Twenty-five years with companion diagnostics
Introduction
For decades, pharmacotherapy has been hampered by significant patient variability in terms of treatment outcome. Owing to pronounced heterogeneity, patients with apparently the same diagnosis often respond differently to an identical pharmacological intervention, which applies to both efficacy and possible side effects. A survey conducted at the beginning of this century on a number of major drugs within several important disease areas showed considerable variability in terms of efficacy (1). For the majority of drugs included in this survey, efficacy rates ranged between 40% and 60%, with some disease areas experiencing even lower rates. For instance, the use of chemotherapy in oncology yielded an estimated average efficacy rate of 25%. Our inability to predict the outcome of a pharmacological intervention in an individual patient has negatively impacted the value of pharmacotherapy. However, over the past 30–40 years, advances in molecular medicine have increased our understanding of the pathophysiology and mechanisms of action of drugs. This understanding has led to significant advancements in hematology and oncology, and the use of predictive biomarkers has played an important role in improving treatment outcomes. This type of predictive biomarker assay has attracted the attention of the regulator agencies, where they are referred to as companion diagnostic (CDx) (2). This article provides an overview of the evolution of the CDx area since the Food and Drug Administration (FDA) approved the first drug, along with an accompanying assay, a quarter century ago.
Trastuzumab and HercepTest
In 1987, an article published in Science described the association between HER2 amplification and poor prognosis in women with breast cancer. Based on this observation, the authors concluded that the HER2 gene product might function as a growth factor receptor involved in the pathogenesis of the disease (3). Furthermore, the authors suggested that a receptor antagonist could have significant therapeutic implications. Years later, the antagonist mentioned in the Science article became the monoclonal antibody trastuzumab, and in the early 1990s, when Genentech initiated the clinical development of the drug, they developed an immunohistochemical (IHC) assay for detecting HER2 protein expression in tumor tissue (4). Preclinical studies had demonstrated an association between the level of HER2 expression and the ability of trastuzumab to inhibit tumor growth (5,6). This information was crucial for the clinical development in order to identify the right patient population for treatment with trastuzumab and thereby be able to enrich the trial populations with HER2 positive patients. Throughout phases I to III, this IHC assay was utilized for patient stratification, and in these clinical trials, a clear correlation between HER2 overexpression and the efficacy of trastuzumab was further confirmed (7-9).
On September 25, 1998, the FDA granted approval to trastuzumab for the treatment of HER2-positive metastatic breast cancer in conjunction with the IHC assay HercepTest, which was developed by the diagnostic company Dako (Glostrup, Denmark) (4). The concurrent approval of trastuzumab and the HercepTest was meaningful, as the HER2 assay must be accessible simultaneously with the drug to select the right patients for treatment (10). Since then, the FDA has adopted a policy of simultaneous approval of drugs and diagnostics, mainly in the field of hematology and oncology. The HercepTest became the first regulatory approved CDx but certainly not the last. As of August 2023, the FDA has approved more than 60 drugs and drug combinations, along with a CDx assay (11).
CDx
According to the FDA-National Institutes of Health (NIH) Biomarker Working Group, a predictive biomarker is defined as a biomarker used to identify individuals who are more likely than similar individuals without the biomarker to experience a favorable or unfavorable effect from exposure to a medical product or an environmental agent (12). The most common predictive biomarkers for pharmacotherapy are selected based on the mechanism of action of the drug and the understanding of the pathophysiology. These biomarkers often represent patient characteristics such as molecular alterations related to deoxyribonucleic acid (DNA), messenger ribonucleic acid (mRNA), receptors, enzymes, or signal proteins. When a predictive biomarker is associated with a specific drug or drug class, regulatory agencies employ the term CDx, which the FDA defines as an in vitro diagnostic device that provides information that is essential for the safe and effective use of a corresponding therapeutic product (13). The FDA has identified four areas where a CDx assay could be essential: (I) to identify patients who are most likely to benefit from the therapeutic product; (II) to identify patients likely to be at increased risk of serious adverse reactions as a result of treatment with the therapeutic product; (III) to monitor the response to treatment with the therapeutic product for the purpose of adjusting treatment (e.g., schedule, dose, discontinuation) to achieve improved safety or effectiveness; and (IV) to identify patients in the population for whom the therapeutic product has been adequately studied and found safe and effective, i.e., there is insufficient information about the safety and effectiveness of the therapeutic product in any other population. The fourth element mentioned in the definition is related to the type of trial design most often used for the clinical validation of CDx assays: the enrichment trial design (2). Please note that the extrapolation of clinical outcome data cannot be applied to populations beyond those defined by the CDx assay, as stated by the FDA, and any prescription of a drug outside this population will be considered an off-label prescription (13). The German Federal Institute for Drugs and Medical Devices have expressed similar opinions regarding CDx testing and off-label prescriptions (14).
