Biliary tract cancers: epidemiology, molecular pathogenesis and genetic risk associations
Introduction
Biliary tract cancers (BTC) represent the second most common type of hepatobiliary cancer worldwide, and are typically classified as intrahepatic cholangiocarcinoma (iCCA), perhilar/hilar cholangiocarcinoma (pCCA), distal cholangiocarcinoma (dCCA) and gallbladder cancer (GBC). It is difficult to obtain accurate worldwide incidence estimates for these cancers due to challenges with diagnosis, particularly in low- and middle-income countries, and discrepancies in classification methods worldwide. The most recent estimates from the Global Burden of Disease study are of 139,500 deaths from BTC in 2013, a 22% increase from the estimated 115,400 deaths in 1990, equivalent to age-standardized death rates of 2.3 per 100,000 per year and 3.4 per 100,000 per year, respectively (1). Worldwide, GBC is more common in females, while eCCA has a male predominance (2). In the United States (US) approximately 23,000 cases of BTC are diagnosed annually (3,4). Only 10% of these patients present at an early stage when they would be candidates for surgical resection. The vast majority present with locally advanced or metastatic BTC, for which there are very few therapeutic options (5). The lack of therapeutic options stems in part from our limited understanding of the etiology, risk factors, and pathogenesis of CCA. The risk factors most strongly associated with BTC are those characterized by chronic inflammatory states, such as primary sclerosing cholangitis (PSC), chronic biliary tract infection [i.e., Salmonella typhi (S. typhi), Opisthorchis viverrini (O.viverrini), Clonorchis sinensis (C. sinensis), hepatitis B virus (HBV), and hepatitis C virus (HCV)], asymptomatic stone disease, and diabetes mellitus, among others. Hereditary factors may also play a role, and gene-environment interactions might be important in the pathogenesis of BTC. Thus, BTC, similar to most other cancers, is a complex disease resulting from the combination of familial genetic predisposition and certain environmental factors. Consequently, identifying the genetic variants and modifiers that influence the pathophysiological processes involved in BTC is crucial to understanding the gene-environment interactions important for BTC development. Recent advances using genome-wide association studies (GWAS) have facilitated the identification of multiple replicable common genetic variations associated with cancer, including colorectal cancer, breast, lung, pancreas, melanoma and brain cancers (6,7). Over the last few years, a number of single nucleotide polymorphism (SNP) association studies have identified genetic variants as risk-conferring or protective in the development of BTC. However, the mechanisms by which such SNP variations influence the pathogenesis of BTC are poorly understood. Additionally, some genetic variants are specific to certain populations, which limit the generalizability of recent discoveries. Thus, further epidemiological and functional investigations of these variants are needed to elucidate the etiology and pathologic process of this devastating disease. The results obtained could also potentially lead to the discovery of novel, cancer-specific biomarkers useful for assessing the risk for BTC, the prognosis of BTC patients, and predicting their response to specific treatments (8). In this review we provide an epidemiological overview of the BTCs and discuss the types of results anticipated from GWAS and their potential benefits.
Classification
BTC are highly lethal cancers that comprise a spectrum of carcinomas originating in the bile ducts, the gallbladder or the ampulla of Vater. Cancers of the bile duct are classified into three types based on their location (9). Intrahepatic bile duct cancers develop in the branches of the biliary system located within the liver; those that arise from the ducts outside the liver are referred to as hilar, also called perihilar cholangiocarcinoma (CCA), and dCCAs (10). The latter belong to the extrahepatic subtype of CCAs and are separated by the insertion of the cystic duct (10). Most extrahepatic bile duct tumors arise in the hilar region (60–70%), whereas those arising distally account for about 20–30% of extrahepatic biliary malignancies (11,12). Previously, tumors arising from the gallbladder and from the ampulla of Vater along with these two subtypes of CCA were all classified as extrahepatic biliary cancers. Under the previous classification, iCCA would be designated a primary liver cancer (13). Differences in the methods of classification of BTC can lead to misleading or erroneous epidemiological data (14). Changes in the classification codes over time also confound the interpretation of the available epidemiological data. Thus, epidemiologic studies on the BTC must be interpreted with caution (14). Overall, considering all three types of bile duct cancer, iCCA and pCCA each appear to comprise about 40% of CCA, while dCCA comprise the remaining 20%.
Epidemiology
The global epidemiological trends in incidence of BTC vary according to geographic regions, which in turn relate to the distribution of the risk factors associated with BTC (14). We will consider the epidemiology of GBC and CCA separately.
The incidence rate of GBC is highest in South America, specifically in the Andean region as discussed in further details in this issue by Arroyo et al. (15). Other populations with high rates are North American Indians and Mexican Americans (16). Cancer of the gallbladder is the most common gastrointestinal malignancy among Southwestern Native Americans and Mexican Americans (17). In South America, the main risk factor linked to GBC is symptomatic gallstone disease, particularly in Chile, Bolivia, and Ecuador. Of these countries, Chile has the highest mortality worldwide (18). In Europe, the highest incidence rates are found in Poland, the Czech Republic, and Slovakia (16). India, Pakistan, Korea and Japan are also high-incidence countries (19).
In the US the incidence of GBC is relatively low and actually decreased 0.5% per year between 1999–2011 among women (from 1.5 to 1.38 cases per 100,000) while remaining stable among men (20). Despite the decrease, GBC is still the most common BTC (4). GBC has an up to three times higher incidence in women than in men (16,20,21). The incidence of GBC in adults younger than 45 years of age increased by 2.4% per year from 0.04 to 0.06 cases per 100,000 between 1999 and 2011, while the incidence among patients aged 45 to 84 years remained stable. These data must be interpreted cautiously because the increased incidence in young patients occurred simultaneously with an improvement in diagnostic technologies. Thus, the apparent increased incidence may be related to more accurate diagnostic tools. In those older than 85 years, the incidence decreased 0.9% per year, from 11.74 to 9.93 cases per 100,000 (20).
Among the racial categories, American Indians and Alaskan Natives have higher incidence of GBC than non-Hispanic Whites (20). Relative to Whites, American Indians/Alaska Natives have a racial/ethnic incidence rate ratio (IRR) of 4.5 for women and 5.4 for men, while Hispanics had ratios of 3.1 and 1.8, respectively. Analysis of the temporal trends between 1992 and 2009 demonstrates that the incidence rates among the different races decreased among all except Blacks (21).
Regarding the global epidemiology of CCA, the incidence of iCCA has been increasing in Europe, Asia, Japan, Australia, and North America. In the US, the incidence of iCCA is significantly higher than eCCA, 1.6 vs. 1.3 per 100,000 years, P<0.01, with Asians having the highest incidence for both subtypes, however, this report excluded Alaskan Natives due to small numbers precluding the development of precise incidence estimates (22). Overall, CCA incidence is higher among men than women. In Caucasian populations, this may reflect the higher prevalence of PSC in males (13,18,22). When the incidence of CCA in men is compared among different races in the US, Alaskan Natives have a significantly higher incidence rate (3.4 per 100,000) compared to Whites, (1.6 per 100,000) and Blacks (1.4 per 100,000) (23). Another study compared the incidence rates of eCCA by race/ethnicity between 1992 and 2009 and found that the rates increased throughout the time period for all races and sex groups except for Hispanic males. This study also reported that Alaskan Natives and American Indians had the highest rates among all racial/ethnic groups (21). Secular trends in the incidence of risk factors associated with iCCA, such as HCV, alcoholic liver disease, and cirrhosis, may contribute, at least partially, to the rising trend.
Worldwide, the highest incidence rates of CCA are found in Thailand, which has age-standardized rates of 113 per 100,000 person years in males, and 50 per 100,000 person years in females (14,24). Infection with the liver fluke O. viverrini, which is acquired by eating raw fish, is endemic to northeast Thailand, and is the risk factor most strongly associated with development of CCA. CCA comprises 89% of all primary liver cancers in Thailand (25-27).
Other key factors influencing the risk of BTC are age, gender, race, and presence of comorbidities. In general, the incidence of GBC and CCA increases progressively with age. The reported average age of patients with iCCA is 70 years (9). For those individuals with PSC or choledochal cysts, the average age falls to 30–50 years (13).
Risk factors for biliary tract cancer
The increasing number of BTC worldwide is linked to several important risk factors. Gallstone disease is one of the strongest risk factors for GBC. Even though gallstones are present in 70% to 90% of GBC cases, the overall incidence of GBC in patients with cholelithiasis is 0.5% to 3% (28-32). The exact mechanism by which gallstones predispose to GBC is still unknown, but chronic mucosal damage and constant epithelial irritation may be involved (33). Stones >3 cm carry a 9.2 to 10.1 times greater risk of GBC than of stones <1 cm (33,34). The association of gallstones with CCA is less well established than the association of gallstones with GBC, however, the association of bile duct stones or hepatolithiasis with CCA is quite strong (13). Compared to the West (1–2%), hepatolithiasis is far more prevalent in Southeast Asia, particularly in Taiwan (20% in adults) (35,36). Fifty to seventy percent of patients that undergo resection for CCA in Taiwan have associated hepatolithiasis (13). A Korean study found that patients with hepatolithiasis had a 50-fold increase in the risk of CCA (37).
PSC, which is characterized by cholestasis with chronic inflammation of the biliary duct that ultimately results in fibrosis and stricturing of the bile ducts, is also a risk factor for CCA (38). The annual incidence of CCA in patients with PSC has been estimated to be between 0.5–1.5%, with a lifetime risk ranging from 5% to 15% (39-44). The increased prevalence of PSC may be due either to increased awareness of the disease or to as yet unidentified environmental factors (45,46). PSC patients develop CCA earlier than those without, typically in their 40s as opposed to 70s, and the incidence is higher in men (45,47-49). Thirty to fifty percent of CCA cases occurring in persons with PSC are diagnosed at the time of PSC diagnosis (44,48,50). The diagnosis of CCA in patients with PSC is extremely challenging because the inflammation-related changes in PSC may mimic CCA (47,51). Numerous genetic association studies have examined genetic variants associated with PSC, and a strong association has been found with HLA complex polymorphisms, with the most robust findings localizing to chromosome 6p21 (52,53). Other susceptibility loci have been identified in chromosomes 2q35, 3p21 and 13q31 (53). A recent genetic study identified four new genome-wide significant susceptibility loci for PSC: rs7556897, rs17032705, rs12369214, rs11649613 (54).
The association of diabetes with BTC is difficult to assess due to the close associations of diabetes with obesity and gallstones, but many studies support the concept that obesity increases the risk of BTC (55-59). As BMI increases, the risk of BTC, especially iCCA, increases as well (56,60,61). A meta-analysis of 21 studies showed an increased risk of BTC in persons with diabetes compared to non-diabetics [relative risk (RR): 1.4; 95% CI: 1.2–1.7] (62). Another meta-analysis, assessed the risk by subtype: the RR for all CCA was 1.6 (95% CI: 1.6–2.5), for eCCA 1.6 (95% CI: 1.3–2.1), and for iCCA 2.0 (95% CI: 1.6–2.5) (63). Yet another meta-analysis focused on the risk of eCCA reported very similar risk [odds ratio (OR): 1.6, 95% CI: 1.1–2.5] (64). In a case-control study from Shanghai, the association of diabetes and GBC was strong and independent of obesity (OR: 2.6; 95% CI: 1.5–4.7), but the effect was mediated in part by biliary stones and in part by low serum HDL (57). Metformin, an oral anti-hyperglycemic agent of the biguanide family, may function as a protective agent against cancer in diabetic patients (65,66). A recent study concluded that metformin use significantly reduced the risk of iCCA in diabetic patients by 60% (67). Metformin inhibits the growth of iCCA cells by activating the AMPK/mTOR complex 1 (mTORC1) pathway, which in turn activates the p53 protein, inducing cell cycle arrest and preventing tumor cell proliferation (68).
Patients with choledochal cysts and Caroli’s disease, a rare congenital fibropolycystic liver disease characterized by cystic dilations of the large intrahepatic bile ducts, appear to be at risk for iCCA (69,70). The overall lifetime incidence of CCA in persons with choledochal cysts is 10%, while an incidence as high as 28% has been reported in those that do not receive treatment (71-73). Patients with Caroli’s disease have a 100-fold increase in risk of CCA when compared to the general population (71). Up to 90% of cases of congenital choledochal cysts present with an abnormal pancreaticobiliary junction (APBJ), which is a rare anatomic variation in which the pancreatic duct drains into the common bile duct (18,74). Prolonged exposure of the biliary epithelium to the refluxed pancreatic juice promotes carcinogenesis (75). Even though bile duct cancer is observed, GBC is much more common in patients with APBJ (18,75).
