Expression of YAP suppresses cell proliferation and elevates the sensitivity of chemotherapy in retinoblastoma cells through lipid-peroxidation induced ferroptosis
Highlight box
Key findings
• Our study creatively highlights that over-expression of yes-associated protein (YAP) can mediate lipid peroxidation induced ferroptosis and further improve the sensitivity of retinoblastoma (RB) to cisplatin/etoposide.
What is known and what is new?
• RB is a tumor known for low expression of YAP, which correlated with the phenomenon of chemo-resistance.
• We demonstrate the expression of YAP suppresses RB cells proliferation through lipid peroxidation induced ferroptosis, and confirm that PY-60, a specific activator of YAP transcriptional activity, can be used in combination with cisplatin/etoposide to improve the therapeutic effect.
What is the implication, and what should change now?
• Our experimental results will provide a new choice for the follow-up treatment of RB and provide preliminary basic support for the clinical application of ferroptosis agonists in RB.
Introduction
Retinoblastoma (RB) is the most common primary intraocular malignant tumor in children, accounting for 17% of the total number of infant tumors. Around the world, more than 8,000 children are diagnosed with RB every year, with an incidence rate of 1:15,000–1:20,000. The survival rate of RB patients is more than 95% in high-income countries, but less than 30% globally (1). The occurrence and progression of RB are closely related to the interaction of multiple genes (2). The researchers have been proved that the expression of genes such as UHRF1 can promote RB cells proliferation and invasion (3). RB mostly occurs in developing countries, among which the most common initial signs and referral indications are a white pupil, and there may also be red and swollen eyes, exophthalmos, and other symptoms (1,4). If the patient is not treated in time, fatality may occur due to the development of metastatic RB. Therefore, the primary goal of treatment is to save lives, followed by retaining the eyeballs, preserving vision, and improving patient quality of life.
At present, the treatment methods of RB mainly include intravenous chemotherapy (IVC), intra-arterial chemotherapy (IAC), intravitreal chemotherapy (IvitC), eyeball enucleation, local laser, cryotherapy, and so on (5,6). Determination of the treatment mode needs to consider the grading, staging, and clinical characteristics of RB. At the current stage, chemotherapy is the first-line treatment for RB. At present, the drugs used for RB chemotherapy include carboplatin, cisplatin (7), vincristine, etoposide, cyclophosphamide, melphalan, and topotecan (8). However, long-term chemotherapy not only induces various side effects related to the dosage of chemotherapy drugs, but also causes chemotherapeutic resistance (9). The poor prognosis caused by chemotherapeutic resistance poses a huge challenge to maintaining the effect of cancer treatment (10,11). Therefore, our research attempts to ascertain the relevant mechanism of RB resistance to cisplatin, and to reverse and improve the occurrence of resistance (12,13).
Yes-associated protein (YAP), is a key effector of Hippo signal pathway, and also a connexin and transcriptional coactivator in multifunctional cells (14). It plays a significant role in signal transduction and gene transcription regulation in normal cells (15). Many research results show that YAP is involved in the occurrence and development of multiple systemic tumors (16-18), and most of them are carcinogenic genes in human body. In the past 10 years of research, many researchers have confirmed that disorder of the Hippo signaling pathway may cause the occurrence and development of many human malignant tumors (19). Analysis of The Cancer Genome Atlas (TCGA) has shown that the copy number of the YAP gene is often amplified in many types of cancer cells. Elevated expression of YAP has been found in a variety of human cancer tissues, including lung cancer (20), hepatocellular carcinoma (HCC) (21), ovarian cancer (22), cervical cancer (23), endometrial carcinoma (24), colorectal carcinoma (25), RB (26), and so on. Contrary to the abnormal amplification of YAP gene copy number, in many cancer patients, tumor suppressor kinase genes upstream of the Hippo signal pathway, such as NF2 and LATS1/2, are often deleted or mutated (27). These evidences strongly support that the Hippo/YAP signaling pathway may be an important potential target for cancer treatment. However, the role of YAP in RB still remains largely unclear.
Ferroptosis is a new iron dependent programmed cell death mode. The main characteristics of ferroptosis include the accumulation of reactive oxygen species (ROS), unrestricted lipid peroxidation and subsequent membrane damage (28). The main mechanism and signal pathway of ferroptosis are relatively complex, which are closely related to cystine/glutamate reverse transporter, glutathione peroxidase 4, ferroptosis inhibitor protein 1, and dihydrowhey dehydrogenase, among others (29). In recent years, ferroptosis has been confirmed to provide a new therapeutic direction for interfering with the progress of different types of malignant tumors (30,31). To date, several types of ferroptosis inducers and inhibitors have been found to provide advantages for improving the sensitivity of chemotherapy in pre-clinical research (32).
Based on the above, we hypothesized that the silenced expression of YAP has a profound correlation with the malignant progression of RB. In this study, we conducted relevant experimental work to verify the biological role of YAP in RB cells. We mainly selected the human RB cell lines Y79/RB3823 according to their lower expression level of YAP. Through over-expression of the YAP gene, it was confirmed that YAP inhibited the growth of RB cells, led to the increased lipid peroxidation and ferroptosis. As a result, expression of YAP elevated the sensitivity of cisplatin and etoposide in RB cells. We present this article in accordance with the MDAR reporting checklist (available at https://cco.amegroups.com/article/view/10.21037/cco-23-97/rc).
