Stepwise analysis of thyroid diagnostic modalities with genomic imprinting detection
Highlight box
Key findings
• Given the preliminary thyroid malignancy discrimination obtained from ultrasonography [area under the receiver operating characteristics curve (AUROC): 0.79], improvements in diagnostic contributions were significant when thyroid function serology (AUROC: 0.82) and FNA cytopathology (AUROC: 0.88) were stepwise added. Including quantitative chromogenic imprinted gene in-situ hybridization (QCIGISH) as an adjunctive molecular test for the standard clinical assessments further enhanced the preceding model’s diagnostic performance (AUROC: 0.95).
What is known and what is new?
• Recent studies have highlighted how genomic imprinting biomarkers through QCIGISH significantly advanced early thyroid cancer detection by identifying subtle molecular changes associated with carcinogenesis.
• This research demonstrated the stepwise diagnostic contribution of QCIGISH to standard modalities in malignancy risk stratification of thyroid nodules which has not been previously explored.
What is the implication, and what should change now?
• A collective clinical malignancy risk assessment using QCIGISH in addition to standard diagnostic modalities may prove useful for improving the overall preoperative evaluation of thyroid nodules, and their subsequent therapeutic management.
Introduction
Thyroid nodules are prevalent in the general adult population (1), but the vast majority of these nodules represent benign lesions with only 5% clinically diagnosed as malignant (2). Although most thyroid cancers tend to behave more indolently with a relatively slower development, certain cases will subsequently become invasive and metastasize (3). Therefore, an accurate preoperative diagnosis of biologically significant cancers to enable timely clinical intervention for high-risk patients, while avoiding unnecessary diagnostic testing and potential thyroid surgery in low-risk individuals with benign diseases, is particularly essential. To manage the risk assessment for thyroid nodules, a combination of different post-clinical evaluation diagnostic modalities including ultrasonography, thyroid function serology, fine-needle aspiration (FNA) cytopathology and supplementary molecular tests are recommended by current clinical guidelines (4-6).
As a non-invasive approach for the initial clinical assessment of thyroid nodules, several ultrasonographic reporting systems have been proposed, among which, the American College of Radiology Thyroid Imaging, Reporting and Data System (ACR TI-RADS) is the most widely used (7). According to certain morphological characteristics including solid composition, hypoechogenicity, microcalcification, nonparallel orientation and irregular margins, ACR TI-RADS classify thyroid nodules into five categories with an increasing risk of malignancy: 1, benign; 2, not suspicious; 3, mildly suspicious; 4, moderately suspicious; and 5, highly suspicious (7). Suspicious nodules, with sizes above the specific criteria defined individually for categories 3 to 5, are recommended for FNA for a more definitive diagnosis (4,5).
As an endocrine gland, the aberrant serum levels of thyroid secreted hormones such as tri-iodothyronine (T3) and thyroxine (T4) can provide clinical insights into diagnosing thyroid disorders (8,9). The relationships between elevated levels of serum free thyroxine (FT4) with increased risks of thyroid malignancy have been well documented (10-12). Inconsistent free T3 (FT3) levels have also been reported in thyroid cancers (13-16). Although, certain studies have shown that increased FT4/FT3 ratio could be a more reliable malignancy predictor for thyroid nodules (17-19). Despite being important risk factors for malignancy, the FT4/FT3 ratio results do not provide a conclusive diagnosis for thyroid cancer.
FNA cytopathology uses morphological evidences for the preoperative diagnosis of thyroid nodules. Under the Bethesda classification system, thyroid nodules are classified into six categories with an increasing risk of malignancy: Bethesda I, non-diagnostic or unsatisfactory; Bethesda II, benign; Bethesda III, atypia of undetermined significance or follicular lesion of undetermined significance; Bethesda IV, follicular neoplasm or suspicious for a follicular neoplasm; Bethesda V, suspicious for malignancy; and Bethesda VI, malignant (20). The Bethesda III to V categories, comprising approximately 20–30% of thyroid nodules, are considered indeterminate predominantly due to unclear morphology (21,22). The clinical dilemma from inconclusive cytopathological diagnoses can be further aggravated by the potentially inconsistent results from earlier diagnostic steps including suspicious findings from prior ultrasound or thyroid function tests. For these atypical and suspicious results from cytopathology, patients with truly benign thyroid nodules are potentially subjected to unwarranted surgical interventions with lifelong repercussions, while patients with truly malignant thyroid nodules might remain undiagnosed thereby increasing the risk for distant metastasis.