Infectious diseases, including parasites, bacteria and viruses, cause inflammation of the biliary tract, and a number of these diseases have been linked to the development of biliary tract malignancies. A strong association exists between liver fluke infestation and the development of biliary tract malignancies, notable by the high incidence of CCA in areas in Asia and Eastern Europe where liver fluke infestation is prevalent (76-80). Endemic areas for C. sinensis infestation include eastern Russia and Manchuria, South Korea, mainland China (except the northwest), Taiwan, and northern Vietnam, while O. viverrini is endemic in Laos and Northeast Thailand (80). In particular, northeast Thailand has very high overall CCA incidence rates (85/100,000 population) (78). The traditional consumption of raw or undercooked wild-caught cyprinoid fish and fish-based dishes causes infestation of humans with the adult forms of liver flukes, most commonly O. viverrini and C. sinenesis (81-83). The liver flukes occupy the gallbladder and biliary tree of the human host, causing desquamation of the biliary epithelium, inducing chronic inflammation with development of adenomatous hyperplasia and periductal fibrosis, and eventually promoting the malignant transformation of the biliary epithelium (84-86). The secretion of parasite proteins with mitogenic properties into the bile creates a tumorigenic environment that may drive this transformation (83). Potential cofactors in the carcinogenic process include carcinogens such as nitrosamines produced by bacteria in fermented fish and other foods, smoking, alcohol, and HBV infection (13).
Chronic infection with HBV and HCV also contribute to the unique geographical distribution of BTC. The prevalence of viral infection is higher in low- and middle-income Eastern countries, particularly in Southeast Asia (HBV 9.1%; HCV 3.6%), China (HBV 12%; HCV 3%), and Korea (HBV 12%; HCV 2%), with relatively low rates in Western countries (87,88). A cohort study found that HCV conferred more than two-fold elevated risk of iCCA (HR: 2.6; 95% CI: 1.3–5.0), while the risk of eCCA, on the other hand, was not significantly increased (HR: 1.5; 95% CI: 0.6–1.9) (89). Another study analyzed the risk factors of patients seen at the Mayo Clinic in Rochester, MN, and found that HCV infection is associated with an OR of 6.4 for iCCA (95% CI: 1.4–28.5; P<0.001) (67).
Regarding the association of iCCA with HBV infection, a meta-analysis found that persons with HBV infection had an increased risk of iCCA (RR: 3.4; 95% CI: 2.5–43.7) compared to those without HBV (90). Another meta-analysis that included studies performed in regions of both high and low prevalence of hepatobiliary cancers, concluded that the presence of HBV was associated with a combined OR of 5.5 (95% CI: 3.2–9.6) for iCCA (91). The association between HBV and CCA is stronger in Asian countries (OR: 6.0) than in Western countries (OR: 4.0) (92,93). Furthermore, the presence of cirrhosis increases the risk of iCCA even more, by 2.5-fold (95% CI: 1.2–5.1; P=0.02) in HBV, and 3.2-fold (95% CI: 1.231–8.148, P=0.017) in HCV patients (94). Co-infection with HBV and HCV with concomitant cirrhosis increased the risk of iCCA by 12.6-fold (95% CI: 2.5–62.9; P=0.002) (94). A case-control study of patients with and without CCA found nonspecific cirrhosis to be significantly more prevalent among cases (OR, 27.2; P<0.0001) (58). In addition, a meta-analysis of persons with unspecified liver cirrhosis found that the overall OR for iCCA was 22.9 (95% CI: 18.2–28.8) (58,92). In a separate study, cirrhosis was associated with an increased OR of 8.0 (95% CI: 1.8–36.5; P<0.007) for iCCA (67). Thus, there is a clear and strong association between viral hepatitis and CCA.
Bacterial infection occurs through bacterial invasion of the mucosal surface of the intestine, with spread into organs such as the liver, spleen, and bone marrow after phagocytosis by macrophages (95). Chronic infection with S. typhi is one such example (96,97). S. typhi is a gram-negative serovar of the Salmonella enterica subspecies that spreads to the gallbladder via the vasculature or through the bile ducts from the liver during enterohepatic circulation (98,99). The primary reservoirs for S. typhi are chronic carriers who shed bacteria through their feces and urine (100-102). Annually, this bacterium causes 21 million newly diagnosed cases of typhoid fever and about 200,000 deaths (95,103). In areas endemic for S. typhi approximately <5% of all individuals acutely infected will become chronic asymptomatic carriers (104,105). Although individuals with chronic S. typhi infections are contagious with infection persisting for decades, it is difficult to identify chronic carriers due to its asymptomatic nature (106,107). Several studies have shown that chronic carriers of S. typhi have an increased risk of GBC (108-110). Chronic carriage of S. typhi is twice as common in women compared to men (111).
Helicobacters, spiral gram-negative bacteria, have also been identified as potential infectious carcinogens. Various species of helicobacter have been identified in the bile, gallbladder and liver tissue of patients with hepatobiliary diseases (112-114). Helicobacter pylori (H. pylori) colonize the gastric epithelium, living in the mucus layer that coats the internal lining of the stomach. There have been associations between BTC and H. pylori; however, a direct cause-and-effect relationship has not been established (115-118). Some studies have suggested that H. pylori are involved in the development of biliary neoplasms through enhancement of inflammation and proliferation of biliary cells. Most studies have had small sample sizes of CCA patients, and this relationship is still controversial (119).
Helicobacter bilis (H. bilis) is an opportunistic helicobacter associated with chronic liver disease, BTC and GBC, and chronic diarrhea (120-123). The isolation of Helicobacter species from the biliary system has stimulated interest in the role of these species in carcinogenesis. A meta-analysis found the infection rate of Helicobacter species to be higher in persons with biliary tract cancer compared to unaffected individuals, but did not reach statistical significance. H. pylori, H. hepaticus, and H. bilis but not H. ganmani, were significantly more frequent in the malignant group than in the benign biliary disease group (124). Mouse studies have linked H. bilis to the pathogenesis of chronic hepatitis and hepatocellular carcinoma; further, H. bilis has been implicated in cholesterol gallstone formation (125-128). Recent studies have shown that H. bilis infections activate NF-кB signaling, thus increasing the cellular expression of pro-angiogenic VEGF in BTC (129,130). However, a direct link between H. bilis and BTC has not been confirmed (131).
A variety of biological and chemical toxins have also been implicated in biliary tract carcinogenesis. Aflatoxin is a mycotoxin produced by Aspergillus fungi, mainly Aspergillus flavus and Aspergillus parasiticus, which are abundant in warm, humid areas (132). Aflatoxins are naturally occurring food contaminants and can be found in a wide range of produce including cereals, oilseeds, nuts, spices, milk, and meat (133). The contamination of Chilean red chili peppers from Santiago with aflatoxins was confirmed in a study aimed at identifying mutagens present in this produce, but the aflatoxin concentrations were relatively low (62,134). Aflatoxins were first recognized as carcinogenic in 1976, but their role in gallbladder carcinogenesis had not been assessed until recently (133,135). The proposed mechanism is exposure of the gallbladder to the carcinogenic metabolites of aflatoxin when these are stored for excretion in the bile (134). A case-control study found significantly more circulating aflatoxin-albumin adducts in patients with GBC compared to population controls (OR: 13.0; 95% CI: 3.0–52.5) (136).
The concentrations of aflatoxins B1, B2, G1, and G2, were found to be low in some preparations of red chili peppers from Chile (Santiago), Peru (Trujillo, Cusco and Lima) and Bolivia (La Paz) (137). Therefore, other mycotoxins, such as ochratoxin A (OTA), may also be associated with GBC development (137). OTA is produced by Penicillium and Aspergillus species and, similar to aflatoxins, is found in spices, cereals, and nuts as well as in cocoa, beer, and coffee (137,138). With the objective of assessing the association between the mycotoxins (both aflatoxin and ochratoxin) and the incidence of GBC, the authors measured the concentration of the mycotoxins in dried red chili peppers from these countries. They found that red chili peppers from Peru have higher levels of OTA than aflatoxins. Furthermore, since Chile and Bolivia have a higher GBC incidence than Peru and the mean OTA concentrations in the dried red chili peppers from these two countries were higher than in peppers from Peru, the authors suggest a stronger association between OTA contamination of red chili peppers and the development of GBC (16,137).
The recently established link between patients diagnosed with CCA at a young age and their employment in proof-printing plants has led the Japanese Ministry of Health, Labour and Welfare to categorize CCA as an occupational disease (139). Seventeen workers from a proof-printing plant in Osaka, Japan, were diagnosed with iCCA between November 1996 and November 2012. By February 2014, 83 patients had filed claims for workers compensation. Chronic exposure to organic solvents used in printing, such as 1,2-dicholoropropane (1,2-DCP) and dichloromethane (DCM), has been implicated as a causative factor (139). Studies have confirmed the exposure of some of these workers to very high levels of 1,2-DCP for prolonged periods of time (139-141). These patients were diagnosed between the ages of 25 to 45 years, were all exposed to organic solvents and presented with regional dilation of the bile ducts, high serum γ-glutamyl transpeptidase activity, and lesions arising from the large intrahepatic bile ducts (142). Similar results were found in another cohort of nine CCA patients from seven printing companies in Japan (143). For this reason, it is important to assess occupational history when evaluating a patient with suspected CCA (144).
Survival of patients with BTC
BTCs are highly lethal cancers. Due to substantial variation in the availability of complex medical care across the countries and regions with high prevalence of BTC, there is significant regional variation in patient outcomes. However, even in the most developed settings, BTCs are among the most aggressive, therapy-resistant, and recurrent of all cancers.
Data from the National Cancer Data Base of the American College of Surgeons report that the 5-year survival rate for patients with stage 0 GBC as 80%, for stage I 50% stage II 28% stage IIA 8%, stage IIB 7%, stage IVA 4%, and stage IVB 2% (145). Data from the US Surveillance Epidemiology and End Results (SEER) cancer registry on 5-year survival rates of CCA are also reported according to the stage of the disease. The 5-year overall survival rates for patients with localized, regional and distant metastatic iCCA are 15%, 6% and 2%, respectively. For the extrahepatic subtype, the rates are 30% for patients with localized disease, 24% for regional, and 2% for distant disease (9). A study performed in Thailand reported an overall 5-year survival rate for pCCA of 20.6% (95% CI: 13.8–28.4), with a median overall survival of 19.9 months (146).
Regarding patients receiving surgical therapy, a meta-analysis of 4,756 iCCA patients receiving surgical resection found that the 5-year overall survival following surgical management was 30% (range, 5–56%), with a median overall survival of approximately 28 months (range, 9–53 months) (147). Tumor recurrence was common following surgical resection, with 61% to 98% of patients experiencing recurrence 5 years after surgery (147). The median overall survival of 56 patients with dCCA who underwent pancreaticoduodenectomy was 36.9 months, and recurrence occurred in 67% (148). A Chinese study of 814 patients with pCCA found that patients who had surgery with curative intent had a median overall survival of 26.3 months (149).
The survival rates of patients with BTC vary with their underlying biliary or hepatic disease. For example, PSC patients with CCA who undergo surgical resection with negative tumor margins have a 3-year survival rate of <20% (44,48,50). A recent meta-analysis concluded that patients with iCCA and HBV infection had better overall and disease-free survival with a hazard ratio (HR) of 0.76 (95% CI: 0.70–0.83) and 0.78 (95% CI: 0.66–0.94), respectively. HCV infection, on the other hand, was associated with shortened overall survival, with a HR of 2.64 (95% CI: 1.77–3.93) (150).
Molecular pathogenesis
The molecular pathogenesis of BTC is poorly understood Figure 1 (151). Thus far, two key pathogenic mechanisms have been identified in GBC (16,18,32). The main mechanism is through cholelithiasis, which leads to chronic cholecystitis and subsequent oncogenesis. GBC is strongly associated with race, female gender, age older than 65, and past medical history of symptomatic gallstone disease (16,18,32). Another mechanism implicated in GBC pathogenesis is the presence of an APBJ. GBC arising in the presence of an APBJ tends to occur in younger patients and have a lower incidence of associated cholelithiasis (16,18,32). GBC cases that develop in the context of an APBJ consistently demonstrate KRAS mutations, which activate inappropriate growth signals, while those with cholelithiasis rarely have KRAS mutations (5,32). Both pathogenic mechanisms induce mutations in the p53 gene; however, the effects on the p53 pathway are induced during different stages of oncogenesis. Early-onset p53 mutations are characteristic of cholelithiasis-induced GBC, while late onset mutations are more common in APBJ-associated cases (18). In general, the frequency of p53 mutations in GBC ranges from 35% to 92%, with most studies showing a frequency >50% (16,32).