Methods
Cells and cell culture
Human RB cell line Y79 were purchased from ATCC, Human RB cell line SO-RB50 and Human RB cell line RB3823 were kindly provided by Brenda Gallie’ Lab. Human retina Müller cells were purchased from Procell company. Both the Y79, RB3823, SO-RB50 and Müller cells were cultured in Dulbecco’s Modified Eagle Medium (Gibco, Thermo Fisher, Waltham, MA, USA) supplemented with 10% FBS (Gibco) and 1% double-antibiotics (penicillin-streptomycin). All cells were incubated in a humidified atmosphere with 5% CO2 at 37 ℃.
MTS assay
Human RB cells were subjected to [1–2]×103 cells/200 µL/well, incubated in 96-well plate for 24 hours. In the last 4 hours of incubation, added 20 µL to each hole according to the product protocol for MTS assay (Promega, Madison, WI, USA). Determine the cell viability by measuring the absorption at 490 nm; The cell survival curve was drawn according to the data results.
Quantitative real-time polymerase chain reaction
According to the corresponding kit protocols, the first step is to extract the total RNA of cells using TRIzol reagents (RNAiso PLUS, Invitrogen, Carlsbad, CA, USA). The isolated RNA samples were measured by Bioanalyzer 2200 (Agilent, Santa Clara, CA, USA), and the RNA concentration, sample quality and purity were calculated before reverse transcription to obtain DNA (cDNA). According to the corresponding kit protocols, the Real-Time PCR Detection System (Lightcycle 480 II, Roche, Indianapolis, IN, USA) was used for quantitative real-time polymerase chain reaction (qRT-PCR). Application 2−ΔΔCt method was used to analyze the multiple change of reaction control groups.
Western blot
First, the cell sample was lysed using the RIPA buffer (Beyotime, Shanghai, China) adding with protease and phosphatase inhibitors (Life Technologies, Carlsbad, CA, USA). Then, according to the corresponding kit protocols, the BCA kit (Thermo Fisher, USA) is used to detect the concentration of protein samples. Protein samples were subjected to 8–10% sodium dodecyl sulfate polyacrylamide gel electrophoresis (SDS-PAGE), and then transferred to polyvinylidene fluoride (PVDF) membrane (Bio Rad, Hercules, CA, USA). After placing the sample in 5% skimmed milk for 1 hour, incubate the PVDF membrane with the primary antibody at 4 ℃ for overnight. The primary antibody is directed against YAP (1:1,000, Thermo Fisher) β-specific antibody to actin (1:1,000). The next day, PVDF membrane were washed with tri-buffered saline added with 0.1% Tween-20 (TBST), and then incubated with horseradish peroxide (HRP) anti-rabbit (1:1,000, CST) secondary antibody for 2 hours. Finally, the PVDF membrane protein bands were developed using a chemiluminescence imager (Thermo Fisher, MA, USA) and the results were recorded.
YAP overexpressed stable cell lines construction
According to the specific coding sequence information of YAP messenger RNA (mRNA) (NM_001282101.2), corresponding to the cDNA of YAP synthesis, was completely embedded into the pLenti-CMV-Puro plasmid; At the same time, the lentivirus expression plasmid was used to transfect and package 293T cells. The culture medium of Y79 or RB3823 cells transfected was the same as that of 293T cells transfected. Use concentration of 2 µg/mL puromycin (Sigma Aldrich, St. Louis, MO, USA) screened the target cells with YAP over-expression. After 14 days of continuous screening, stable cells with stable growth of YAP over-expression were selected as YAP stable cells.
Flow cytometry
The level of apoptosis was measured by Annexin V-FITC/PI double staining. First, 0.25% pancreatin without ethylenediaminetetraacetic acid (EDTA) was used to digest Y79 cells and RB3823 cells. Use 1× After the combination of buffer premixed and resuspended, the target cells were treated with Annexin V-FITC and PI (KeyGen, Rockville, MD, USA), and incubated at room temperature and the dark for 30 minutes. After incubation, discard the supernatant and resuspend the cells in 0.5 mL 1× binding buffer. Finally, flow cytometry [Becton, Dickinson, and Co. (BD) Biosciences, Franklin Lakes, NJ, USA] was used to determine the apoptosis results of cell samples within 1 hour.
Intracellular ROS measurement and analysis
Measurement of intracellular ROS and mitochondrial ROS levels
The number of ROS activity in cells was read and determined by fluorescent microscope of fluorescent plate reader and DCFH-DA (D6883, Sigma-Aldrich) staining. In each group of samples, the cells density of 5×104 is inoculated in 6 parallel holes within the 96-well black plates. After inoculating cells for 24 hours, added 10 µM DCFH-DA, dyed in darkness at 37 ℃ for 15 minutes. After the completion of DCFH-DA staining, each well cell sample was washed three times with phosphate buffer saline (PBS), and the level of ROS in the cell was measured by flow cytometer. The activity level of mitochondrial ROS in cell samples was determined by MitoSOX Red (Invitrogen) assay, which is a redox sensitive fluorescent probe for mitochondria in cells. In each group of samples, the cells density of 5×104 is inoculated in 6 parallel holes within the 96-well black plates. After inoculating cells for 24 hours, added 5 mmol/L MitoSOX red probe to dye for 10 minutes in the dark at 37 ℃. After the MitoSOX red probe is dyed, each well cell sample is washed with phosphate buffered saline (PBS) for three times, and the ROS activity level in mitochondria is determined by detecting red fluorescence at 510 and 580 nm excitation and emission wavelengths through Flexstation2 fluorescent plate reader.