In 1998, when the FDA granted approval for the HercepTest, this category of assays was referred to as pharmacodiagnostics or theranostics. The term CDx was introduced years later in an article published in Nature Biotechnology that discussed targeted cancer therapy and molecular diagnostics (15). In this article, the authors posited that the therapeutic potential of the drug in question would only be realized with the concurrent development of a CDx assay. They emphasized that such an assay could simplify the drug discovery process and render clinical trials more efficient and informative. Moreover, the authors underlined that the use of molecular diagnostics held the promise of tailoring therapy to individual patients, which is why this approach was closely associated with the concept of personalized medicine (16). In 2014, when the FDA issued their regulatory guidance document on In Vitro Companion Diagnostic Devices, they adopted the name CDx (13). Globally, other regulatory bodies, including the Australian Therapeutic Goods Administration (TGA), the Canadian Health Care Service Corporation (HCSC), the Japanese Pharmaceuticals and Medical Devices Agency (PMDA), and the Korean Ministry of Food and Drug Safety (MFDS), have issued similar regulatory guidance documents for CDx (17,18). In May 2022, the new In Vitro Diagnostic Regulation (IVDR) came into force in Europe, which introduced a number of changes to the legal framework for CDx in the European Union (19-21). For CDx assays in Europe, it is expected that the new IVDR will be fully implemented by May 2026.
CDx platforms
CDx assays encompass a variety of analytical platforms, each with distinct methodologies. The first FDA-approved CDx assay employed IHC, which was the HercepTest assay, intended to determine HER2 overexpression in breast cancer tissue specimens, and used as an aid in the assessment of patients for whom trastuzumab treatment was considered (10). In 2001, a few years after the approval of HercepTest, the first fluorescence in situ hybridization (FISH) assay, the PathVysion HER2 DNA Probe Kit, received FDA approval. The intended use of this assay is to detect HER2 gene amplification in breast cancer tissue specimens, and used as an aid in assessing patients eligible for treatment with trastuzumab, just like the HercepTest (11).
A decade later, a new CDx platform, real-time polymerase chain reaction (PCR), was introduced. The first assay of this type was the Cobas 4800 BRAF V600 Mutation Test, intended for identifying the BRAF V600E mutation in melanoma tissue and used as an aid in selecting patients for treatment with vemurafenib (11). In 2013, a somewhat different CDx platform emerged with the FDA’s approval of the FerriScan, a magnetic resonance imaging (MRI) device to monitor liver iron concentration. This imaging CDx is intended as an aid in the identification and monitoring of non-transfusion-dependent thalassemia patients receiving deferasirox (11). The introduction of next-generation sequencing (NGS) as a CDx platform came with the approval of the FoundationFocus CDxBRCA Assay in 2016. This assay is intended for the qualitative detection of BRCA1 and BRCA2 gene alterations in ovarian tumor tissue and is used as an aid in identifying ovarian cancer patients for whom treatment with rucaparib is being considered (11). The most recent analytical platform to achieve FDA approval as a CDx is the electrochemiluminescence immunoassay (ECLIA). In June 2023, the AAV5 DetectCDx assay gained regulatory approval as an aid in the selection of adult hemophilia A patient’s being considered for valoctocogene roxaparvovec treatment (11). Figure 1 provides a timeline illustrating the introduction and regulatory approval of the different CDx platforms.
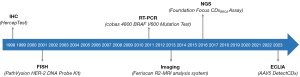
At the end of August 2023, the FDA has cleared or approved a total of 56 CDx assays (11). As shown in Figure 2, PCR-based assays constitute the largest category, accounting for 34%, followed by IHC assays at 25%. NGS, in situ hybridization (ISH), ECLIA, and MRI constitute 19%, 18%, 2%, and 2% of the cases, respectively. The figures for ISH included both FISH and chromogenic in situ hybridization (CISH) assays. The majority of the assays are related to oncological drugs and designed to test tissue biopsies. However, in the past 5 to 6 years, PCR and NGS-based assays have emerged and gained approval for liquid biopsies, which involve the analysis of circulating cell-free DNA from plasma (22,23). Examples of such assays are the Cobas EGFR Mutation Test v2, Guardant360 CDx, and FoundationOne Liquid CDx (11). However, it is important to note that a negative plasma specimen result does not necessarily confirm the absence of the specific genomic alterations in the patient’s tumor. Owing to this limitation, the FDA has included a statement for this type of assay, indicating that patients with a negative mutation test result should undergo routine biopsy, and their tumor mutation status should be verified using an FDA-approved tumor tissue test (24-26).