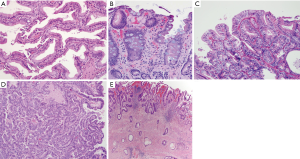
The progressive accumulation of oncogenic aberrations in the biliary epithelium induce malignant transformation (16). A definitive, stepwise model of the cellular and molecular events during malignant biliary transformation has not been established; yet current evidence supports the sequence of intestinal metaplasia to dysplasia, followed by carcinoma in situ, and finally, invasive carcinoma (5). The fact that about 60% of GBC have intestinal metaplasia and more than 90% have dysplasia in the adjacent mucosa supports this hypothesis (18,152,153). The spectrum and temporal sequence of mutations occurring in the intestinal metaplasia to dysplasia to GBC sequence differ from the sequence seen in the adenoma to dysplasia to carcinoma sequence, such as is typical of colorectal cancer. Gallbladder adenomas appear distinct from non-adenomatous intestinal metaplasia/dysplasia, with a substantially lower propensity for transformation into GBC. Mutations in the CTNNB1 gene are found at a high frequency in gallbladder adenomas, while they are virtually absent in metaplastic/dysplastic lesions, and rarely observed in GBC (154-156). In contrast, dysplastic lesions associated with progression to GBC, particularly those associated with an APBJ, frequently demonstrate KRAS mutations. Thus, there appears to be an inverse relationship between CTNNB1 and KRAS mutations observed in adenomas compared to pre-cancerous metaplastic/dysplastic lesions. The reciprocity of these molecular defects suggests that GBC preceded by metaplastic/dysplastic lesions is a different biological entity than those that develop from adenomas, with the former having a higher malignant potential (13). This concept is further supported by the fact that metaplasia/dysplasia is frequently found in association with GBC, while adenoma is a rare finding (5).
The pathogenic mechanisms leading to development of CCA are also unclear. There is a general consensus that the malignant transformation of the biliary epithelium is mediated through a chronic inflammatory state induced by the release of pro-inflammatory mediators. The resulting biliary damage generates cholestasis, which causes aberrant bile acid signaling. The subsequent activation of growth factors promotes cholangiocyte proliferation. These changes, occurring in an inflammatory milieu that promotes the accumulation of additional genetic and epigenetic alterations, lead to uncontrolled proliferation, survival, angiogenesis, invasion and metastasis (157,158). Recent evidence that aspirin use reduces the risk of all subtypes of CCA is consistent with this hypothesis (159).
Two key premalignant precursor lesions have been defined during the development of CCA: biliary intraepithelial neoplasia (BillN) and intraductal papillary neoplasm of bile ducts (IPNB) Figure 2 (5,160). Figure 3 grossly demonstrates an IPNB tumor in a dilated duct. Figure 4 displays the papillary projections in low power magnification. The molecular profiles of these lesions have not yet been completely defined, although mutations in p53 and CDKN2A have been described and loss of SMAD4 has also been shown by immunohistochemistry (5,160,161). CDKN2A encodes the cyclin-dependent kinase (CDK) inhibitor INK4, also known as p16, which inhibits CDK-4 and -6. Active CDK-4 and -6 form complexes with cyclin D1 to phosphorylate and inactivate the retinoblastoma (Rb) tumor suppressor protein, thus inducing progression of the cell cycle from the G1 to S phase (32,160). Consequently, inactivation of the p16 cell cycle inhibitor leads to checkpoint abrogation and abnormal progression through the cell cycle. Immunohistochemistry of IPMN lesions demonstrated cyclin D1 positivity in 65% of samples, less so in the BillN lineage (162). Although oncogenic molecular aberrations are observed in all CCA subtypes, the specific aberrant genes vary between iCCA, pCCA and dCCA and the genetic alterations reported thus far also vary in studies performed in different populations, suggesting population and/or etiologic variations in the carcinogenic mechanisms (163). We will now discuss the alterations most commonly found in the different CCA subtypes.
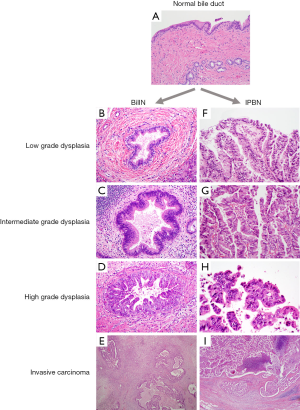
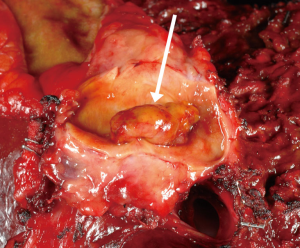
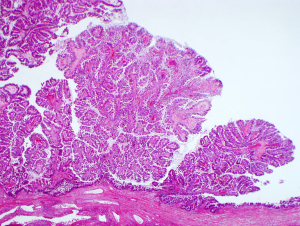
The most frequently identified mutations in iCCA include TP53, KRAS/NRAS, and IDH1/2, whereas PIK3CA mutations are rarely observed (164-170). KRAS and TP53 mutation and loss of PTEN by mutation or epigenetic silencing have been associated with worse survival in patients with iCCA (171,172). Whole exome sequencing has identified mutations in the chromatin remodeling genes BAP1, ARID1A, and PBRM1 and these appear more frequently in iCCA than eCCA (164,165,173). FGFR2 fusions and IDH1/2 mutations also are much more prevalent in the intrahepatic subtype, since they are rarely found in eCCA or HCC (174).
Two molecular subclasses of iCCA have been described in two independent studies: the proliferation molecular subclass or the poor prognosis subclass and the inflammation subclass or good prognosis subclass (175,176). The former is characterized by activation of oncogenic receptor tyrosine kinase signaling pathways, including MET, EGFR, HER2, ERBB3 and RAS-MAPK. The second subclass is distinguished by the activation of cytokine-related pathways and constitutive activation of STAT3 (174).
The mutational profiles of the eCCA subtypes have been studied less extensively than the intrahepatic subtype. Although KRAS and TP53 mutations are relatively common in all CCA, KRAS and TP53 mutations are notably more frequent in eCCA than iCCA (174,177,178). Genetic alterations in the chromatin remodeling genes BAP1 and PRBM1 have been associated with bone metastasis and worse survival in patients with eCCA (173).
Genetic association studies in biliary tract cancer
Over the past three decades, technological advances have sparked substantial interest in the genetic basis of cancer. Cancers are complex diseases resulting from the combination of genetic, environmental, and lifestyle factors; yet, in BTC, the contributions of each of these factors to carcinogenesis and tumor progression are still poorly understood (179). In order to elucidate the inherited genetic variants related to the development of BTC, scientists have conducted genetic association studies that, analogous to traditional epidemiologic association studies, seek association between a risk variable, in this case genetic variants, and a disease outcome, BTC (180).
There are two primary types of genetic association studies: candidate gene and genome wide. In the genome-wide approach, the entire genome of numerous patients with the disease of interest and disease-free controls are screened simultaneously for a large number of known genetic variants present in the genome (180,181). The most common genetic variant and the one most frequently studied is the SNP. SNPs of interest are identified by comparing the distribution of particular variants in cases and controls, under the premise of “common disease-common variant” (7). This premise assumes that common variants in many genes will each lead to a small rise, or fall, in the risk of disease and the sum of each, plus the effect of environmental exposures, account for the overall risk of disease (180). Only those SNPs reaching a certain threshold, that is, for which there is a statistically significant difference in the frequency between cases and control, are considered to be associated with the disease of interest. The associations between SNPs and cases do not necessarily imply that nearby genes are drivers of the disease, however, they may point to key mechanisms involved in carcinogenesis (52).
In the alternate approach of candidate gene studies, a variant is selected based on its hypothesized biological role in the disease and is genotyped in a case-control study (180). This type of study searches for a statistical correlation between the specific genetic variant(s) and the disease. Because these studies are based on the ability to predict functional candidate genes and variants, this approach has been subject to the criticism that current knowledge is insufficient to make accurate and reliable predictions of causative risk variants (182).
With the exception of one Japanese GWAS, which found that the SNP rs7504990 in the deleted in colon cancer (DCC) gene is associated with an increased risk of GBC in a small sample (OR: 7.0; 95% CI: 3.4–14.1, P=7.46×10−8), all reported genetic association studies on BTC thus far have used the candidate gene approach Figure 5 (183). Tables 1-3 summarize the genetic variants that have been associated with GBC, CCA, and BTC. In this section we will discuss the genetic variants most frequently studied in the context of BTC.
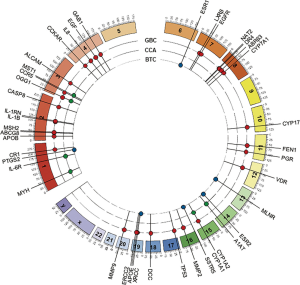
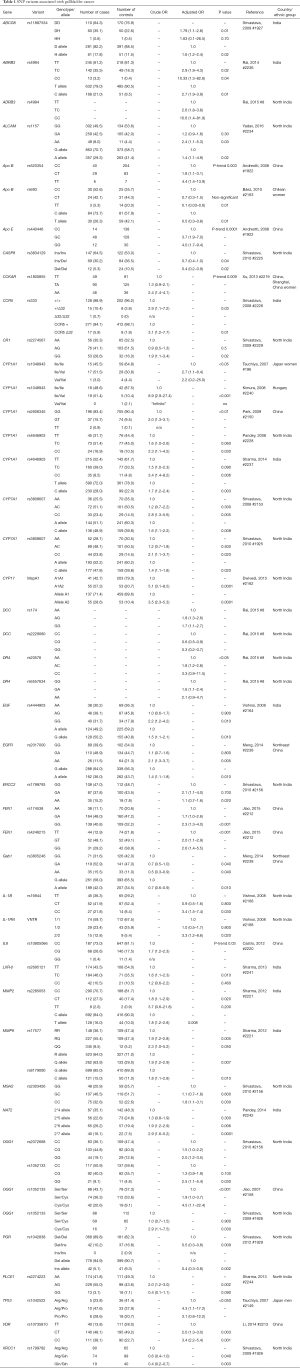
Full table
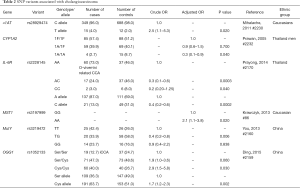
Full table
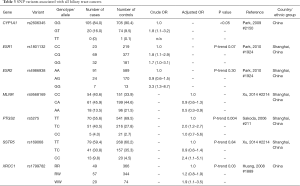
Full table
The cytochrome P450 enzymes play a role in the synthesis of steroid hormones, bile acids, and certain fats, as well as in the metabolism of medications and toxins (184). Aryl-hydrocarbon hydroxylase, a phase I enzyme encoded by the CYP1A1 gene, forms part of the xenobiotic-metabolizing machinery, which is responsible for metabolizing exogenous compounds such as drugs, tobacco and agricultural chemicals (185). Furthermore, this enzyme assists in metabolizing polycyclic aromatic hydrocarbons, nitrosamines, and aromatic amines to carcinogenic intermediates (186). The altered metabolism of xenobiotics may contribute to susceptibility to GBC.
The CC genotype resulting from the transition from T to C in position 6235 of the CYP1A1 gene (rs4646903, also known as the CYP1A1 Msp1 polymorphism) is associated with a 2.3-fold increase in the risk of GBC (95% CI: 1.1–4.5, P=0.026) compared to the TT genotype (187). The C allele of this polymorphism has also been linked to smoking-related cancers (188). Another polymorphism in the CYP1A1 gene, rs1048943, with a transition from A to G at position 1506 of the mRNA, results in the substitution of isoleucine by valine at position 462 of the protein. Compared to those with the Ile/Ile genotype, patients with the Ile/Val genotype are 2.7 times more likely to develop GBC (95% CI: 1.1–6.4, P<0.05). Furthermore, carriers of the T allele of rs2606345 of CYP1A1 had an OR of 2.0 for GBC (95% CI: 1.3–3.0, P<0.01) (189). Regarding the rs4646903 polymorphism, the T allele of IVS1 + 606 was associated with twice the risk of GBC compared to the G allele (OR: 2.0; 95% CI: 1.3–3.0) (190).