Fatty acid-dependent oxygen consumption rate measurement
For mitochondrial fuel flex test, cell preparation and flux cartridges were loaded according to the manufacturer’s instructions. Glucose, glutamine and fatty-acid dependency values were obtained using the Agilent Seahorse XF Mito Fuel Flex Test Kit. Oxygen consumption rate parameters were derived from calculations based on the manufacturer’s instructions. All results were normalized to relative cell numbers per well. Data were analyzed using Wave 2.6.1.
Malondialdehyde (MDA) levels measurement
The lipid peroxidation of RB cells was measured by the synthesis level of MDA. Complete the pretreatment of RB cells according to the experimental requirements. The cells were then washed with PBS and the cell samples were lysed with lysis buffer. After centrifugation, the supernatant was collected and used for MDA detection. Complete the determination of MDA level according to the experimental protocols. Microplate reader (Synergy HT, Biotek, Broadview, IL, USA) was used to measure the absorbance value of cell MDA at 532 nm, reflecting the synthesis level of cell MDA.
Statistical analysis
All data are expressed by mean ± standard deviation (SD). The statistical difference between the two groups were analyzed through the unpaired student t-test. The statistical difference between groups were analyzed by two-way ANOVA multiple comparisons. P<0.05 indicates statistical significance difference. In all data results, *P<0.05, **P<0.01, ***P<0.001.
Results
Over-expression of YAP inhibits the growth and proliferation of RB cells
We used western blot to identify the YAP expression of Y79, RB3823 and SO-RB50 cells selected from human RB cell lines (Human Müller cells as control cells). The results of western blot confirmed that YAP was lowly expressed in human RB cell lines Y79, RB3823 and SO-RB50 (Figure 1A). Therefore, we selected the RB cell lines Y79 and RB3823 with more significantly low expression level of YAP protein to construct YAP over-expression cell lines, and verified them again with western blot, which showed that the constructed Y79 and RB3823 YAP over-expression cell lines were successful (Figure 1B). The results of subsequent MTS assay also indicated that compared with human RB cell lines Y79 and RB3823, the cell proliferation of YAP overexpressed cells was significantly inhibited (Figure 1C,1D). These results indicate that over-expression of YAP can suppress the growth and proliferation of human RB cells.
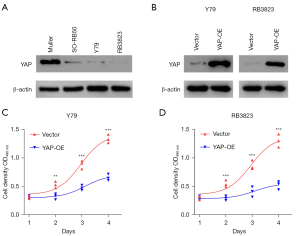
Over-expression of YAP promotes RB cell apoptosis
To explore the effect of YAP over-expression on the growth of human RB cells, we needed to confirm whether the level of apoptosis had changed. The apoptosis level of human RB cell lines Y79 and RB3823 and YAP over-expression cell lines were detected by flow cytometry. Comparing the YAP over-expression cell lines and human RB cell line Y79, the total apoptosis rate (32.72% vs. 3.75%) and the long-term apoptosis rate (14.75% vs. 2.50%) measured by flow cytometry were significantly increased, with significant statistical differences (Figure 2A). Similarly, the YAP over-expression cell line of RB3823 was significantly higher than that of human RB cell line RB3823 in terms of total apoptosis rate (40.32% vs. 6.73%) and long-term apoptosis rate (16.02% vs. 1.74%) measured by flow cytometry, with significant statistical differences (Figure 2B). These results indicate that over-expression of YAP can promote the overall apoptosis level of human RB cells.
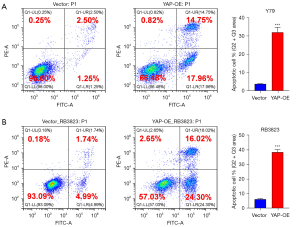
Over-expression of YAP causes the increase of lipid peroxidation level
To identify the cell apoptosis caused by YAP expression, the levels of glucose, glutamine, and FA substrates in human RB cell lines RB3823 and YAP over-expressed cell lines were measured by Agilent Seahorse XF analyzer. Comparing with human RB cell lines, the FA dependency in YAP over-expression cell lines was increased, while the content of glucose was decreased (Figure 3A). Although the over-expression of YAP increased the level of apoptosis, the mechanism of apoptosis was unclear, and there was a correlation between mitochondria and cytoplasm. In order to further verify the correlation, DCFH-DA and MitoSOX measurements indicated that the content of ROS in YAP over-expression cell lines was significantly higher than vector cells both in cytoplasm and mitochondria (Figure 3B,3C). The FA-dependent oxygen consumption rate (OCR) of YAP over-expression cell lines were significantly higher than vector cells (Figure 3D). The above results reveal that YAP over-expression may be related to the mechanism of increased mitochondrial fatty acid β-oxidation, which leading to excessive ROS accumulation. Cellular MDA measurement result suggest that YAP over-expression elevated lipid peroxidation (Figure 3E). Consistent findings were performed by qRT-PCR assay, which showed ferroptosis related markers COX2, ACSL4, PTGS2 and NOX1 were up-regulated, whereas GPX4 and FTH1 were down-regulated. This phenomenon implied that YAP over-expression promoted ferroptosis through lipid peroxidation (Figure 3F).