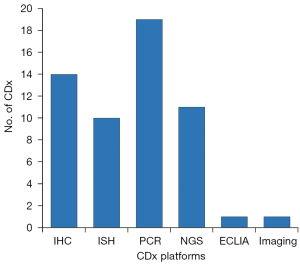
Drug CDx combinations
Since the FDA approved the first CDx in 1998, the number of this type of assays has steadily increased, as illustrated in Figure 3. The increasing number of CDx assays reflects what has happened within oncology drug development in the past 25 years with respect to the regulatory approval of new targeted therapies. Until 2010, the number of CDx assays remained relatively modest and was largely dominated by IHC and ISH. However, significant growth in the CDx assays was observed a decade ago, with the introduction of PCR- and NGS-based assays. As of August 2023, the number of drugs and drug combinations with a CDx assay linked to their use has reached 65. Table 1 provides a list of FDA-approved drugs and their respective biomarkers (11,27). Among the 58 drugs listed in Table 1, only deferasirox, setmelanotide acetate, and valoctocogene roxaparvovec are not for hematological and oncological indications. Deferasirox, as previously mentioned, addresses chronic iron overload in individuals with non-transfusion-dependent thalassemia, whereas setmelanotide is indicated for chronic weight management in patients with monogenic or syndromic obesity. Valoctocogene roxaparvovec is a recently approved adeno-associated virus vector-based gene therapy indicated for treating adults with severe hemophilia A without preexisting antibodies to adeno-associated virus serotype 5 (11,27). In addition to the CDx assays linked to the individual drugs in Table 1, the FDA has approved assays for a few drug combinations, as detailed in Table 2 (11).
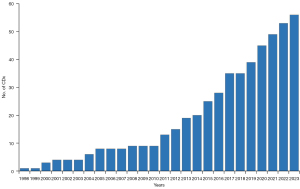
Table 1
Biomarker | Drug (generic name) |
---|---|
ALK/ALK | Alectinib; brigatinib; ceritinib; crizotinib; lorlatinib |
Anti-AAV5 Antibodies | Valoctocogene roxaparvovec* |
BRAF | Dabrafenib; trametinib; vemurafenib |
BRCA1/BRCA2 | Niraparib; olaparib; rucaparib; talazoparib |
BRCA1/BRCA2/ATM | Olaparib |
c-KIT/KIT | Imatinib mesylate |
dMMR | Dostarlimab; pembrolizumab |
EGFR/EGFR | Afatinib; amivantamab; cetuximab; dacomitinib; erlotinib; gefitinib; mobocertinib; osimertinib; panitumumab |
ESR1 | Elacestrant |
EZH2 | Tazemetostat |
FGFR2 | Infigratinib; pemigatinib |
FGFR3 | Erdafitinib |
FLT3 | Gilteritinib; midostaurin; quizartinib |
FOLR1 | Mirvetuximab soravtansine |
HER2/HER2 | Pertuzumab; trastuzumab; trastuzumab deruxtecan; trastuzumab emtansine |
HLA | Tebentafusp |
IDH1 | Ivosidenib; olutasidenib |
IDH2 | Enasidenib |
Ki-67 | Abemaciclib |
KRAS | Cetuximab; panitumumab |
KRAS/NRAS | Panitumumab |
KRAS G12C | Sotorasib, adagrasib |
Liver imaging | Deferasirox* |
MET | Capmatinib |
MSI-H | Pembrolizumab |
NTRK1/2/3 | Entrectinib; larotrectinib |
PDGFRA | Avapritinib |
PDGFRB | Imatinib mesylate |
PD-L1 | Atezolizumab; cemiplimab; pembrolizumab |
PIK3CA | Alpelisib |
POMC/PCSK1/ LEPR | Setmelanotide acetate* |
RET | Pralsetinib; selpercatinib |
ROS1 | Crizotinib; entrectinib |
t(9;21) Ph chromosome | Nilotinib |
TMB-H | Pembrolizumab |
TP53 | Venetoclax |
*, non-oncological and hematological drugs. FDA, Food and Drug Administration.