Since there is a female predominance of GBC, endogenous estrogens and their metabolites may play an etiologic role in the development of this malignancy. Cytochrome P450C17a mediates steroid 17a-hydroxylase and 17,20-lyase activity and is, therefore, a key enzyme in estrogen metabolism (191). The rs743572 polymorphism at nucleotide 27 produces a transition from thymidine to cytosine. The T allele has been represented as the A1 allele, and the C allele has been denominated A2. A2 allele carriers may have higher levels of estrogens because the nucleotide substitution results in an additional Sp1-binding site with enhanced promoter activity and increased transcription rates. Subsequent studies demonstrated no difference in the promoter activity and mRNA expression between the two alleles (192). A case-control study from China found that carriers of the A1 allele of the CYP17 MspA1 polymorphism had an increased risk of GBC compared to those with the A2A2 genotype (OR: 1.5; 95% CI: 1.1–2.1) (193). A study from North India performed on considerably fewer subjects found the risk of GBC was 3.5 times greater in carriers of the A2 allele (95% CI: 2.3–5.3; P=0.0001) (192). The discrepancies between these two studies, both performed on the same polymorphism in different populations, attest to the fact that the frequency of variant alleles and their effects on phenotypes vary substantially by racial group. Therefore, it is imperative to reproduce association studies in different racial or ethnic groups (194,195).
Super-saturation of bile with cholesterol has been implicated in gallstone formation (196). Conversion of cholesterol into bile acids is a major pathway for eliminating cholesterol from the body (197). Because bile acids play a major role in cholesterol homeostasis, polymorphisms in the CYP7A1 gene, which encodes for the first and rate-limiting step in the classical bile acid synthesis pathway, may influence susceptibility to GBC. The CYP7A1 gene is located in chromosome 8q11-q12, and a SNP conferring an A>C transition is present at the 204 position of the gene. Subjects with the CC genotype are 2.8 times more likely to develop GBC than those with the AA genotype (95% CI: 1.3–5.6, P=0.005) (198). The mechanism by which GBC develops appears to be mediated by accumulation of toxic substances in the gallbladder, rather than gallstone formation. However, this must be confirmed with additional studies and in different populations (198).
The apolipoproteins are another class of key mediators of cholesterol homeostasis. Apolipoproteins are major components of lipoproteins and play a critical role in the transport of cholesterol to the liver. The APO E and APO B genes are located on chromosomes19q13.2 and 2p24-p23, respectively, and encode apolipoproteins, which are major carriers and binding proteins for low-density lipoproteins (LDL). Genetic polymorphisms in the apolipoprotein genes have been associated with gallstones and BTC, and some polymorphisms have been linked to higher serum levels of cholesterol and LDL and lower levels of HDL. Male carriers of the G allele of APOE IVS1 + 69C>G appear to have a 3.7-fold increased risk of bile duct cancer (95% CI: 2.0–7.0) (199). The same sex-specificity was found for the T allele of IVS6 + 360C>T (OR 2.0; 95% CI: 1.2–3.4) (199). The increased risk conferred by these variants was independent of the presence of gallstones. Rs693 is another polymorphism of the APO B gene specifically associated with GBC and is protective for carriers of the T allele (OR: 0.5; 95% CI: 0.3–0.8; P=0.01) (200). The TT genotype was demonstrated to be protective of GBC compared to CC (OR: 0.1; 95% CI: 0.03–0.6; P=0.01) (200).
It is well known that impairment in DNA repair processes leads to cancer. Polymorphisms of DNA repair genes may affect the host’s capacity to repair damaged DNA, leading to the accumulation of mutations and an increased risk of cancer. The 8-oxoguanine glycosylase 1 (OGG1) gene localized at chromosome 3p25 encodes a protein that initiates the base excision repair (BER) pathway and is responsible for the elimination of 8-oxoG, a byproduct of the attack of reactive oxygen species on DNA. A rs1052133 polymorphism GG (Cys/Cys) variant at position 1245 (Ser326Cys) reduces the capacity of OGG1 to repair oxidative DNA damage during conditions of intracellular oxidative stress. Cellular accumulation of Cys326-OGG1 protein under conditions of intracellular oxidative stress appears to contribute to the defect in DNA repair (201). The GG variant was associated with an OR of 2.9 (95% CI: 1.1–7.5; P=0.025) for development of GBC versus the CC (Ser/Ser) variant (202). Similarly, in a population from northern India, the GG genotype was associated with an OR of 2.5 (95% CI: 1.1–5.4, P=0.03) compared to the CC genotype (203). A Chinese population study demonstrated that individuals carrying the GG genotype had a higher risk of iCCA (OR: 2.9; 95% CI: 1.5–5.8) compared to individuals bearing the wild-type CC genotype (204). Another study conducted in China found that the heterozygous CG (Ser/Cys) genotype had an OR of 4.5 vs. CC (Ser/Ser) (95% CI: 1.1–22.4) (205). These studies have consistently shown that OGG1 is associated with BTC.
ERCC2 is also involved in the repair of damaged DNA, and a polymorphism in rs1799793 has been linked to GBC risk. Compared to the homozygous wild-type GG genotype, the AA genotype (Asp312Asn) was 2.1 more frequent in GBC cases than in controls (95% CI: 1.1–4.0; P=0.02) (203). The same study found an OR of 1.8 (95% CI: 1.1–3.1; P=0.03) for the IVS1 + 9G>C polymorphism in rs2303426 of MSH2 when comparing the CC genotype to the wild-type GG genotype.
The X-ray repair cross-complementing group 1 gene (XRCC1) at chromosome 19q13.2 is involved in the single-strand break repair and BER pathways. Several variants of this gene have been implicated in the increased risk of bile duct cancer, including a substitution of arginine for tryptophan at position 194 (R194W), which was associated with an increased risk of bile duct cancer (OR: 1.9; 95% CI: 1.1–3.5, P=0.03), and a substitution of arginine for histidine (R280H) variant, which was associated with a reduced risk of bile duct cancer (166). Interestingly, the 399Gln allele of the Arg399Gln polymorphism conferred a significantly reduced risk of GBC but not bile duct cancer (OR: 0.37; 95% CI: 0.2–0.7, P=0.003) (202).
The MutY homolog is another key enzyme in the BER pathway. Mutations in this gene result in predisposition to colorectal and stomach cancer and its role in CCA tumorigenesis has not been established. One study found the TG genotype in the rs3219476 polymorphism to be associated with a decreased risk of CCA compared to the TT genotype (OR: 0.4; 95% CI: 0.2–0.8; P=0.006) (206).
Growth factors play important roles in carcinogenesis by promoting cell proliferation, inhibiting apoptosis, and enhancing invasion, metastasis, and angiogenesis. Polymorphisms of vascular endothelial factor (VEGF), epidermal growth factor (EGF) and transforming growth factor β 1 (TGFβ1) have been studied in the context of GBC. Results from a case-control study in India demonstrated that subjects with GG genotype at rs4444903 in the EGF gene are 2.2 times more likely to have GBC than those with the AA genotype (95% CI: 1.2–4.2; P=0.012) (207). The presence of the T allele in rs3025039 of the VEGF gene was associated with an OR of 0.7 compared to the CC genotype (95% CI: 0.5–1.0) (208). The G allele of rs1570360 (g.43737830A>G), also located within the VEGF gene region, was associated with a decreased risk of GBC (OR: 0.7; 95% CI: 0.5–0.9; P=0.008) (209). On the contrary, the T allele of rs112005313 (c.237C>T) is linked to an increased risk of GBC compared to those that carry the C allele (OR: 1.6; 95% CI: 1.1–2.4; P=0.02) (209).
A Japanese study found that DCC rs714 was associated with increased susceptibility to GBC. Another study assessed this association in a population in Northern India and failed to replicate the results (183,210).
The strongest risk factors for CCA are related to chronic inflammation, which is characterized by up-regulation of the inflammation-related genes, cyclooxygenase (COX-2), also known as prostaglandin-endoperoxidase synthase 2 (PTGS-2), and the cytokine interleukin-6 (IL-6) (211). Polymorphic variants in the PTGS-2 gene located at 1q25.2-25.3 on chromosome 1 may affect cancer susceptibility. The C allele in Ex10 + 83T>C of the PTGS-2 gene is associated with a 1.8-fold increased risk of bile duct cancer when compared to the T allele (95% CI: 1.2–2.7) (212). The SNPs rs2143417 and rs689466 in the PTGS-2 gene are significantly associated with increased risk for CCA (OR: 1.4; 95% CI: 1.1–1.7; P=0.005 and OR: 1.5; 95% CI: 1.2–1.9; P=0.0003, respectively). To emphasize the high likelihood of false-positive results and the importance of validation of candidate gene SNP studies, of all the candidate gene studies discussed in this review, this study was the only one that conducted a replication study in a validation cohort but failed to replicate the original results found in the test cohort (211).
Since chronic inflammation predisposes to malignancy, cytokines that mediate inflammatory immune responses could be involved in the pathogenesis of such malignancies. IL-6 is a multifunctional cytokine that plays an important role in a wide range of biologic activities, mostly mediated by binding to the IL-6 receptor (IL-6R) (213). Association analyses identified that rs8192284 in exon 9 of IL-6R is associated with Opistorchiasis-related CCA in the Thai population. This polymorphism causes non-synonymous substitution from asparagine to alanine in position 358 (D358A). Allele C protects against CCA when compared to allele A (OR: 0.4; 95% CI: 0.2–0.6, P=0.0002) (214). Rs8192284 has also been linked to inflammatory diseases including diabetes, obesity, arthritis, and periodontitis and reportedly plays a pivotal role in the pathogenesis of pancreatic, gastric, and renal cell carcinoma (214).
The rs10805066 polymorphism of IL-8 is located outside the promoter region of the gene and the functional effects of the variant have not been reported. However, the CC genotype, compared to the CG (-13985C>G) resulted in an OR for BTC of 1.67 (95% CI: 1.2–2.3, P=0.03) (215). Interleukin-1 receptor antagonist (IL-1RA) is a naturally occurring anti-inflammatory cytokine, and an Indian study assessed the association between one of its genetic variants and GBC susceptibility. Specifically, the 2/2 genotype, when compared to 1/1, has an increased risk of 3.3-fold (95% CI: 1.2–8.6; P=0.02). In vitro and in vivo studies have shown allele 2 of the IL-1RN polymorphism increases the production of IL-1B (216).
The natural killer cell receptor G2D (NKG2D) is part of the innate immune system and plays an important role in tumor surveillance by modulating the activation of lymphocytes and promoting immunity to eliminate ligand-expressing cells (217). The rs11053781 and rs2617167 polymorphism of NKG2D conferred an increased risk of CCA in Scandinavian patients with PSC (OR: 2.1; 95% CI: 1.3–3.3, P=0.01; OR: 2.3; 95% CI: 1.5–3.7; P=0.002, respectively) (218). Clarifying the role of NKG2D in cholangiocarcinogenesis could stimulate the development of immunotherapies that target these molecules. However, this association was not confirmed in a validation study performed in a US cohort (211).
The MST1 gene, also referred to as MSP, codes for a protein involved in the MSP/RON signaling axis, which modifies cellular processes such as innate immunity, macrophage activation, and chemotaxis (219). MSP has been implicated in lung cancer, breast cancer, and pancreatic cancer (220). The rs3197999 variant in the MST1 gene has been associated with PSC in GWAS, and a study performed in a Caucasian population demonstrated a significant (P=0.02) association between the MST1 genotype AA and the extrahepatic subtype of BTC (OR: 2.0; 95% CI: 1.1–3.8) compared to carriers of the common GG allele (219). This missense coding variant results in a p.R689C amino acid substitution within the beta chain of the MSP protein.
Although each of the previously mentioned variants may produce a weak effect, when considered collectively a polygenic risk score (PRS) can be generated that incorporates the risk associated with each SNP; this creates a multiplicative model that allows an accurate prediction of individual risk given the number of risk alleles carried by the individual. Thus, in the future, clinicians may be able to stratify the population according to their risk level and implement appropriate surveillance strategies for early detection and even prevention of BTC. As opposed to broad knowledge of the average risk according to the population to which the patient belongs, personalized information can then be provided to each patient. More and larger GWAS are necessary to achieve this level of risk estimation for BTCs. Moreover, such GWAS must be performed in different racial groups, as the associations found in one racial group cannot be generalized to other groups.