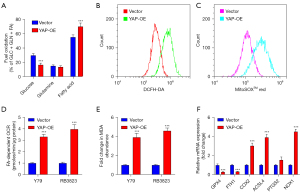
PY-60 promotes apoptosis and inhibits RB cell growth
PY-60 treatment has been confirmed to liberate ANXA2 from the membrane, ultimately generated a transcriptionally active form of YAP. To further investigate the role of YAP activation on RB cells, flow cytometry was used to detect apoptosis of human RB cells after gradient dosage of PY-60 treatment (0, 1, 5, 10 µM). It was confirmed that the total apoptosis rate (3.21% vs. 8.41% vs. 18.44% vs. 34.54%) and long-term apoptosis rate (1.93% vs. 4.16% vs. 6.61% vs. 15.21%) increased significantly with the increase of PY-60 concentration (Figure 4A). The IC50 of PY-60 in human RB cells Y79 and RB3823 were determined by MTS experiment (Figure 4B). Cell proliferation of Y79 and RB3823 cells were suppressed by 10 µM PY-60 confirmed by MTS assay (Figure 4C,4D). These results consistently indicated that transcriptionally active form of YAP induced by PY-60 treatment inhibited RB cells in vitro.
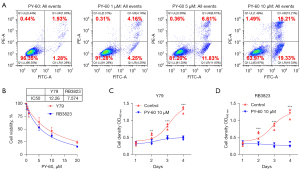
Combination of PY-60 to improve the chemotherapeutic sensitivity of cisplatin and etoposide
Cisplatin and etoposide, as first-line drugs in RB chemotherapy, are often reported to be resistant to chemotherapy. To investigate the therapeutic effect of YAP activation, PY-60 combined with cisplatin or etoposide were used to evaluate the cell viability. MTS assay showed 10 µM PY-60 increased the sensitivity of cisplatin and etoposide in RB cell lines SO-RB50, Y79 and RB3823, respectively (Figure 5A-5F). The combination with PY-60 showed significant suppression when compared with cisplatin or etoposide single drug group in three RB cell lines. These results indicate that PY-60 combined with cisplatin/etoposide can significantly enhance the chemo-sensitivity of human RB in vitro.
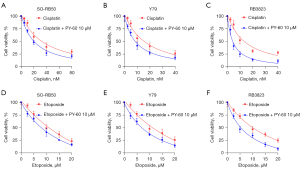
Discussion
RB is the most common ocular malignant tumor in adolescents and children, although platinum chemotherapy has always been the first-line treatment option, it is very prone to chemo-resistance (33,34). In our study, we found that over-expression of YAP can improve the drug sensitivity of platinum in the treatment of human RB cells, which to a certain extent shows that over-expression of YAP may facilitate the occurrence of platinum chemotherapy resistance to a certain extent. The mechanism of resistance to platinum chemotherapy has been described by many authors. Currently, the mainstream opinions include the following aspects: abnormal DNA damage repair function (35), changes in drug cell accumulation (36), intracellular drug inactivation (37), cell autophagy (38), influence on cellular apoptosis pathway (39), drug resistance related gene changes (40), and so on.
Although there are many factors that cause resistance to platinum chemotherapeutic drugs, more studies at present tend to focus on autophagy, drug-resistance related gene changes, and apoptosis. Cisplatin and etoposide will produce a large amount of ROS in the process of action, and excessive accumulation of ROS will have a huge impact on the survival of tumor cells. However, when tumor cells start to change their metabolic microenvironment and inhibit the formation of ROS, and enhance their resistibility to oxidative stress, they can induce the formation of chemotherapy resistance (41). The destruction of redox homeostasis is the indispensable factor for tumor cells to produce chemo-resistance. After chemotherapy, some tumor cells can go through the process of “redox remodeling”, so that the intracellular ROS and antioxidant system are in a high level of balance, and then mediate the drug resistance of tumor cells. Ferroptosis is a new iron dependent and lipid peroxide accumulation cell death mode. It is closely related to the occurrence, development, and chemotherapeutic resistance of tumors, but the specific mechanism causing chemotherapy resistance is still need further study (42).
