Model of Fractionated Head and Neck Irradiation. Radiat Res 2017;187:570-80. [Crossref] [PubMed]
- Miller R, Song A, Ali A, et al. Scalp-Sparing Radiation With Concurrent Temozolomide and Tumor Treating Fields (SPARE) for Patients With Newly Diagnosed Glioblastoma. Front Oncol 2022;12:896246. [Crossref] [PubMed]
- Larson DA. Surgical management of radiated scalp in patients with recurrent glioma. Neurosurgery 1994;34:1105. [Crossref] [PubMed]
- Kasper EM, Ridgway EB, Rabie A, et al. Staged scalp soft tissue expansion before delayed allograft cranioplasty: a technical report. Neurosurgery 2012;71:15-20; discussion 21. [Crossref] [PubMed]
- Barami K, Fernandes R. Incidence, risk factors and management of delayed wound dehiscence after craniotomy for tumor resection. J Clin Neurosci 2012;19:854-7. [Crossref] [PubMed]
- Golas AR, Boyko T, Schwartz TH, et al. Prophylactic plastic surgery closure of neurosurgical scalp incisions reduces the incidence of wound complications in previously-operated patients treated with bevacizumab (Avastin®) and radiation. J Neurooncol 2014;119:327-31. [Crossref] [PubMed]
- Pivotal, Randomized, Open-label Study of Optune® (Tumor Treating Fields) Concomitant With RT & TMZ for the Treatment of Newly Diagnosed GBM - Full Text View - ClinicalTrials.gov n.d. Available online: https://clinicaltrials.gov/ct2/show/NCT04471844 (accessed December 15, 2022).
- Lacouture ME, Davis ME, Elzinga G, et al. Characterization and management of dermatologic adverse events with the NovoTTF-100A System, a novel anti-mitotic electric field device for the treatment of recurrent glioblastoma. Semin Oncol 2014;41:S1-14. [Crossref] [PubMed]
- Hanif F, Muzaffar K, Perveen K, et al. Glioblastoma Multiforme: A Review of its Epidemiology and Pathogenesis through Clinical Presentation and Treatment. Asian Pac J Cancer Prev 2017;18:3-9. [Crossref] [PubMed]
- Sherriff J, Tamangani J, Senthil L, et al. Patterns of relapse in glioblastoma multiforme following concomitant chemoradiotherapy with temozolomide. Br J Radiol 2013;86:20120414. [Crossref] [PubMed]
- Mayo C, Yorke E, Merchant TE. Radiation associated brainstem injury. Int J Radiat Oncol Biol Phys 2010;76:S36-41. [Crossref] [PubMed]
- Stupp R, Mason WP, van den Bent MJ, et al. Radiotherapy plus concomitant and adjuvant temozolomide for glioblastoma. N Engl J Med 2005;352:987-96. [Crossref] [PubMed]
- NRG > About Us > Center for Innovation in Radiation Oncology > Brain Tumors n.d. Available online: https://www.nrgoncology.org/ciro-brain (accessed November 28, 2022).
- Cabrera AR, Kirkpatrick JP, Fiveash JB, et al. Radiation therapy for glioblastoma: Executive summary of an American Society for Radiation Oncology Evidence-Based Clinical Practice Guideline. Pract Radiat Oncol 2016;6:217-25. [Crossref] [PubMed]
- Gilbert MR, Dignam J, Won M, et al. RTOG 0825: Phase III double-blind placebo-controlled trial evaluating bevacizumab (Bev) in patients (Pts) with newly diagnosed glioblastoma (GBM). J Clin Oncol 2013;18:1.
- Li T, Shukla G, Peng C, et al. Dosimetric Impact of a Tumor Treating Fields Device for Glioblastoma Patients Undergoing Simultaneous Radiation Therapy. Front Oncol 2018;8:51. [Crossref] [PubMed]
- Song A, Bar-Ad V, Martinez N, et al. Initial experience with scalp sparing radiation with concurrent temozolomide and tumor treatment fields (SPARE) for patients with newly diagnosed glioblastoma. J Neurooncol 2020;147:653-61. [Crossref] [PubMed]
- Kim J, Ahn KC, Chang H, et al. Surgical Treatment of Radiation-Induced Late-Onset Scalp Wound in Patients Who Underwent Brain Tumor Surgery: Lessons from a Case Series. Biomed Res Int 2022;2022:3541254. [Crossref] [PubMed]
- Haubner F, Ohmann E, Pohl F, et al. Wound healing after radiation therapy: review of the literature. Radiat Oncol 2012;7:162. [Crossref] [PubMed]
- Guberina N, Pöttgen C, Kebir S, et al. Combined radiotherapy and concurrent tumor treating fields (TTFields) for glioblastoma: Dosimetric consequences on non-coplanar IMRT as initial results from a phase I trial. Radiat Oncol 2020;15:83. [Crossref] [PubMed]
- Briere TM, McAleer MF, Levy LB, et al. Sparing of normal tissues with volumetric arc radiation therapy for glioblastoma: single institution clinical experience. Radiat Oncol 2017;12:79. [Crossref] [PubMed]
- Yao CY, Zhou GR, Wang LJ, et al. A retrospective dosimetry study of intensity-modulated radiotherapy for nasopharyngeal carcinoma: radiation-induced brainstem injury and dose-volume analysis. Radiat Oncol 2018;13:194. [Crossref] [PubMed]
- Ferguson I, Huecker J, Huang J, et al. Risk factors for radiation-induced optic neuropathy: a case-control study. Clin Exp Ophthalmol 2017;45:592-7. [Crossref] [PubMed]
- Roy S, Lahiri D, Maji T, et al. Recurrent Glioblastoma: Where we stand. South Asian J Cancer 2015;4:163-73. [Crossref] [PubMed]