Once common variants and the genes where they are located are identified, it is important to determine the effects of the genetic variation on individual gene expression and the pertinent cell-signaling pathways (221). This will provide insights into the molecular and cellular mechanisms affected by the genetic variation and their involvement in the pathogenesis of BTC. This knowledge may be critical to develop effective preventive and therapeutic strategies for BTC (222).
Summary, conclusions and future directions/studies
The epidemiology of BTC demonstrates substantial geographical variability due to large differences in regional prevalence of the main environmental risk factors. The risk factors with the strongest links to the development of BTC are those that induce inflammation over prolonged periods of time. Among these are infectious agents such as O. viverrini, C. sinensis, HBV, and HCV, which are more common in the Asian continent, PSC, which is the most recognized risk factor in the Western countries, and biliary stone disease. Even though these factors have been linked to carcinogenesis, no risk factor is identified in the vast majority of cases. Therefore, there is an unmet need for better identification strategies for those patients at increased risk for BTC, with the aims of early detection or prevention. By identifying SNPs that confer increased risk, clinicians may be able to identify at-risk patients and to implement appropriate surveillance strategies to monitor BTCs at earlier stages, when more effective therapeutic interventions are feasible. Clearly, we are still a long way from this goal, and therefore, there is an urgent need for large GWAS to identify and validate known as well as novel candidate SNPs. Furthermore, since the associations vary according to population, these studies must be replicated in different at-risk populations. Functional studies performed on the variants identified through GWAS could eventually shed light into potential therapeutic targets for the development of targeted precision therapies for BTC.
Acknowledgements
The authors would like to acknowledge Kevin Youel for his contribution in the creation the Figure 5.
Funding: Funding for this project was made possible by the Mayo Clinic Center for Clinical and Translational Science (UL1TR000135) and The Cholangiocarcinoma Foundation.
Footnote
Conflicts of Interest: Dr. Lewis Roberts has received grant support from Gilead Sciences, ARIAD Pharmaceuticals, Wako Diagnostics, and BTG International. The other authors have no conflicts of interest to declare.
References
- GBD 2013 Mortality and Causes of Death Collaborators. Global, regional, and national age-sex specific all-cause and cause-specific mortality for 240 causes of death, 1990-2013: a systematic analysis for the Global Burden of Disease Study 2013. Lancet 2015;385:117-71. [Crossref] [PubMed]
- Randi G, Malvezzi M, Levi F, et al. Epidemiology of biliary tract cancers: an update. Ann Oncol 2009;20:146-59. [Crossref] [PubMed]
- Yang JD, Kim B. Biliary tract cancers in Olmsted County, Minnesota, 1976-2008. Am J Gastroenterol 2012;107:1256-62. [Crossref] [PubMed]
- SEER Training Modules. Introduction to Pancreatic & Biliary Tract Cancers. Cited 2016 May 12. Available online: http://training.seer.cancer.gov/biliary/intro/
- Hezel AF, Deshpande V, Zhu AX. Genetics of biliary tract cancers and emerging targeted therapies. J Clin Oncol 2010;28:3531-40. [Crossref] [PubMed]
- Rajaraman P, Melin B, Wang Z, et al. Genome-wide association study of glioma and meta-analysis. Hum Genet 2012;131:1877-88. [Crossref] [PubMed]
- Zhang K, Civan J, Mukherjee S, et al. Genetic variations in colorectal cancer risk and clinical outcome. World J Gastroenterol 2014;20:4167-77. [Crossref] [PubMed]
- Chang YT, Chang MC, Huang KW, et al. Clinicopathological and prognostic significances of EGFR, KRAS and BRAF mutations in biliary tract carcinomas in Taiwan. J Gastroenterol Hepatol 2014;29:1119-25. [Crossref] [PubMed]
- American Cancer Society. What is bile duct cancer? Available online: http://www.cancer.org/cancer/bileductcancer/detailedguide/bile-duct-cancer-what-is-bile-duct-cancer
- de Groen PC, Gores GJ, LaRusso NF, et al. Biliary tract cancers. N Engl J Med 1999;341:1368-78. [Crossref] [PubMed]
- Nakeeb A, Pitt HA, Sohn TA, et al. Cholangiocarcinoma. A spectrum of intrahepatic, perihilar, and distal tumors. Ann Surg 1996;224:463-73. [Crossref] [PubMed]
- DeOliveira ML, Cunningham SC, Cameron JL, et al. Cholangiocarcinoma: thirty-one-year experience with 564 patients at a single institution. Ann Surg 2007;245:755-62. [Crossref] [PubMed]
- Lowe RC, Afdhal NH, Anderson CD. Epidemiology, pathogenesis, and classification of cholangiocarcinoma. Available online: http://www.uptodate.com/contents/epidemiology-pathogenesis-and-classification-of-cholangiocarcinoma
- Bergquist A, von Seth E. Epidemiology of cholangiocarcinoma. Best Pract Res Clin Gastroenterol 2015;29:221-32. [Crossref] [PubMed]
- Arroyo GF, Gentile A, Parada LA. Gallbladder cancer: South American experience. Chin Clin Oncol 2016;5:67.
- Lazcano-Ponce EC, Miquel JF, Munoz N, et al. Epidemiology and molecular pathology of gallbladder cancer. CA Cancer J Clin 2001;51:349-64. [Crossref] [PubMed]
- Diehl AK. Epidemiology of gallbladder cancer: a synthesis of recent data. J Natl Cancer Inst 1980;65:1209-14. [PubMed]
- Mehrotra B. Gallbladder Cancer: Epidemiology, Risk Factors, Clinical Features, and Diagnosis. Accessed on July 29, 2015. Available online: http://www.uptodate.com/contents/gallbladder-cancer-epidemiology-risk-factors-clinical-features-and-diagnosis
- Randi G, Franceschi S, La Vecchia C. Gallbladder cancer worldwide: geographical distribution and risk factors. Int J Cancer 2006;118:1591-602. [Crossref] [PubMed]
- Henley SJ, Weir HK, Jim MA, et al. Gallbladder Cancer Incidence and Mortality, United States 1999-2011. Cancer Epidemiol Biomarkers Prev 2015;24:1319-26. [Crossref] [PubMed]
- Castro FA, Koshiol J, Hsing AW, et al. Biliary tract cancer incidence in the United States-Demographic and temporal variations by anatomic site. Int J Cancer 2013;133:1664-71. [Crossref] [PubMed]
- Mosadeghi S, Liu B, Bhuket T, et al. Sex-specific and race/ethnicity-specific disparities in cholangiocarcinoma incidence and prevalence in the USA: An updated analysis of the 2000-2011 Surveillance, Epidemiology and End Results registry. Hepatol Res 2016;46:669-77. [PubMed]
- Alberts SR, Kelly JJ, Ashokkumar R, et al. Occurrence of pancreatic, biliary tract, and gallbladder cancers in Alaska Native people, 1973-2007. Int J Circumpolar Health 2012;71:17521. [Crossref] [PubMed]
- Sithithaworn P, Yongvanit P, Duenngai K, et al. Roles of liver fluke infection as risk factor for cholangiocarcinoma. J Hepatobiliary Pancreat Sci 2014;21:301-8. [Crossref] [PubMed]
- Sriamporn S, Pisani P, Pipitgool V, et al. Prevalence of Opisthorchis viverrini infection and incidence of cholangiocarcinoma in Khon Kaen, Northeast Thailand. Trop Med Int Health 2004;9:588-94. [Crossref] [PubMed]
- Vatanasapt V, Martin N, Sriplung H, et al. Cancer incidence in Thailand, 1988-1991. Cancer Epidemiol Biomarkers Prev 1995;4:475-83. [PubMed]
- Sripa B, Pairojkul C. Cholangiocarcinoma: lessons from Thailand. Curr Opin Gastroenterol 2008;24:349-56. [Crossref] [PubMed]
- Hsing AW, Bai Y, Andreotti G, et al. Family history of gallstones and the risk of biliary tract cancer and gallstones: a population-based study in Shanghai, China. Int J Cancer 2007;121:832-8. [Crossref] [PubMed]
- Paraskevopoulos JA, Dennison AR, Ross B, et al. Primary carcinoma of the gallbladder: a 10-year experience. Ann R Coll Surg Engl 1992;74:222-4. [PubMed]
- Kaushik SP. Current perspectives in gallbladder carcinoma. J Gastroenterol Hepatol 2001;16:848-54. [Crossref] [PubMed]
- Carriaga MT, Henson DE. Liver, gallbladder, extrahepatic bile ducts, and pancreas. Cancer 1995;75:171-90. [Crossref] [PubMed]
- Wistuba II, Gazdar AF. Gallbladder cancer: lessons from a rare tumour. Nat Rev Cancer 2004;4:695-706. [Crossref] [PubMed]
- Kanthan R, Senger JL, Ahmed S, et al. Gallbladder Cancer in the 21st Century. J Oncol 2015;2015:967472.