In our project, we found that over-expression of YAP can improve the sensitivity of RB to chemotherapy, which had not previously been systematically recognized. Our results confirm that YAP over-expression induces ferroptosis/lipid-peroxidation, and that when cisplatin/etoposide is combined with PY-60 (10 µM), it can further improve the sensitivity of RB to platinum drugs. This is consistent with the previous findings of many researchers. When the Hippo signal pathway is out of order, YAP plays a role in promoting the occurrence and development of cancer as a cancer promoting protein. In recent years, mounting studies have found the Hippo signaling pathway to be involved in the development of chemo-resistance of tumors in multiple systems. Trastuzumab is a first-line targeted drug for the treatment of human epidermal growth factor receptor 2 (HER2) positive breast cancer. González-Alonso et al. (43) found that the Hippo signaling pathway effector molecule YAP1/TEAD complex can induce HER2 positive breast cancer to obtain resistance to trastuzumab. In the research on the resistance of non-small cell lung cancer (NSCLC) to cisplatin chemotherapy, when YAP and TAZ genes are knocked out by cells in vitro, the sensitivity of NSCLC to cisplatin can be enhanced (39,44). Ciamporcero et al. confirmed in vivo and in vitro that the expression and activation of the Hippo pathway effector molecule YAP can protect urothelial carcinoma cells from DNA damage induced by chemotherapy and radiation, and is negatively related to the sensitivity of urothelial carcinoma cells to cisplatin (45). In addition, YAP can induce the expression of cyclooxygenase-2 to increase the drug resistance of colorectal cancer to paclitaxel (46). However, the specific molecular mechanism of how the relevant molecules in the Hippo signaling pathway participate in drug resistance has not been fully clarified, and whether it can reverse the drug resistance of targeted drugs and chemotherapy through the Hippo signaling pathway deserves further exploration.
Many researchers have proposed that abnormal iron metabolism, especially iron overload, is highly correlated with tumor chemotherapy resistance. Iron overload in tumor cells can catalyze the production of ROS, which can satisfy the basic needs of tumor cell proliferation to a certain extent; However, when tumor cells were treated with chemotherapy drugs, a large number of ROS will be induced, which will have a huge impact on the biological activity of tumor cells. Once the tumor cell starts the mechanism of changing its metabolic microenvironment, it will inhibit the further generation of ROS, they will induce the occurrence of chemotherapy resistance (47). To date, many intracellular factors related to mitochondrial ferroptosis have been reported, such as COX2, ACSL4, PTGS2, and NOX1, which are up-regulated in ferroptosis cells (48), whereas GPX4 and FTH1 are down regulated in ferroptosis cells (49). Our experimental results also support the feasibility of the above views. In this regard, Guo et al. (50) found that cisplatin can simultaneously induce apoptosis and ferroptosis after treating multiple tumor cells. At present, the known mechanism of chemotherapy resistance has an effect on cisplatin-induced apoptosis, but has no effect on cisplatin-induced ferroptosis.
Therefore, ferroptosis, as a completely independent new mode of regulating cell apoptosis, opens up a new way to effectively solve the resistance of tumor cells to cisplatin chemotherapy. The combination of cisplatin and ferroptosis inducer Erastin has a significant enhancing effect on its anti-tumor activity. Sato et al. (51) finished pretreatment of cisplatin-resistant ovarian cancer cells with Elastin, the results suggest that Elastin can promote the sensitivity of cisplatin chemotherapy, reflecting its good synergy with cisplatin chemotherapy. In addition, Roh et al. (52) found that Erastin can reverse the chemotherapy resistance of head and neck tumors to cisplatin. Erastin significantly enhanced the anti-tumor activity of the first-line chemotherapy drugs cytarabine and doxorubicin in HL60 cells. Induction of ferroptosis can overcome the drug resistance of acute myeloid leukemia (AML) cells. In addition, in some central primary tumor cells, Erastin can enhance the efficacy of chemotherapy drugs (such as temozolomide, cisplatin, cytarabine, and doxorubicin). However, ferroptosis inducers can not promote the chemo-sensitivity of cisplatin in all tumor cells. Yang et al. (53) found that RAS gene mutation caused iron overload in cells, which increased the sensitivity of tumor cells to ferroptosis inducers. In clinical practice, it is known that 20% of human tumors have active mutations in RAS oncogene, which is always related to drug resistance, providing an important basis for guiding the clinical prognosis and treatment selection of patients with related tumors (54,55). However, the subtype of ferroptosis sensitive tumors is still uncertain. According to the sensitivity analysis of 117 tumor cell lines to Erasin, the sensitivity of RAS mutant tumor cell lines to ferroptosis inducers is not higher than the normal control tumor cells, and RAS mutation is not enough to be used as a marker for its determination. Further exploration is needed to find more accurate prognostic markers (49).
In summary, we found that over-expression of YAP was beneficial to improve the overall effect of platinum chemotherapy; YAP over-expression can improve the efficacy of cisplatin by inducing ferroptosis/lipid-peroxidation and cooperating with ferroptosis related upstream and downstream gene pathways. In future, we will conduct more in-depth research on its specific mechanism, and further determine the platinum chemo-resistance subtype related to the expression of YAP. The specific drugs that utilize the over-expression of YAP will have the opportunity to become a potential new hotspot in the treatment of RB patients.