Table 2
Biomarker | Drugs (generic name) |
---|---|
BRAF | Atezolizumab in combination with cobimetinib and vemurafenib |
Cobimetinib in combination with vemurafenib | |
Dabrafenib in combination with trametinib | |
Encorafenib in combination with binimetinib | |
Encorafenib in combination with cetuximab | |
PD-L1 | Nivolumab in combination with ipilimumab |
pMMR | Pembrolizumab in combination with lenvatinib |
FDA, Food and Drug Administration; PD-L1, programmed death-ligand 1; pMMR, proficient mismatch repair.
The CDx assays have played a significant role in the clinical development of the drugs listed in Tables 1,2. In the drug-diagnostic codevelopment model, a CDx assay is developed in parallel with the drug and subsequently used for patient stratification to enrich the trial population with likely responding patients (2). This enrichment design has enabled to demonstrate drug efficacy in relatively small-scale clinical trials (28). Over the past decade, numerous targeted hematological and oncological drugs have received regulatory approval through one of the FDA’s expedited programs based on a clinical development program comprising one or two small-sized, non-randomized, single-arm enrichment trials. These FDA-expedited programs aim to facilitate an expedite development and review of new drugs that address unmet medical needs in the treatment of severe or life-threatening conditions (29,30).
For some of the drugs listed in Table 1, the total number of patients included in the clinical development program to demonstrate efficacy was less than 200. This holds true for drugs, such as ceritinib, gilteritinib, olaparib, pralsetinib, and sotorasib (27). A few drugs, such as amivantamab, capmatinib, and erdafitinib, along with tissue-agnostic drugs, such as entrectinib and larotrectinib, have demonstrated efficacy in biomarker enriched trial populations consisting of fewer than 100 patients (28,31-35). Addressing the small sample sizes in these clinical trials, an important aspect to remember is that a large number of the drugs listed in Table 1 are classified as orphan drugs. When a population of cancer patients is subdivided based on molecular testing, the number of patients with a specific characteristic can be limited. The prevalence of several driver mutations involved in the pathogenesis of non-small cell lung cancer is low. For instance, ROS1-positivity is identified in 1–2% of patients, while the MET exon 14 skipping mutation appears in 3–4% (36). For patients with solid tumors harboring NRTK fusions who are candidates for treatment with the tissue agnostic drugs entrectinib and larotrectinib, the prevalence of these gene fusions is on average less than 1% (37).
The drug-diagnostic codevelopment model has been studied extensively using data from various public databases, and the results of these studies suggest that integrating CDx assays into clinical drug development can significantly increase the likelihood of achieving a successful outcome (38,39). Specifically, for hematological and oncological drugs, the biomarker-based enrichment trial design has been shown to improve the success rate by a significant margin compared to the traditional all-comers design. Furthermore, the implementation of the drug-diagnostic codevelopment strategy not only improves the probability of success but also reduces both the cost and time spent on clinical development activities. These findings have been corroborated by multiple studies, which collectively support the potential benefits of this approach to drug development.
Following regulatory approval, the CDx assays play a pivotal role in ensuring optimal therapy for the individual patients in the clinical setting. This emphasizes the importance of achieving simultaneous regulatory approval of both drugs and diagnostics. Most CDx assays have been prospectively developed in parallel with drugs, facilitating simultaneous approval (2,40). However, in recent years, several targeted oncological drugs and new indications have gained FDA approval without a corresponding CDx, although a predictive biomarker assay has been used for patient selection during clinical development (41). The lack of a CDx assay could have implications for the correct use of these drugs, and ultimately impact patient safety. Therefore, regulatory bodies and pharmaceutical companies need to take an active stance to avoid such situations.