- Misra S, Chaturvedi A, Misra NC, et al. Carcinoma of the gallbladder. Lancet Oncol 2003;4:167-76. [Crossref] [PubMed]
- Su CH, Lui WY, P'eng FK. Relative prevalence of gallstone diseases in Taiwan. A nationwide cooperative study. Dig Dis Sci 1992;37:764-8. [Crossref] [PubMed]
- Tyson GL, El-Serag HB. Risk factors for cholangiocarcinoma. Hepatology 2011;54:173-84. [Crossref] [PubMed]
- Lee TY, Lee SS, Jung SW, et al. Hepatitis B virus infection and intrahepatic cholangiocarcinoma in Korea: a case-control study. Am J Gastroenterol 2008;103:1716-20. [Crossref] [PubMed]
- Eaton JE, Talwalkar JA, Lazaridis KN, et al. Pathogenesis of primary sclerosing cholangitis and advances in diagnosis and management. Gastroenterology 2013;145:521-36. [Crossref] [PubMed]
- Lee YM, Kaplan MM. Primary sclerosing cholangitis. N Engl J Med 1995;332:924-33. [Crossref] [PubMed]
- Bergquist A, Ekbom A, Olsson R, et al. Hepatic and extrahepatic malignancies in primary sclerosing cholangitis. J Hepatol 2002;36:321-7. [Crossref] [PubMed]
- Burak K, Angulo P, Pasha TM, et al. Incidence and risk factors for cholangiocarcinoma in primary sclerosing cholangitis. Am J Gastroenterol 2004;99:523-6. [Crossref] [PubMed]
- Boberg KM, Bergquist A, Mitchell S, et al. Cholangiocarcinoma in primary sclerosing cholangitis: risk factors and clinical presentation. Scand J Gastroenterol 2002;37:1205-11. [Crossref] [PubMed]
- Bergquist A, Glaumann H, Persson B, et al. Risk factors and clinical presentation of hepatobiliary carcinoma in patients with primary sclerosing cholangitis: a case-control study. Hepatology 1998.311-6. [Crossref] [PubMed]
- Chapman MH, Webster GJ, Bannoo S, et al. Cholangiocarcinoma and dominant strictures in patients with primary sclerosing cholangitis: a 25-year single-centre experience. Eur J Gastroenterol Hepatol 2012;24:1051-8. [Crossref] [PubMed]
- Lazaridis KN, LaRusso NF. The Cholangiopathies. Mayo Clin Proc 2015;90:791-800. [Crossref] [PubMed]
- Eaton JE, Juran BD, Atkinson EJ, et al. A comprehensive assessment of environmental exposures among 1000 North American patients with primary sclerosing cholangitis, with and without inflammatory bowel disease. Aliment Pharmacol Ther 2015;41:980-90. [Crossref] [PubMed]
- Björnsson E, Angulo P. Cholangiocarcinoma in young individuals with and without primary sclerosing cholangitis. Am J Gastroenterol 2007;102:1677-82. [Crossref] [PubMed]
- Fevery J, Henckaerts L, Van Oirbeek R, et al. Malignancies and mortality in 200 patients with primary sclerosering cholangitis: a long-term single-centre study. Liver Int 2012;32:214-22. [Crossref] [PubMed]
- Williamson KD, Chapman RW. Primary sclerosing cholangitis: a clinical update. Br Med Bull 2015;114:53-64. [Crossref] [PubMed]
- Bergquist A, Broomé U. Hepatobiliary and extra-hepatic malignancies in primary sclerosing cholangitis. Best Pract Res Clin Gastroenterol 2001;15:643-56. [Crossref] [PubMed]
- Rizvi S, Eaton JE, Gores GJ. Primary Sclerosing Cholangitis as a Premalignant Biliary Tract Disease: Surveillance and Management. Clin Gastroenterol Hepatol 2015;13:2152-65. [Crossref] [PubMed]
- Karlsen TH, Melum E, Franke A. The utility of genome-wide association studies in hepatology. Hepatology 2010;51:1833-42. [Crossref] [PubMed]
- Karlsen TH, Schrumpf E, Boberg KM. Primary sclerosing cholangitis. Best Pract Res Clin Gastroenterol 2010;24:655-66. [Crossref] [PubMed]
- Ellinghaus D, Jostins L, Spain SL, et al. Analysis of five chronic inflammatory diseases identifies 27 new associations and highlights disease-specific patterns at shared loci. Nat Genet 2016;48:510-8. [Crossref] [PubMed]
- Grainge MJ, West J, Solaymani-Dodaran M, et al. The antecedents of biliary cancer: a primary care case-control study in the United Kingdom. Br J Cancer 2009;100:178-80. [Crossref] [PubMed]
- Welzel TM, Graubard BI, El-Serag HB, et al. Risk factors for intrahepatic and extrahepatic cholangiocarcinoma in the United States: a population-based case-control study. Clin Gastroenterol Hepatol 2007;5:1221-8. [Crossref] [PubMed]
- Shebl FM, Andreotti G, Rashid A, et al. Diabetes in relation to biliary tract cancer and stones: a population-based study in Shanghai, China. Br J Cancer 2010;103:115-9. [Crossref] [PubMed]
- Shaib YH, El-Serag HB, Davila JA, et al. Risk factors of intrahepatic cholangiocarcinoma in the United States: a case-control study. Gastroenterology 2005;128:620-6. [Crossref] [PubMed]
- Lee BS, Park EC, Park SW, et al. Hepatitis B virus infection, diabetes mellitus, and their synergism for cholangiocarcinoma development: a case-control study in Korea. World J Gastroenterol 2015;21:502-10. [Crossref] [PubMed]
- Engeland A, Tretli S, Austad G, et al. Height and body mass index in relation to colorectal and gallbladder cancer in two million Norwegian men and women. Cancer Causes Control 2005;16:987-96. [Crossref] [PubMed]
- Hsing AW, Sakoda LC, Rashid A, et al. Body size and the risk of biliary tract cancer: a population-based study in China. Br J Cancer 2008;99:811-5. [Crossref] [PubMed]
- Ren HB, Yu T, Liu C, et al. Diabetes mellitus and increased risk of biliary tract cancer: systematic review and meta-analysis. Cancer Causes Control 2011;22:837-47. [Crossref] [PubMed]
- Jing W, Jin G, Zhou X, et al. Diabetes mellitus and increased risk of cholangiocarcinoma: a meta-analysis. Eur J Cancer Prev 2012;21:24-31. [Crossref] [PubMed]
- Zhang LF, Zhao HX. Diabetes mellitus and increased risk of extrahepatic cholangiocarcinoma: a meta-analysis. Hepatogastroenterology 2013;60:684-7. [PubMed]
- Lee MS, Hsu CC, Wahlqvist ML, et al. Type 2 diabetes increases and metformin reduces total, colorectal, liver and pancreatic cancer incidences in Taiwanese: a representative population prospective cohort study of 800,000 individuals. BMC Cancer 2011;11:20. [Crossref] [PubMed]
- Evans JM, Donnelly LA, Emslie-Smith AM, et al. Metformin and reduced risk of cancer in diabetic patients. BMJ 2005;330:1304-5. [Crossref] [PubMed]
- Chaiteerakij R, Yang JD, Harmsen WS, et al. Risk factors for intrahepatic cholangiocarcinoma: association between metformin use and reduced cancer risk. Hepatology 2013;57:648-55. [Crossref] [PubMed]
- Ling S, Feng T, Ke Q, et al. Metformin inhibits proliferation and enhances chemosensitivity of intrahepatic cholangiocarcinoma cell lines. Oncol Rep 2014;31:2611-8. [PubMed]
- Jang MH, Lee YJ, Kim H. Intrahepatic cholangiocarcinoma arising in Caroli's disease. Clin Mol Hepatol 2014;20:402-5. [Crossref] [PubMed]
- Dayton MT, Longmire WP Jr, Tompkins RK. Caroli's Disease: a premalignant condition? Am J Surg 1983;145:41-8. [Crossref] [PubMed]
- Chapman RW. Risk factors for biliary tract carcinogenesis. Ann Oncol 1999;10 Suppl 4:308-11. [Crossref] [PubMed]
- Lipsett PA, Pitt HA, Colombani PM, et al. Choledochal cyst disease. A changing pattern of presentation. Ann Surg 1994;220:644-52. [Crossref] [PubMed]
- Scott J, Shousha S, Thomas HC, et al. Bile duct carcinoma: a late complication of congenital hepatic fibrosis. Case report and review of literature. Am J Gastroenterol 1980;73:113-9. [PubMed]
- Arora A, Mukund A, Thapar S, et al. Anomalous pancreaticobiliary junction. J Pediatr Gastroenterol Nutr 2014;58:e13. [Crossref] [PubMed]
- Kamisawa T, Kuruma S, Chiba K, et al. Biliary carcinogenesis in pancreaticobiliary maljunction. J Gastroenterol 2016. [Epub ahead of print]. [Crossref] [PubMed]
- Watanapa P, Watanapa WB. Liver fluke-associated cholangiocarcinoma. Br J Surg 2002;89:962-70. [Crossref] [PubMed]
- Shin HR, Oh JK, Masuyer E, et al. Epidemiology of cholangiocarcinoma: an update focusing on risk factors. Cancer Sci 2010;101:579-85. [Crossref] [PubMed]
- Bragazzi MC, Cardinale V, Carpino G, et al. Cholangiocarcinoma: Epidemiology and risk factors. Transl Gastrointest Cancer 2012;1:21-32.
- Shaib Y, El-Serag HB. The epidemiology of cholangiocarcinoma. Semin Liver Dis 2004;24:115-25. [Crossref] [PubMed]
- Lim JH. Liver flukes: the malady neglected. Korean J Radiol 2011;12:269-79. [Crossref] [PubMed]
- Kurathong S, Lerdverasirikul P, Wongpaitoon V, et al. Opisthorchis viverrini infection and cholangiocarcinoma. A prospective, case-controlled study. Gastroenterology 1985;89:151-6. [Crossref] [PubMed]
- Jang KT, Hong SM, Lee KT, et al. Intraductal papillary neoplasm of the bile duct associated with Clonorchis sinensis infection. Virchows Arch 2008;453:589-98. [Crossref] [PubMed]
- Sripa B, Bethony JM, Sithithaworn P, et al. Opisthorchiasis and Opisthorchis-associated cholangiocarcinoma in Thailand and Laos. Acta Trop 2011;120 Suppl 1:S158-68. [Crossref] [PubMed]
- Honjo S, Srivatanakul P, Sriplung H, et al. Genetic and environmental determinants of risk for cholangiocarcinoma via Opisthorchis viverrini in a densely infested area in Nakhon Phanom, northeast Thailand. Int J Cancer 2005;117:854-60. [Crossref] [PubMed]
- Zhang GW, Lin JH, Qian JP, et al. Identification of risk and prognostic factors for patients with clonorchiasis-associated intrahepatic cholangiocarcinoma. Ann Surg Oncol 2014;21:3628-37. [Crossref] [PubMed]
- Khan SA, Thomas HC, Davidson BR, et al. Cholangiocarcinoma. Lancet 2005;366:1303-14. [Crossref] [PubMed]
- Parkin DM. The global health burden of infection-associated cancers in the year 2002. Int J Cancer 2006;118:3030-44. [Crossref] [PubMed]
- Shepard CW, Finelli L, Alter MJ. Global epidemiology of hepatitis C virus infection. Lancet Infect Dis 2005;5:558-67. [Crossref] [PubMed]
- El-Serag HB, Engels EA, Landgren O, et al. Risk of hepatobiliary and pancreatic cancers after hepatitis C virus infection: A population-based study of U.S. veterans. Hepatology 2009;49:116-23. [Crossref] [PubMed]
- Li M, Li J, Li P, et al. Hepatitis B virus infection increases the risk of cholangiocarcinoma: a meta-analysis and systematic review. J Gastroenterol Hepatol 2012;27:1561-8. [Crossref] [PubMed]
- Zhou HB, Hu JY, Hu HP. Hepatitis B virus infection and intrahepatic cholangiocarcinoma. World J Gastroenterol 2014;20:5721-9. [Crossref] [PubMed]
- Palmer WC, Patel T. Are common factors involved in the pathogenesis of primary liver cancers? A meta-analysis of risk factors for intrahepatic cholangiocarcinoma. J Hepatol 2012;57:69-76. [Crossref] [PubMed]
- Zhou Y, Zhao Y, Li B, et al. Hepatitis viruses infection and risk of intrahepatic cholangiocarcinoma: evidence from a meta-analysis. BMC Cancer 2012;12:289. [Crossref] [PubMed]
- Lee CH, Chang CJ, Lin YJ, et al. Viral hepatitis-associated intrahepatic cholangiocarcinoma shares common disease processes with hepatocellular carcinoma. Br J Cancer 2009;100:1765-70. [Crossref] [PubMed]
- Vladoianu IR, Chang HR, Pechère JC. Expression of host resistance to Salmonella typhi and Salmonella typhimurium: bacterial survival within macrophages of murine and human origin. Microb Pathog 1990;8:83-90. [Crossref] [PubMed]