Conclusions
Ferroptosis plays a key role in the proliferation and chemo-resistance of multiple systemic tumors; However, it has not been paid attention to in the field of RB treatment. RB has been confirmed to be a tumor with reduced expression of YAP; our research results found that the over-expression of YAP induced iron cell apoptosis/lipid-peroxidation in RB and improved the sensitivity of RB cells to cisplatin/etoposide chemotherapy. However, there are still some problems that deserve our continued attention and efforts to explore new results: (I) the internal relationship between ferroptosis and other forms of apoptosis in RB is not clear now; (II) whether iron ion-related preparations can promote the therapeutic effect of RB chemotherapy; (III) what are the changes in core metabolites of fatty acid metabolism caused by lipid peroxidation; (IV) whether there are other metabolic pathway changes that can affect the therapeutic effect of chemotherapy drugs on RB; (V) how to use the related target of ferroptosis as soon as possible to predict the therapeutic effect of clinical patients. At present, we are actively exploring the specific mechanism of ferroptosis and drug resistance in RB. To clarify the specific mechanism of ferroptosis/lipid peroxidation on chemotherapy resistance of RB provides a lot of reference basis for improving the therapeutic effect of RB, will further guide front-line clinical doctors to build an accurate molecular prediction model for the treatment of RB patients, which is helpful to improve the overall treatment effect and survival time of RB patients.
Acknowledgments
Funding: This study was supported by Special Fund for Major R&D of China National Health Commission (No. 2021KYSHX01508), National Natural Science Foundation of China (No. 82271081), and the Natural Science Foundation of Guangdong Province (No. 2022A1515010302).
Footnote
Reporting Checklist: The authors have completed the MDAR reporting checklist. Available at https://cco.amegroups.com/article/view/10.21037/cco-23-97/rc
Data Sharing Statement: Available at https://cco.amegroups.com/article/view/10.21037/cco-23-97/dss
Peer Review File: Available at https://cco.amegroups.com/article/view/10.21037/cco-23-97/prf
Conflicts of Interest: All authors have completed the ICMJE uniform disclosure form (available at https://cco.amegroups.com/article/view/10.21037/cco-23-97/coif). The authors have no conflicts of interest to declare.
Ethical Statement: The authors are accountable for all aspects of the work in ensuring that questions related to the accuracy or integrity of any part of the work are appropriately investigated and resolved.
Open Access Statement: This is an Open Access article distributed in accordance with the Creative Commons Attribution-NonCommercial-NoDerivs 4.0 International License (CC BY-NC-ND 4.0), which permits the non-commercial replication and distribution of the article with the strict proviso that no changes or edits are made and the original work is properly cited (including links to both the formal publication through the relevant DOI and the license). See: https://creativecommons.org/licenses/by-nc-nd/4.0/.
References
- Jansen RW, van der Heide S, Cardoen L, et al. Magnetic Resonance Imaging Can Reliably Differentiate Optic Nerve Inflammation from Tumor Invasion in Retinoblastoma with Orbital Cellulitis. Ophthalmology 2022;129:1275-86. [Crossref] [PubMed]
- Wen Y, Zhu M, Zhang X, et al. Integrated analysis of multiple bioinformatics studies to identify microRNA-target gene-transcription factor regulatory networks in retinoblastoma. Transl Cancer Res 2022;11:2225-37. [Crossref] [PubMed]
- Zhang JJ, Du J, Kong N, et al. Mechanisms and pharmacological applications of ferroptosis: a narrative review. Ann Transl Med 2021;9:1503. [Crossref] [PubMed]
- Jiang H, Niu C, Guo Y, et al. Wedelolactone induces apoptosis and pyroptosis in retinoblastoma through promoting ROS generation. Int Immunopharmacol 2022;111:108855. [Crossref] [PubMed]
- Sweid A, El Naamani K, Sajja KC, et al. Incidence and predictors of ophthalmic artery occlusion in intra-arterial chemotherapy for retinoblastoma. J Neurointerv Surg 2021;13:652-6. [Crossref] [PubMed]
- Runnels J, Acosta G, Rose A, et al. The role for intra-arterial chemotherapy for refractory retinoblastoma: a systematic review. Clin Transl Oncol 2021;23:2066-77. [Crossref] [PubMed]