Tissue agnostic drugs
In 2017, the FDA approved the programmed death 1 inhibitor pembrolizumab for the treatment of adult and pediatric patients with unresectable or metastatic microsatellite instability-high (MSI-H) or deficient mismatch repair (dMMR) solid tumors, regardless of tumor site or histology (27,42,43). Remarkably, the FDA did not approve pembrolizumab for a specific cancer type but for all patients with MSI-H or dMMR solid tumors who fulfilled the specific indication requirements. Following this approval, an article appeared in the New England Journal of Medicine written by medical reviewers at the FDA with the title: ‘First FDA Approval Agnostic of Cancer Site - When a Biomarker Defines the Indication’ (42). For decades, cancers have been classified according to their site of origin and histology, which have also been determinative when specifying indications for oncological drugs; however, for some molecular aberrations, this seems secondary, and here it is a specific biomarker that defines the indication (44). For MSI-H and dMMR indications for pembrolizumab, two CDx assays have been developed: the NGS assay FoundationOne CDx for detection of MSI-H and the IHC assay Ventana MMR RxDx Panel for detection of dMMR (11).
Pembrolizumab is not the only drug approved by the FDA for a tissue-agnostic indication. In 2018 and 2019, the tropomyosin receptor kinase (TRK) inhibitors, larotrectinib and entrectinib were approved for patients with solid tumors harboring a neurotrophic receptor tyrosine kinase (NTRK) gene fusion (27). Subsequently, more drugs have followed, and Table 3 lists the FDA-approved tissue agnostic drugs and drug combinations together with their biomarkers. Molecular testing for tissue-agnostic drugs is central in order to identify the correct patient population for treatment. Thus, the development of CDx requires specific attention, and the parallel development of drugs and diagnostics is crucial to ensure that the assay is regulatory approved simultaneously with the drug, as it is a biomarker that determines the use of this type of drugs (42,44). In a recently issued draft guidance on ‘Tissue Agnostic Drug Development in Oncology’, the FDA defines the term tissue agnostic drug as a drug that targets a specific molecular alteration across multiple cancer types as defined, for example, by organ, tissue, or tumor type (45).
Table 3
Biomarker | Drug (generic name) |
---|---|
MSI-H or dMMR | Pembrolizumab |
TMB-H | Pembrolizumab |
NTRK1/2/3 | Larotrectinib; entrectinib |
RET | Selpercatinib |
dMMR | Dostarlimab |
BRAF V600E | Dabrafenib in combination with trametinib |
FDA, Food and Drug Administration; MSI-H, microsatellite instability-high; dMMR, deficient mismatch repair; TMB-H, tumor mutational burden-high.
Conclusions and future trends
Modern molecular analytical methods have played a significant role in our understanding of the complexity and heterogeneity of cancer and are important facilitators for the development of new therapeutic options. For a quarter century, CDx assays have played an increasing role in clinical patient stratification by offering objective molecular characteristics for therapy selection, thereby moving away from the “trial and error” approach.
We will continue to see the CDx area being developed in relation to the improvement of the current method, such as digital or computational pathology, in relation to the slide based IHC and ISH assays (46,47). It is expected that the utilization of PCR and NGS based liquid biopsy assays will increase in the future due to the convenience of obtaining a blood sample as compared to performing a biopsy (22,23). Furthermore, new platforms, such as imaging CDx, may also play a more prominent role in the future (48,49).
Despite progress has been made, there are still many unmet medical needs in the treatment of cancer. Although an initial high response rate and improved progression free survival were observed in patients treated with targeted therapy, resistance is frequently observed. We need to further increase our understanding of the complex molecular disease mechanisms, and an avenue to follow could be an integration of data from the genome, transcription, and proteome using specific designed algorithms (50).
The development of CDx assays has thus far been driven mainly by genomic biomarkers, as shown in Figure 2, where more than 70% of the FDA approved assays belong to this category. Genomic data are definitely important but should not be seen in isolation. The human molecular picture is complex, with approximately 22,000 coding genes and with exponentially growing numbers when we look at the number of molecules in the transcriptome and proteome with approximately 100,000 transcripts and 1,000,000 proteins, respectively (51). The National Cancer Institute has recently argued for the integration of proteomics with genomic data, which they named ‘proteogenomics’ (50). They emphasize that the complexity of proteins and their post-translational modifications are often overlooked and not fully captured by genomics. Proteins are the primary target for most pharmacological interventions, and the integration of proteomic data with other types of molecular data through data science may help to better understand the underlying biology and improve treatment options for patients with cancer. It is hoped that such integration will lead to a more complete picture of the relationship between genotype and phenotype and ultimately result in better patient outcomes.
Acknowledgments
Funding: None.