- Kumar S, Kumar S, Kumar S. Infection as a risk factor for gallbladder cancer. J Surg Oncol 2006;93:633-9. [Crossref] [PubMed]
- Mager DL. Bacteria and cancer: cause, coincidence or cure? A review. J Transl Med 2006;4:14. [Crossref] [PubMed]
- Hornick RB, Greisman SE, Woodward TE, et al. Typhoid fever: pathogenesis and immunologic control. N Engl J Med 1970;283:686-91. [Crossref] [PubMed]
- Parry CM, Hien TT, Dougan G, et al. Typhoid fever. N Engl J Med 2002;347:1770-82. [Crossref] [PubMed]
- Vogelsang TM, Bøe J. Temporary and chronic carriers of Salmonella typhi and Salmonella paratyphi B. J Hyg (Lond) 1948;46:252-61. [Crossref] [PubMed]
- Khatri NS, Maskey P, Poudel S, et al. Gallbladder carriage of Salmonella paratyphi A may be an important factor in the increasing incidence of this infection in South Asia. Ann Intern Med 2009;150:567-8. [Crossref] [PubMed]
- Bhan MK, Bahl R, Bhatnagar S. Typhoid and paratyphoid fever. Lancet 2005;366:749-62. [Crossref] [PubMed]
- Crump JA, Luby SP, Mintz ED. The global burden of typhoid fever. Bull World Health Organ 2004;82:346-53. [PubMed]
- Merselis JG Jr, Kaye D, Connolly CS, et al. Quantitative bacteriology of the typhoid carrier state. Am J Trop Med Hyg 1964;13:425-9. [PubMed]
- Levine MM, Black RE, Lanata C. Precise estimation of the numbers of chronic carriers of Salmonella typhi in Santiago, Chile, an endemic area. J Infect Dis 1982;146:724-6. [Crossref] [PubMed]
- Sinnott CR, Teall AJ. Persistent gallbladder carriage of Salmonella typhi. Lancet 1987;1:976. [Crossref] [PubMed]
- Shpargel JS, Berardi RS, Lenz D. Salmonella typhi carrier state 52 years after illness with typhoid fever: a case study. Am J Infect Control 1985;13:122-3. [Crossref] [PubMed]
- Nath G, Singh H, Shukla VK. Chronic typhoid carriage and carcinoma of the gallbladder. Eur J Cancer Prev 1997;6:557-9. [Crossref] [PubMed]
- Nagaraja V, Eslick GD. Systematic review with meta-analysis: the relationship between chronic Salmonella typhi carrier status and gall-bladder cancer. Aliment Pharmacol Ther 2014;39:745-50. [Crossref] [PubMed]
- Dutta U, Garg PK, Kumar R, et al. Typhoid carriers among patients with gallstones are at increased risk for carcinoma of the gallbladder. Am J Gastroenterol 2000;95:784-7. [Crossref] [PubMed]
- Caygill CP, Hill MJ, Braddick M, et al. Cancer mortality in chronic typhoid and paratyphoid carriers. Lancet 1994;343:83-4. [Crossref] [PubMed]
- Fukuda K, Kuroki T, Tajima Y, et al. Comparative analysis of Helicobacter DNAs and biliary pathology in patients with and without hepatobiliary cancer. Carcinogenesis 2002;23:1927-31. [Crossref] [PubMed]
- Chen W, Li D, Cannan RJ, et al. Common presence of Helicobacter DNA in the gallbladder of patients with gallstone diseases and controls. Dig Liver Dis 2003;35:237-43. [Crossref] [PubMed]
- Huang Y, Fan XG, Wang ZM, et al. Identification of helicobacter species in human liver samples from patients with primary hepatocellular carcinoma. J Clin Pathol 2004;57:1273-7. [Crossref] [PubMed]
- Chang JS, Tsai CR, Chen LT. Medical risk factors associated with cholangiocarcinoma in Taiwan: a population-based case-control study. PLoS One 2013;8:e69981. [Crossref] [PubMed]
- Bulajic M, Maisonneuve P, Schneider-Brachert W, et al. Helicobacter pylori and the risk of benign and malignant biliary tract disease. Cancer 2002;95:1946-53. [Crossref] [PubMed]
- Pandey M, Shukla M. Helicobacter species are associated with possible increase in risk of hepatobiliary tract cancers. Surg Oncol 2009;18:51-6. [Crossref] [PubMed]
- Murphy G, Michel A, Taylor PR, et al. Association of seropositivity to Helicobacter species and biliary tract cancer in the ATBC study. Hepatology 2014;60:1963-71. [Crossref] [PubMed]
- Leelawat K, Suksumek N, Leelawat S, et al. Detection of VacA gene specific for Helicobactor pylori in hepatocellular carcinoma and cholangiocarcinoma specimens of Thai patients. Southeast Asian J Trop Med Public Health 2007;38:881-5. [PubMed]
- Vorobjova T, Nilsson I, Terjajev S, et al. Serum antibodies to enterohepatic Helicobacter spp. in patients with chronic liver diseases and in a population with high prevalence of H. pylori infection. Dig Liver Dis 2006;38:171-6. [Crossref] [PubMed]
- Murata H, Tsuji S, Tsujii M, et al. Helicobacter bilis infection in biliary tract cancer. Aliment Pharmacol Ther 2004;20 Suppl 1:90-4. [Crossref] [PubMed]
- Matsukura N, Yokomuro S, Yamada S, et al. Association between Helicobacter bilis in bile and biliary tract malignancies: H. bilis in bile from Japanese and Thai patients with benign and malignant diseases in the biliary tract. Jpn J Cancer Res 2002;93:842-7. [Crossref] [PubMed]
- Romero S, Archer JR, Hamacher ME, et al. Case report of an unclassified microaerophilic bacterium associated with gastroenteritis. J Clin Microbiol 1988;26:142-3. [PubMed]
- Zhou D, Wang JD, Weng MZ, et al. Infections of Helicobacter spp. in the biliary system are associated with biliary tract cancer: a meta-analysis. Eur J Gastroenterol Hepatol 2013;25:447-54. [PubMed]
- Silva CP, Pereira-Lima JC, Oliveira AG, et al. Association of the presence of Helicobacter in gallbladder tissue with cholelithiasis and cholecystitis. J Clin Microbiol 2003;41:5615-8. [Crossref] [PubMed]
- Pellicano R, Mazzaferro V, Grigioni WF, et al. Helicobacter species sequences in liver samples from patients with and without hepatocellular carcinoma. World J Gastroenterol 2004;10:598-601. [Crossref] [PubMed]
- Fox JG, Dewhirst FE, Tully JG, et al. Helicobacter hepaticus sp. nov., a microaerophilic bacterium isolated from livers and intestinal mucosal scrapings from mice. J Clin Microbiol 1994;32:1238-45. [PubMed]
- Maurer KJ, Ihrig MM, Rogers AB, et al. Identification of cholelithogenic enterohepatic helicobacter species and their role in murine cholesterol gallstone formation. Gastroenterology 2005;128:1023-33. [Crossref] [PubMed]
- Takayama S, Takahashi H, Matsuo Y, et al. Effect of Helicobacter bilis infection on human bile duct cancer cells. Dig Dis Sci 2010;55:1905-10. [Crossref] [PubMed]
- Tak PP, Firestein GS. NF-kappaB: a key role in inflammatory diseases. J Clin Invest 2001;107:7-11. [Crossref] [PubMed]
- Segura-López FK, Güitrón-Cantú A, Torres J. Association between Helicobacter spp. infections and hepatobiliary malignancies: a review. World J Gastroenterol 2015;21:1414-23. [Crossref] [PubMed]
- Aflatoxins. Available online: http://www.cancer.gov/about-cancer/causes-prevention/risk/substances/aflatoxins
- World Health Organization. Public Health Strategies for Preventing Aflatoxin Exposure. Available online: http://www.who.int/ipcs/events/2005/workshop_report.pdf
- Tsuchiya Y, Terao M, Okano K, et al. Mutagenicity and mutagens of the red chili pepper as gallbladder cancer risk factor in Chilean women. Asian Pac J Cancer Prev 2011;12:471-6. [PubMed]
- World Health Organization. International agency for research on cancer. iarc monographs on the evaluation of carcinogenic risks to humans. 2002. Available online: http://monographs.iarc.fr/ENG/Monographs/vol82/mono82.pdf
- Nogueira L, Foerster C, Groopman J, et al. Association of aflatoxin with gallbladder cancer in Chile. JAMA 2015;313:2075-7. [Crossref] [PubMed]
- Ikoma T, Tsuchiya Y, Asai T, et al. Ochratoxin A Contamination of Red Chili Peppers from Chile, Bolivia and Peru, Countries with a High Incidence of Gallbladder Cancer. Asian Pac J Cancer Prev 2015;16:5987-91. [Crossref] [PubMed]
- Ringot D, Chango A, Schneider YJ, et al. Toxicokinetics and toxicodynamics of ochratoxin A, an update. Chem Biol Interact 2006;159:18-46. [Crossref] [PubMed]
- Yamada K, Kumagai S, Nagoya T, et al. Chemical exposure levels in printing workers with cholangiocarcinoma. J Occup Health 2014;56:332-8. [Crossref] [PubMed]
- Yamada K, Kumagai S, Kubo S, et al. Chemical exposure levels in printing and coating workers with cholangiocarcinoma (third report). J Occup Health 2015;57:565-71. [Crossref] [PubMed]
- Yamada K, Kumagai S, Endo G. Chemical exposure levels in printing workers with cholangiocarcinoma (second report). J Occup Health 2015;57:245-52. [Crossref] [PubMed]
- Kubo S, Nakanuma Y, Takemura S, et al. Case series of 17 patients with cholangiocarcinoma among young adult workers of a printing company in Japan. J Hepatobiliary Pancreat Sci 2014;21:479-88. [Crossref] [PubMed]
- Kubo S, Kinoshita M, Takemura S, et al. Characteristics of printing company workers newly diagnosed with occupational cholangiocarcinoma. J Hepatobiliary Pancreat Sci 2014;21:809-17. [Crossref] [PubMed]
- Kumagai S. Two offset printing workers with cholangiocarcinoma. J Occup Health 2014;56:164-8. [Crossref] [PubMed]
- American Cancer Society. Gallbladder Cancer. 2014. Available online: http://www.cancer.org/acs/groups/cid/documents/webcontent/003101-pdf.pdf
- Titapun A, Pugkhem A, Luvira V, et al. Outcome of curative resection for perihilar cholangiocarcinoma in Northeast Thailand. World J Gastrointest Oncol 2015;7:503-12. [PubMed]
- Mavros MN, Economopoulos KP, Alexiou VG, et al. Treatment and Prognosis for Patients With Intrahepatic Cholangiocarcinoma: Systematic Review and Meta-analysis. JAMA Surg 2014;149:565-74. [Crossref] [PubMed]
- Courtin-Tanguy L, Rayar M, Bergeat D, et al. The true prognosis of resected distal cholangiocarcinoma. J Surg Oncol 2016;113:575-80. [Crossref] [PubMed]
- Hu HJ, Mao H, Shrestha A, et al. Prognostic factors and long-term outcomes of hilar cholangiocarcinoma: A single-institution experience in China. World J Gastroenterol 2016;22:2601-10. [Crossref] [PubMed]
- Wang Z, Sheng YY, Dong QZ, et al. Hepatitis B virus and hepatitis C virus play different prognostic roles in intrahepatic cholangiocarcinoma: A meta-analysis. World J Gastroenterol 2016;22:3038-51. [Crossref] [PubMed]
- Miquel JF, Covarrubias C, Villaroel L, et al. Genetic epidemiology of cholesterol cholelithiasis among Chilean Hispanics, Amerindians, and Maoris. Gastroenterology 1998;115:937-46. [Crossref] [PubMed]
- Yamagiwa H. Mucosal dysplasia of gallbladder: isolated and adjacent lesions to carcinoma. Jpn J Cancer Res 1989;80:238-43. [Crossref] [PubMed]
- Yamagiwa H, Tomiyama H. Intestinal metaplasia-dysplasia-carcinoma sequence of the gallbladder. Acta Pathol Jpn 1986;36:989-97. [PubMed]
- Yanagisawa N, Mikami T, Saegusa M, et al. More frequent beta-catenin exon 3 mutations in gallbladder adenomas than in carcinomas indicate different lineages. Cancer Res 2001;61:19-22. [PubMed]
- Rashid A, Gao YT, Bhakta S, et al. Beta-catenin mutations in biliary tract cancers: a population-based study in China. Cancer Res 2001;61:3406-9. [PubMed]
- Chang HJ, Jee CD, Kim WH. Mutation and altered expression of beta-catenin during gallbladder carcinogenesis. Am J Surg Pathol 2002;26:758-66. [Crossref] [PubMed]
- Fava G, Lorenzini I. Molecular pathogenesis of cholangiocarcinoma. Int J Hepatol 2012;2012:630543.