- Kong M, Han Y, Zhao Y, et al. miR-512-3p Overcomes Resistance to Cisplatin in Retinoblastoma by Promoting Apoptosis Induced by Endoplasmic Reticulum Stress. Med Sci Monit 2020;26:e923817. [Crossref] [PubMed]
- Bogan CM, Kaczmarek JV, Pierce JM, et al. Evaluation of intravitreal topotecan dose levels, toxicity and efficacy for retinoblastoma vitreous seeds: a preclinical and clinical study. Br J Ophthalmol 2022;106:288-96. [Crossref] [PubMed]
- Hu JL, Wang W, Lan XL, et al. CAFs secreted exosomes promote metastasis and chemotherapy resistance by enhancing cell stemness and epithelial-mesenchymal transition in colorectal cancer. Mol Cancer 2019;18:91. [Crossref] [PubMed]
- Hu F, Song D, Yan Y, et al. IL-6 regulates autophagy and chemotherapy resistance by promoting BECN1 phosphorylation. Nat Commun 2021;12:3651. [Crossref] [PubMed]
- Cui C, Yang J, Li X, et al. Functions and mechanisms of circular RNAs in cancer radiotherapy and chemotherapy resistance. Mol Cancer 2020;19:58. [Crossref] [PubMed]
- Reinhard J, Wagner N, Krämer MM, et al. Expression Changes and Impact of the Extracellular Matrix on Etoposide Resistant Human Retinoblastoma Cell Lines. Int J Mol Sci 2020;21:4322. [Crossref] [PubMed]
- Kakkassery V, Gemoll T, Kraemer MM, et al. Protein Profiling of WERI-RB1 and Etoposide-Resistant WERI-ETOR Reveals New Insights into Topoisomerase Inhibitor Resistance in Retinoblastoma. Int J Mol Sci 2022;23:4058. [Crossref] [PubMed]
- Shen Y, Wang X, Liu Y, et al. STAT3-YAP/TAZ signaling in endothelial cells promotes tumor angiogenesis. Sci Signal 2021;14:eabj8393. [Crossref] [PubMed]
- Wang H, Zhang S, Zhang Y, et al. TAZ is indispensable for c-MYC-induced hepatocarcinogenesis. J Hepatol 2022;76:123-34. [Crossref] [PubMed]
- Zinatizadeh MR, Miri SR, Zarandi PK, et al. The Hippo Tumor Suppressor Pathway (YAP/TAZ/TEAD/MST/LATS) and EGFR-RAS-RAF-MEK in cancer metastasis. Genes Dis 2019;8:48-60. [Crossref] [PubMed]
- Zhou X, Li Y, Wang W, et al. Regulation of Hippo/YAP signaling and Esophageal Squamous Carcinoma progression by an E3 ubiquitin ligase PARK2. Theranostics 2020;10:9443-57. [Crossref] [PubMed]
- Wang R, Zhu G. A narrative review for the Hippo-YAP pathway in cancer survival and immunity: the Yin-Yang dynamics. Transl Cancer Res 2022;11:262-75. [Crossref] [PubMed]
- Fan H, Wang X, Li W, et al. ASB13 inhibits breast cancer metastasis through promoting SNAI2 degradation and relieving its transcriptional repression of YAP. Genes Dev 2020;34:1359-72. [Crossref] [PubMed]
- Kurppa KJ, Liu Y, To C, et al. Treatment-Induced Tumor Dormancy through YAP-Mediated Transcriptional Reprogramming of the Apoptotic Pathway. Cancer Cell 2020;37:104-122.e12. [Crossref] [PubMed]
- Suemura S, Kodama T, Myojin Y, et al. CRISPR Loss-of-Function Screen Identifies the Hippo Signaling Pathway as the Mediator of Regorafenib Efficacy in Hepatocellular Carcinoma. Cancers (Basel) 2019;11:1362. [Crossref] [PubMed]
- Gao J, Han W, He Y, et al. Livin promotes tumor progression through YAP activation in ovarian cancer. Am J Cancer Res 2020;10:3179-93. [PubMed]
- Abudoukerimu A, Hasimu A, Abudoukerimu A, et al. HIF-1α Regulates the Progression of Cervical Cancer by Targeting YAP/TAZ. J Oncol 2022;2022:3814809. [Crossref] [PubMed]
- Cheng Y, Huang H, Han Y, et al. Expression of YAP in endometrial carcinoma tissues and its effect on epithelial to mesenchymal transition. Transl Cancer Res 2020;9:7248-58. [Crossref] [PubMed]
- Gu Y, Chen Y, Wei L, et al. ABHD5 inhibits YAP-induced c-Met over-expression and colon cancer cell stemness via suppressing YAP methylation. Nat Commun 2021;12:6711. [Crossref] [PubMed]
- Song L, Huang Y, Zhang X, et al. Downregulation of microRNA-224-3p Hampers Retinoblastoma Progression via Activation of the Hippo-YAP Signaling Pathway by Increasing LATS2. Invest Ophthalmol Vis Sci 2020;61:32. [Crossref] [PubMed]
- Staley BK, Irvine KD. Hippo signaling in Drosophila: recent advances and insights. Dev Dyn 2012;241:3-15. [Crossref] [PubMed]
- Tang D, Chen X, Kang R, et al. Ferroptosis: molecular mechanisms and health implications. Cell Res 2021;31:107-25. [Crossref] [PubMed]
- Yang WS, Stockwell BR. Ferroptosis: Death by Lipid Peroxidation. Trends Cell Biol 2016;26:165-76. [Crossref] [PubMed]
- Zhang Y, Zhou L, Wang S, et al. Exploration of retinoblastoma pathogenesis with bioinformatics. Transl Cancer Res 2021;10:3527-37. [Crossref] [PubMed]
- Guo N. Identification of ACSL4 as a biomarker and contributor of ferroptosis in clear cell renal cell carcinoma. Transl Cancer Res 2022;11:2688-99. [Crossref] [PubMed]