Footnote
Peer Review File: Available at https://cco.amegroups.com/article/view/10.21037/cco-23-96/prf
Conflicts of Interest: The author has completed the ICMJE uniform disclosure form (available at https://cco.amegroups.com/article/view/10.21037/cco-23-96/coif). J.T.J. has worked as a Consultant and Advisor for Agilent Technologies, Alligator Biosciences, Argenx, AstraZeneca, Biovica, Visiopharm and Leo Pharma; received royalties from Elsevier; and furthermore, served as a Board Member of the Dansh Society of Cyto and Histochemistry. The author has no other conflicts of interest to declare.
Ethical Statement: The author is accountable for all aspects of the work in ensuring that questions related to the accuracy or integrity of any part of the work are appropriately investigated and resolved.
Open Access Statement: This is an Open Access article distributed in accordance with the Creative Commons Attribution-NonCommercial-NoDerivs 4.0 International License (CC BY-NC-ND 4.0), which permits the non-commercial replication and distribution of the article with the strict proviso that no changes or edits are made and the original work is properly cited (including links to both the formal publication through the relevant DOI and the license). See: https://creativecommons.org/licenses/by-nc-nd/4.0/.
References
- Spear BB, Heath-Chiozzi M, Huff J. Clinical application of pharmacogenetics. Trends Mol Med 2001;7:201-4. [Crossref] [PubMed]
- Jørgensen JT, Hersom M. Clinical and Regulatory Aspects of Companion Diagnostic Development in Oncology. Clin Pharmacol Ther 2018;103:999-1008. [Crossref] [PubMed]
- Slamon DJ, Clark GM, Wong SG, et al. Human breast cancer: correlation of relapse and survival with amplification of the HER-2/neu oncogene. Science 1987;235:177-82. [Crossref] [PubMed]
- Jørgensen JT, Winther H, Askaa J, et al. A Companion Diagnostic With Significant Clinical Impact in Treatment of Breast and Gastric Cancer. Front Oncol 2021;11:676939. [Crossref] [PubMed]
- Shepard HM, Lewis GD, Sarup JC, et al. Monoclonal antibody therapy of human cancer: taking the HER2 protooncogene to the clinic. J Clin Immunol 1991;11:117-27. [Crossref] [PubMed]
- Carter P, Presta L, Gorman CM, et al. Humanization of an anti-p185HER2 antibody for human cancer therapy. Proc Natl Acad Sci U S A 1992;89:4285-9. [Crossref] [PubMed]
- Pegram MD, Lipton A, Hayes DF, et al. Phase II study of receptor-enhanced chemosensitivity using recombinant humanized anti-p185HER2/neu monoclonal antibody plus cisplatin in patients with HER2/neu-overexpressing metastatic breast cancer refractory to chemotherapy treatment. J Clin Oncol 1998;16:2659-71. [Crossref] [PubMed]
- Cobleigh MA, Vogel CL, Tripathy D, et al. Multinational study of the efficacy and safety of humanized anti-HER2 monoclonal antibody in women who have HER2-overexpressing metastatic breast cancer that has progressed after chemotherapy for metastatic disease. J Clin Oncol 1999;17:2639-48. [Crossref] [PubMed]
- Slamon DJ, Leyland-Jones B, Shak S, et al. Use of chemotherapy plus a monoclonal antibody against HER2 for metastatic breast cancer that overexpresses HER2. N Engl J Med 2001;344:783-92. [Crossref] [PubMed]
- FDA. Full prescribing information for Herceptin (trastuzumab). Initial U.S. Approval: 1998. Revised: 11/2018. Available online: https://www.accessdata.fda.gov/drugsatfda_docs/label/2018/103792s5345lbl.pdf. Accessed September 30, 2023.
- FDA. List of Cleared or Approved Companion Diagnostic Devices (In Vitro and Imaging Tools). Available online: https://www.fda.gov/medical-devices/in-vitro-diagnostics/list-cleared-or-approved-companion-diagnostic-devices-in-vitro-and-imaging-tools. Accessed August 22, 2023.
- FDA-NIH Biomarker Working Group. BEST (Biomarkers, EndpointS, and other Tools) Resource [Internet]. Silver Spring (MD): Food and Drug Administration (US). Predictive Biomarker. Co-published by National Institutes of Health (US), Bethesda (MD), December 22, 2016. Available online: https://www.ncbi.nlm.nih.gov/books/NBK326791/. Accessed October 21, 2023.
- FDA. In Vitro Companion Diagnostic Devices. Guidance for Industry and Food and Drug Administration Staff. August 2014. Available online: https://www.fda.gov/regulatory-information/search-fda-guidance-documents/in-vitro-companion-diagnostic-devices. Accesses August 14, 2023.