- Patel T. New insights into the molecular pathogenesis of intrahepatic cholangiocarcinoma. J Gastroenterol 2014;49:165-72. [Crossref] [PubMed]
- Choi J, Ghoz HM, Peeraphatdit T, et al. Aspirin use and the risk of cholangiocarcinoma. Hepatology 2016;64:785-96. [Crossref] [PubMed]
- Bickenbach K, Galka E, Roggin KK. Molecular mechanisms of cholangiocarcinogenesis: are biliary intraepithelial neoplasia and intraductal papillary neoplasms of the bile duct precursors to cholangiocarcinoma? Surg Oncol Clin N Am 2009;18:215-24. vii. [Crossref] [PubMed]
- Taniai M, Higuchi H, Burgart LJ, et al. p16INK4a promoter mutations are frequent in primary sclerosing cholangitis (PSC) and PSC-associated cholangiocarcinoma. Gastroenterology 2002;123:1090-8. [Crossref] [PubMed]
- Itatsu K, Zen Y, Ohira S, et al. Immunohistochemical analysis of the progression of flat and papillary preneoplastic lesions in intrahepatic cholangiocarcinogenesis in hepatolithiasis. Liver Int 2007;27:1174-84. [Crossref] [PubMed]
- Andersen JB. Molecular pathogenesis of intrahepatic cholangiocarcinoma. J Hepatobiliary Pancreat Sci 2015;22:101-13. [Crossref] [PubMed]
- Chan-On W, Nairismägi ML, Ong CK, et al. Exome sequencing identifies distinct mutational patterns in liver fluke-related and non-infection-related bile duct cancers. Nat Genet 2013;45:1474-8. [Crossref] [PubMed]
- Jiao Y, Pawlik TM, Anders RA, et al. Exome sequencing identifies frequent inactivating mutations in BAP1, ARID1A and PBRM1 in intrahepatic cholangiocarcinomas. Nat Genet 2013;45:1470-3. [Crossref] [PubMed]
- Huang WY, Gao YT, Rashid A, et al. Selected base excision repair gene polymorphisms and susceptibility to biliary tract cancer and biliary stones: a population-based case-control study in China. Carcinogenesis 2008;29:100-5. [Crossref] [PubMed]
- Gao Q, Zhao YJ, Wang XY, et al. Activating mutations in PTPN3 promote cholangiocarcinoma cell proliferation and migration and are associated with tumor recurrence in patients. Gastroenterology 2014;146:1397-407. [Crossref] [PubMed]
- Sia D, Losic B, Moeini A, et al. Massive parallel sequencing uncovers actionable FGFR2-PPHLN1 fusion and ARAF mutations in intrahepatic cholangiocarcinoma. Nat Commun 2015;6:6087. [Crossref] [PubMed]
- Zou S, Li J, Zhou H, et al. Mutational landscape of intrahepatic cholangiocarcinoma. Nat Commun 2014;5:5696. [Crossref] [PubMed]
- Nakamura H, Arai Y, Totoki Y, et al. Genomic spectra of biliary tract cancer. Nat Genet 2015;47:1003-10. [Crossref] [PubMed]
- Wang LJ, He CC, Sui X, et al. MiR-21 promotes intrahepatic cholangiocarcinoma proliferation and growth in vitro and in vivo by targeting PTPN14 and PTEN. Oncotarget 2015;6:5932-46. [Crossref] [PubMed]
- Chen MH, Chiang KC, Cheng CT, et al. Antitumor activity of the combination of an HSP90 inhibitor and a PI3K/mTOR dual inhibitor against cholangiocarcinoma. Oncotarget 2014;5:2372-89. [Crossref] [PubMed]
- Churi CR, Shroff R, Wang Y, et al. Mutation profiling in cholangiocarcinoma: prognostic and therapeutic implications. PLoS One 2014;9:e115383. [Crossref] [PubMed]
- Moeini A, Sia D, Bardeesy N, et al. Molecular Pathogenesis and Targeted Therapies for Intrahepatic Cholangiocarcinoma. Clin Cancer Res 2016;22:291-300. [Crossref] [PubMed]
- Andersen JB, Spee B, Blechacz BR, et al. Genomic and genetic characterization of cholangiocarcinoma identifies therapeutic targets for tyrosine kinase inhibitors. Gastroenterology 2012;142:1021-1031.e15. [Crossref] [PubMed]
- Sia D, Hoshida Y, Villanueva A, et al. Integrative molecular analysis of intrahepatic cholangiocarcinoma reveals 2 classes that have different outcomes. Gastroenterology 2013;144:829-40. [Crossref] [PubMed]
- Voss JS, Holtegaard LM, Kerr SE, et al. Molecular profiling of cholangiocarcinoma shows potential for targeted therapy treatment decisions. Hum Pathol 2013;44:1216-22. [Crossref] [PubMed]
- Lee H, Wang K, Johnson A, et al. Comprehensive genomic profiling of extrahepatic cholangiocarcinoma reveals a long tail of therapeutic targets. J Clin Pathol 2016;69:403-8. [Crossref] [PubMed]
- Craig J. Complex Diseases: Research and Applications Nature Education. 2008. Cited May 17, 2016. Available online: http://www.nature.com/scitable/topicpage/complex-diseases-research-and-applications-748
- Attia J. Genetic association studies: Principles and applications. Available online: http://www.uptodate.com/contents/genetic-association-studies-principles-and-applications?source=search_result&search=genetic+association+studies&selectedTitle=1%7E68
- Attia J, Ioannidis JP, Thakkinstian A, et al. How to use an article about genetic association: A: Background concepts. JAMA 2009;301:74-81. [Crossref] [PubMed]
- Tabor HK, Risch NJ, Myers RM. Candidate-gene approaches for studying complex genetic traits: practical considerations. Nat Rev Genet 2002;3:391-7. [Crossref] [PubMed]
- Cha PC, Zembutsu H, Takahashi A, et al. A genome-wide association study identifies SNP in DCC is associated with gallbladder cancer in the Japanese population. J Hum Genet 2012;57:235-7. [Crossref] [PubMed]
- Johnson EF, Connick JP, Reed JR, et al. Correlating structure and function of drug-metabolizing enzymes: progress and ongoing challenges. Drug Metab Dispos 2014;42:9-22. [Crossref] [PubMed]
- Agundez JA. Cytochrome P450 gene polymorphism and cancer. Curr Drug Metab 2004;5:211-24. [Crossref] [PubMed]
- Shimada T. Xenobiotic-metabolizing enzymes involved in activation and detoxification of carcinogenic polycyclic aromatic hydrocarbons. Drug Metab Pharmacokinet 2006;21:257-76. [Crossref] [PubMed]
- Pandey SN, Choudhuri G, Mittal B. Association of CYP1A1 Msp1 polymorphism with tobacco-related risk of gallbladder cancer in a north Indian population. Eur J Cancer Prev 2008;17:77-81. [Crossref] [PubMed]
- Bartsch H, Nair U, Risch A, et al. Genetic polymorphism of CYP genes, alone or in combination, as a risk modifier of tobacco-related cancers. Cancer Epidemiol Biomarkers Prev 2000;9:3-28. [PubMed]
- Tsuchiya Y, Kiyohara C, Sato T, et al. Polymorphisms of cytochrome P450 1A1, glutathione S-transferase class mu, and tumour protein p53 genes and the risk of developing gallbladder cancer in Japanese. Clin Biochem 2007;40:881-6. [Crossref] [PubMed]
- Park SK, Andreotti G, Sakoda LC, et al. Variants in hormone-related genes and the risk of biliary tract cancers and stones: a population-based study in China. Carcinogenesis 2009;30:606-14. [Crossref] [PubMed]
- Sangrajrang S, Sato Y, Sakamoto H, et al. Genetic polymorphisms of estrogen metabolizing enzyme and breast cancer risk in Thai women. Int J Cancer 2009;125:837-43. [Crossref] [PubMed]
- Dwivedi S, Agrawal S, Singh S, et al. Association of Cytochrome-17 (MspA1) Gene Polymorphism with Risk of Gall Bladder Stones and Cancer in North India. Asian Pac J Cancer Prev 2015;16:5557-63. [Crossref] [PubMed]
- Hou L, Xu J, Gao YT, et al. CYP17 MspA1 polymorphism and risk of biliary tract cancers and gallstones: a population-based study in Shanghai, China. Int J Cancer 2006;118:2847-53. [Crossref] [PubMed]
- Burchard EG, Ziv E, Coyle N, et al. The importance of race and ethnic background in biomedical research and clinical practice. N Engl J Med 2003;348:1170-5. [Crossref] [PubMed]
- Fine MJ, Ibrahim SA, Thomas SB. The role of race and genetics in health disparities research. Am J Public Health 2005;95:2125-8. [Crossref] [PubMed]
- Dixit M, Choudhuri G, Mittal B. Association of lipoprotein receptor, receptor-associated protein, and metabolizing enzyme gene polymorphisms with gallstone disease: A case-control study. Hepatol Res 2006;36:61-9. [Crossref] [PubMed]
- Hofman MK, Weggemans RM, Zock PL, et al. CYP7A1 A-278C polymorphism affects the response of plasma lipids after dietary cholesterol or cafestol interventions in humans. J Nutr 2004;134:2200-4. [PubMed]
- Srivastava A, Pandey SN, Choudhuri G, et al. Role of genetic variant A-204C of cholesterol 7alpha-hydroxylase (CYP7A1) in susceptibility to gallbladder cancer. Mol Genet Metab 2008;94:83-9. [Crossref] [PubMed]
- Andreotti G, Chen J, Gao YT, et al. Polymorphisms of genes in the lipid metabolism pathway and risk of biliary tract cancers and stones: a population-based case-control study in Shanghai, China. Cancer Epidemiol Biomarkers Prev 2008;17:525-34. [Crossref] [PubMed]
- Báez S, Tsuchiya Y, Calvo A, et al. Genetic variants involved in gallstone formation and capsaicin metabolism, and the risk of gallbladder cancer in Chilean women. World J Gastroenterol 2010;16:372-8. [Crossref] [PubMed]
- Kaur MP, Guggenheim EJ, Pulisciano C, et al. Cellular accumulation of Cys326-OGG1 protein complexes under conditions of oxidative stress. Biochem Biophys Res Commun 2014;447:12-8. [Crossref] [PubMed]
- Srivastava A, Srivastava K, Pandey SN, et al. Single-nucleotide polymorphisms of DNA repair genes OGG1 and XRCC1: association with gallbladder cancer in North Indian population. Ann Surg Oncol 2009;16:1695-703. [Crossref] [PubMed]
- Srivastava K, Srivastava A, Mittal B. Polymorphisms in ERCC2, MSH2, and OGG1 DNA repair genes and gallbladder cancer risk in a population of Northern India. Cancer 2010;116:3160-9. [Crossref] [PubMed]
- Ding X, Wang K, Wu Z, et al. The Ser326Cys polymorphism of hOGG1 is associated with intrahepatic cholangiocarcinoma susceptibility in a Chinese population. Int J Clin Exp Med 2015;8:16294-300. [PubMed]
- Jiao X, Huang J, Wu S, et al. hOGG1 Ser326Cys polymorphism and susceptibility to gallbladder cancer in a Chinese population. Int J Cancer 2007;121:501-5. [Crossref] [PubMed]
- You SH, Wang X, Huang S, et al. MYH rs3219476 and rs3219472 polymorphisms and risk of cholangiocarcinoma. Mol Med Rep 2013;7:347-51. [PubMed]
- Vishnoi M, Pandey SN, Modi DR, et al. Genetic susceptibility of epidermal growth factor +61A>G and transforming growth factor beta1 -509C>T gene polymorphisms with gallbladder cancer. Hum Immunol 2008;69:360-7. [Crossref] [PubMed]
- Hsing AW, Sakoda LC, Rashid A, et al. Variants in inflammation genes and the risk of biliary tract cancers and stones: a population-based study in China. Cancer Res 2008;68:6442-52. [Crossref] [PubMed]
- Mishra K, Behari A, Kapoor VK, et al. Vascular endothelial growth factor single-nucleotide polymorphism in gallbladder cancer. J Gastroenterol Hepatol 2013;28:1678-85. [PubMed]
- Rai R, Sharma KL, Tiwari S, et al. DCC (deleted in colorectal carcinoma) gene variants confer increased susceptibility to gallbladder cancer (Ref. No.: Gene-D-12-01446). Gene 2013;518:303-9. [Crossref] [PubMed]
- Chaiteerakij R, Juran BD, Aboelsoud MM, et al. Association between variants in inflammation and cancer-associated genes and risk and survival of cholangiocarcinoma. Cancer Med 2015;4:1599-602. [Crossref] [PubMed]
- Sakoda LC, Gao YT, Chen BE, et al. Prostaglandin-endoperoxide synthase 2 (PTGS2) gene polymorphisms and risk of biliary tract cancer and gallstones: a population-based study in Shanghai, China. Carcinogenesis 2006;27:1251-6. [Crossref] [PubMed]
- Guo Y, Xu F, Lu T, et al. Interleukin-6 signaling pathway in targeted therapy for cancer. Cancer Treat Rev 2012;38:904-10. [Crossref] [PubMed]
- Prayong P, Mairiang E, Pairojkul C, et al. An interleukin-6 receptor polymorphism is associated with opisthorchiasis-linked cholangiocarcinoma risk in Thailand. Asian Pac J Cancer Prev 2014;15:5443-7. [Crossref] [PubMed]
- Castro FA, Koshiol J, Hsing AW, et al. Inflammatory gene variants and the risk of biliary tract cancers and stones: a population-based study in China. BMC Cancer 2012;12:468. [Crossref] [PubMed]
- Vishnoi M, Pandey SN, Choudhuri G, et al. IL-1 gene polymorphisms and genetic susceptibility of gallbladder cancer in a north Indian population. Cancer Genet Cytogenet 2008;186:63-8. [Crossref] [PubMed]
- Spear P, Wu MR, Sentman ML, et al. NKG2D ligands as therapeutic targets. Cancer Immun 2013;13:8. [PubMed]
- Melum E, Karlsen TH, Schrumpf E, et al. Cholangiocarcinoma in primary sclerosing cholangitis is associated with NKG2D polymorphisms. Hepatology 2008;47:90-6. [Crossref] [PubMed]
- Krawczyk M, Höblinger A, Mihalache F, et al. Macrophage stimulating protein variation enhances the risk of sporadic extrahepatic cholangiocarcinoma. Dig Liver Dis 2013;45:612-5. [Crossref] [PubMed]
- Kawaguchi M, Kataoka H. MST1 (macrophage stimulating 1 (hepatocyte growth factor-like)). Available online: http://atlasgeneticsoncology.org/Genes/MST1ID44411ch3p21.html
- Shaik AP, Shaik AS, Al-Sheikh YA, et al. Colorectal cancer: A review of the genome-wide association studies in the kingdom of Saudi Arabia. Saudi J Gastroenterol 2015;21:123-8. [Crossref] [PubMed]
- Gorrell MD. Liver fibrosis: the hepatocyte revisited. Hepatology 2007;46:1659-61. [Crossref] [PubMed]