- Yu M, Gai C, Li Z, et al. Targeted exosome-encapsulated erastin induced ferroptosis in triple negative breast cancer cells. Cancer Sci 2019;110:3173-82. [Crossref] [PubMed]
- Busch M, Papior D, Stephan H, et al. Characterization of etoposide- and cisplatin-chemoresistant retinoblastoma cell lines. Oncol Rep 2018;39:160-72. [PubMed]
- Li Z, Zhang L, Liu D, et al. Knockdown of NRMT enhances sensitivity of retinoblastoma cells to cisplatin through upregulation of the CENPA/Myc/Bcl2 axis. Cell Death Discov 2022;8:14. [Crossref] [PubMed]
- Guo J, Jin D, Wu Y, et al. The miR 495-UBE2C-ABCG2/ERCC1 axis reverses cisplatin resistance by downregulating drug resistance genes in cisplatin-resistant non-small cell lung cancer cells. EBioMedicine 2018;35:204-21. [Crossref] [PubMed]
- Liao XZ, Gao Y, Sun LL, et al. Rosmarinic acid reverses non-small cell lung cancer cisplatin resistance by activating the MAPK signaling pathway. Phytother Res 2020;34:1142-53. [Crossref] [PubMed]
- Lan D, Wang L, He R, et al. Exogenous glutathione contributes to cisplatin resistance in lung cancer A549 cells. Am J Transl Res 2018;10:1295-309. [PubMed]
- Wang H, Chen J, Zhang S, et al. MiR-223 regulates autophagy associated with cisplatin resistance by targeting FBXW7 in human non-small cell lung cancer. Cancer Cell Int 2020;20:258. [Crossref] [PubMed]
- Song J, Xie LX, Zhang XY, et al. Role of YAP in lung cancer resistance to cisplatin. Oncol Lett 2018;16:3949-54. [Crossref] [PubMed]
- He R, Liu H. TRIM59 knockdown blocks cisplatin resistance in A549/DDP cells through regulating PTEN/AKT/HK2. Gene 2020;747:144553. [Crossref] [PubMed]
- Lee KM, Giltnane JM, Balko JM, et al. MYC and MCL1 Cooperatively Promote Chemotherapy-Resistant Breast Cancer Stem Cells via Regulation of Mitochondrial Oxidative Phosphorylation. Cell Metab 2017;26:633-647.e7. [Crossref] [PubMed]
- Wang Y, Zheng L, Shang W, et al. Wnt/beta-catenin signaling confers ferroptosis resistance by targeting GPX4 in gastric cancer. Cell Death Differ 2022;29:2190-202. [Crossref] [PubMed]
- González-Alonso P, Zazo S, Martín-Aparicio E, et al. The Hippo Pathway Transducers YAP1/TEAD Induce Acquired Resistance to Trastuzumab in HER2-Positive Breast Cancer. Cancers (Basel) 2020;12:1108. [Crossref] [PubMed]
- Xu W, Wei Y, Li Y, et al. TAZ inhibition restores sensitivity of cisplatin via AKT/mTOR signaling in lung adenocarcinoma. Oncol Rep 2017;38:1815-21. [Crossref] [PubMed]
- Ciamporcero E, Shen H, Ramakrishnan S, et al. YAP activation protects urothelial cell carcinoma from treatment-induced DNA damage. Oncogene 2016;35:1541-53. [Crossref] [PubMed]
- Li W, Cao Y, Xu J, et al. YAP transcriptionally regulates COX-2 expression and GCCSysm-4 (G-4), a dual YAP/COX-2 inhibitor, overcomes drug resistance in colorectal cancer. J Exp Clin Cancer Res 2017;36:144. [Crossref] [PubMed]
- Galadari S, Rahman A, Pallichankandy S, et al. Reactive oxygen species and cancer paradox: To promote or to suppress? Free Radic Biol Med 2017;104:144-64. [Crossref] [PubMed]
- Zhang HL, Hu BX, Li ZL, et al. PKCβII phosphorylates ACSL4 to amplify lipid peroxidation to induce ferroptosis. Nat Cell Biol 2022;24:88-98. [Crossref] [PubMed]
- Yang WS. Regulation of ferroptotic cancer cell death by GPX4. Cell 2014;156:317-31. [Crossref] [PubMed]
- Guo J, Xu B, Han Q, et al. Ferroptosis: A Novel Anti-tumor Action for Cisplatin. Cancer Res Treat 2018;50:445-60. [Crossref] [PubMed]
- Sato M, Kusumi R, Hamashima S, et al. The ferroptosis inducer erastin irreversibly inhibits system x(c)- and synergizes with cisplatin to increase cisplatin’s cytotoxicity in cancer cells. Sci Rep 2018;8:968. [Crossref] [PubMed]
- Roh JL, Kim EH, Jang HJ, et al. Induction of ferroptotic cell death for overcoming cisplatin resistance of head and neck cancer. Cancer Lett 2016;381:96-103. [Crossref] [PubMed]
- Yang WS, Stockwell BR. Synthetic lethal screening identifies compounds activating iron-dependent, nonapoptotic cell death in oncogenic-RAS-harboring cancer cells. Chem Biol 2008;15:234-45. [Crossref] [PubMed]
- Young A, Lou D, McCormick F. Oncogenic and wild-type Ras play divergent roles in the regulation of mitogen-activated protein kinase signaling. Cancer Discov 2013;3:112-23. [Crossref] [PubMed]
- Steuer CE, Behera M, Berry L, et al. Role of race in oncogenic driver prevalence and outcomes in lung adenocarcinoma: Results from the Lung Cancer Mutation Consortium. Cancer 2016;122:766-72. [Crossref] [PubMed]