- Enzmann H, Benda N, Meyer R, et al. Chapter 17—companion diagnostics and biomarker tests in the European Medicines Agency's Assessment of Medicinal Products. In: Jørgensen JT, editor. Companion and Complementary Diagnostics. Cambridge: Academic Press; 2019:319-33.
- Papadopoulos N, Kinzler KW, Vogelstein B. The role of companion diagnostics in the development and use of mutation-targeted cancer therapies. Nat Biotechnol 2006;24:985-95. [Crossref] [PubMed]
- Langreth R, Waldholz M. New era of personalized medicine: targeting drugs for each unique genetic profile. Oncologist 1999;4:426-7. [Crossref] [PubMed]
- Craig A. Personalised medicine with companion diagnostics: The intercept of medicines and medical devices in the regulatory landscape. EMJ Innov 2017;1:47-53. [Crossref]
- Kang SL, Woo JH, Kim NH, et al. Necessity of strengthening the current clinical regulatory for companion diagnostics: An institutional comparison of the FDA, EMA, and MFDS. Mol Ther Methods Clin Dev 2023;30:447-58. [Crossref] [PubMed]
- European Union. Regulation (EU) 2017/746 of the European Parliament and of the council of 5 April 2017 on in vitro diagnostic medical devices and repealing Directive 98/79/EC and Commission Decision 2010/227/EU. Official Journal of the European Union. April 2017. Available online: https://eur-lex.europa.eu/legal-content/EN/TXT/PDF/?uri=CELEX:32017R0746. Accessed October 21, 2023.
- Verbaanderd C, Trullás Jimeno A, Engelbergs J, et al. Biomarker-Driven Developments in the Context of the New Regulatory Framework for Companion Diagnostics in the European Union. Clin Pharmacol Ther 2023;114:316-24. [Crossref] [PubMed]
- Pignatti F, Ehmann F, Hemmings R, et al. Cancer drug development and the evolving regulatory framework for companion diagnostics in the European union. Clin Cancer Res 2014;20:1458-68. [Crossref] [PubMed]
- Batool SM, Yekula A, Khanna PThe Liquid Biopsy Consortium, et al. Challenges and opportunities for early cancer detection and monitoring. Cell Rep Med 2023;4:101198. [Crossref] [PubMed]
- Sato Y. Clinical utility of liquid biopsy-based companion diagnostics in the non-small-cell lung cancer treatment. Explor Target Antitumor Ther 2022;3:630-42. [Crossref] [PubMed]
- FDA. Premarket Approval for cobas EGFR Mutation Test v2. (04/18/2018). Available online: https://www.accessdata.fda.gov/scripts/cdrh/cfdocs/cfpma/pma.cfm?id=P120019S018. Accessed August 21, 2023.
- FDA. Premarket Approval for Guardant360 CDx. (08/07/2020). Available online: https://www.accessdata.fda.gov/scripts/cdrh/cfdocs/cfpma/pma.cfm?id=p200010. Accessed August 21, 2023.
- FDA. Premarket Approval for FoundationOne Liquid CDx. (08/26/2020). Available online: https://www.accessdata.fda.gov/scripts/cdrh/cfdocs/cfpma/pma.cfm?id=p190032. Accessed August 21, 2023.
- FDA. Drugs@FDA: FDA-approved drugs. Available online: https://www.accessdata.fda.gov/scripts/cder/daf/. Accessed August 22, 2023.
- Jørgensen JT. Oncology drug-companion diagnostic combinations. Cancer Treat Res Commun 2021;29:100492. [Crossref] [PubMed]
- FDA. Guidance for industry. Expedited programs for serious conditions – drugs and biologics. May 2014. Available online: https://www.fda.gov/media/86377/download. Accessed August 22, 2023.
- Beaver JA, Pazdur R. "Dangling" Accelerated Approvals in Oncology. N Engl J Med 2021;384:e68. [Crossref] [PubMed]
- FDA. Full prescribing information Rybrevant (amivantamab). Revised: 05/2021. Available online: https://www.accessdata.fda.gov/drugsatfda_docs/label/2021/761210s000lbl.pdf. Accessed October 1, 2023.
- FDA. Full prescribing information for Tabrecta (capmatinib). Revised: 5/2020. Available online: https://www.accessdata.fda.gov/drugsatfda_docs/label/2020/213591s000lbl.pdf. Accessed October 1, 2023.
- FDA. Full prescribing information for Balversa (erdafitinib). Revised: 04/2019. Available online: https://www.accessdata.fda.gov/drugsatfda_docs/label/2019/212018s000lbl.pdf. Accessed October 1, 2023.
- FDA. Full prescribing information for Rozlytrek (entrectinib). Revised: 8/2019. Available online: https://www.accessdata.fda.gov/drugsatfda_docs/label/2019/212726s000lbl.pdf. Accessed October 1, 2023.
- FDA. Full prescribing information for Vitrakvi (larotrectinib). Revised: 11/2018. Available online: https://www.accessdata.fda.gov/drugsatfda_docs/label/2018/210861s000lbl.pdf. Accessed October 1, 2023.
- Socinski MA, Pennell NA, Davies KD. MET Exon 14 Skipping Mutations in Non-Small-Cell Lung Cancer: An Overview of Biology, Clinical Outcomes, and Testing Considerations. JCO Precis Oncol 2021;5:PO.20.00516.
- Manea CA, Badiu DC, Ploscaru IC, et al. A review of NTRK fusions in cancer. Ann Med Surg (Lond) 2022;79:103893. [Crossref] [PubMed]
- Parker JL, Kuzulugil SS, Pereverzev K, et al. Does biomarker use in oncology improve clinical trial failure risk? A large-scale analysis. Cancer Med 2021;10:1955-63. [Crossref] [PubMed]
- Lara Gongora AB, Carvalho Oliveira LJ, Jardim DL. Impact of the biomarker enrichment strategy in drug development. Expert Rev Mol Diagn 2020;20:611-8. [Crossref] [PubMed]
- Conn CW, Jin J. The value of companion diagnostics in oncology drug development. Expert Rev Mol Diagn 2022;22:591-3. [Crossref] [PubMed]
- Jørgensen JT. Missing Companion Diagnostic for US Food and Drug Administration-Approved Hematological and Oncological Drugs. JCO Precis Oncol 2022;6:e2200100. [Crossref] [PubMed]
- Lemery S, Keegan P, Pazdur R. First FDA Approval Agnostic of Cancer Site - When a Biomarker Defines the Indication. N Engl J Med 2017;377:1409-12. [Crossref] [PubMed]
- Le DT, Uram JN, Wang H, et al. PD-1 Blockade in Tumors with Mismatch-Repair Deficiency. N Engl J Med 2015;372:2509-20. [Crossref] [PubMed]
- Jørgensen JT. Site-agnostic biomarker-guided oncology drug development. Expert Rev Mol Diagn 2020;20:583-92. [Crossref] [PubMed]
- FDA. Draft Guidance for the Industry: Tissue Agnostic Drug Development in Oncology. Food and Drug Administration, October 2022. Available online: https://www.fda.gov/regulatory-information/search-fda-guidance-documents/tissue-agnostic-drug-development-oncology. Accessed August 27, 2023.
- Verghese G, Lennerz JK, Ruta D, et al. Computational pathology in cancer diagnosis, prognosis, and prediction - present day and prospects. J Pathol 2023;260:551-63. [Crossref] [PubMed]
- Li AC, Zhao J, Zhao C, et al. Quantitative digital imaging analysis of HER2 immunohistochemistry predicts the response to anti-HER2 neoadjuvant chemotherapy in HER2-positive breast carcinoma. Breast Cancer Res Treat 2020;180:321-9. [Crossref] [PubMed]
- Tully MK, Sobol NB, Pereira PMR et al. Molecular Imaging Companion Diagnostics. In: Jørgensen JT, editor. Companion and Complementary Diagnostics: From Biomarker Discovery to Clinical Implementation. London: Academic Press; 2019:201-28.
- Liao S, Zhou M, Wang Y, et al. Emerging biomedical imaging-based companion diagnostics for precision medicine. iScience 2023;26:107277. [Crossref] [PubMed]
- Rodriguez H, Zenklusen JC, Staudt LM, et al. The next horizon in precision oncology: Proteogenomics to inform cancer diagnosis and treatment. Cell 2021;184:1661-70. [Crossref] [PubMed]
- National Cancer Institute. Molecular Diagnostics for Cancer Treatment: Completing the Picture. Available online: https://www.cancer.gov/research/annual-plan/scientific-topics/molecular-diagnostics/molecular-diagnostics-for-cancer-treatment-infographic. Accessed August 29, 2023.