To address such clinical predicament, the American Thyroid Association has suggested the molecular profiling of thyroid nodules with indeterminate cytopathology as supplement diagnostic methods (23), including BRAF V600E mutation (24,25), ThyroseqTM gene sequencing (26,27), Afirma GEC or GSCTM gene expression classifier (28) and mir-THYtypeTM microRNA (miRNA) classifier (29). In addition to these molecular tests, the quantitative chromogenic imprinted gene in-situ hybridization (QCIGISH) methodology has previously been reported to directly visualize and quantitatively assess epigenetic imprinting alterations in clinical specimens obtained from ten cancer types, including thyroid cancer (30). Genomic imprinting is an epigenetic regulatory mechanism that controls the single-allelic expression of imprinted genes via allele-specific methylation and long non-coding RNAs (lncRNAs) (31,32). Regular genomic imprinting plays an important role in mammalian embryo development, while aberrant expressions of imprinted genes are related to tumorigenesis (32,33). Through this in-situ hybridization (ISH)-based approach, the expression sites of imprinted genes have been visualized and the aberrant expressions quantified including their biallelic, multiallelic and total expressions. The previous research demonstrated the differential epigenetic imprinting signatures for cancers, predominantly observed with elevated aberrant allelic expressions, which were significantly different as compared with those obtained from benign lesions and normal controls. As a molecular profiling test based on epigenetic imprinting alterations which precede morphological changes, QCIGISH could provide clearer malignancy differentiation for thyroid nodules (30,34,35). However, validation studies are necessary to independently evaluate the diagnostic contribution of QCIGISH as an adjunctive molecular test in further improving the capabilities of existing clinical assessments for stratifying malignancy risks in thyroid nodules (36).
In this study, we aimed to substantiate the stepwise contributions of each diagnostic modality, namely ultrasonography, thyroid function serology and FNA cytopathology in thyroid malignancy prediction as similarly explored in literature (37,38). However, as molecular testing of FNA biopsy specimens continue to gain acceptance as a substantial advancement in the accurate clinical diagnosis of indeterminate thyroid nodules (39-41), we also investigated the potential diagnostic significance of QCIGISH, a molecular test based on epigenetic imprinting alterations previously reported as efficient cancer biomarkers for the diagnosis of morphologically indeterminate thyroid nodules, adjunctive to standard clinical diagnostic procedures. Using a cohort of surgical patients, we formulated a univariate baseline model using the ultrasonographic results as predictor, being the first diagnostic step for risk-stratifying thyroid malignancy. All other subsequent diagnostic factors were individually and sequentially considered—thyroid function serology, FNA cytopathology and molecular imprinting detection through QCIGISH, respectively, into the multivariate logistic regression model with the objective of evaluating the additive contribution of each diagnostic step in the ability to accurately predict thyroid cancer. We present this article in accordance with the STROBE reporting checklist (available at https://cco.amegroups.com/article/view/10.21037/cco-23-89/rc).
Methods
Patients
Patients with thyroid nodules detected from ultrasound examination who were recommended for fine-needle aspiration biopsy (FNAB), driven by suspicious findings from ultrasonography, nodule growth, elevated clinical risk factors or patient preference, were consecutively recruited from Taizhou People’s Hospital, Taizhou Third People’s Hospital, Shanghai Tenth People’s Hospital of Tongji University School of Medicine and Jiangyuan Hospital Affiliated to Jiangsu Institute of Nuclear Medicine between May 21, 2019 and Jan 5, 2021 following a defined list of study inclusion and exclusion criteria (Appendix 1, Figure 1). Patients with complete clinical, ultrasonographic and serological diagnostic information gathered retrospectively; and preoperative cytopathologic, QCIGISH detection and postsurgical histopathologic evaluation results obtained prospectively following the procedures described under Appendix 1 and Figure S1 were included in the analysis. Histopathological diagnoses were reviewed by three independent pathologists (Rulong Shen, Wenbin Huang and Hongyu Yu). The study was conducted in accordance with the Declaration of Helsinki (as revised in 2013). The study was approved by the Ethics Committees of Taizhou People’s Hospital and Taizhou Third People’s Hospital (approval No. TZ20190520), Shanghai Tenth People’s Hospital (approval No. SHSY-IEC-4.1/19-6/01) and Jiangyuan Hospital Affiliated to Jiangsu Institute of Nuclear Medicine (approval No. YL201811). Informed consent was obtained from all individual participants.
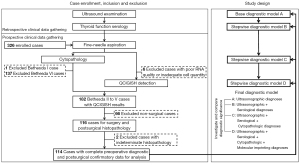
Sample size calculation applied the equation used for evaluating diagnostic accuracy studies involving binormal receiver operating characteristics (ROC) curve indices with ordinal discrete categories as response criterion (42). Under an assumption of a 75% ratio between the negative and positive groups, a minimum sample size of 35 from the positive group and 26 from the negative group achieves 81% power to detect a difference of at least 0.25, 0.20 and 0.15 between the AUROC values under the null hypothesis of 0.70, 0.75 and 0.80 (hypothesized for the ultrasonographic, serological or cytopathologic assessments considered as existing standards) and an AUROC under the alternative hypothesis of 0.95 (hypothesized for the imprinting assessment considered as the new test under evaluation) using a two-sided Z-test at a significance level of 0.05. Accounting for a 20% dropout rate, at least 75 cases will be gathered for the study. The sample size calculation was estimated using PASS V.21.0.3 (NCSS, Kaysville, Utah, USA).
Stepwise diagnostic model development and validation
Factors representing the diagnostic steps identified in the study, namely ultrasonography, thyroid function serology, FNA cytopathology and molecular imprinting detection through QCIGISH were preprocessed and transformed accordingly, as described under Supplement: Materials and Methods. Serving as model predictors, all were initially entered into a univariate analysis to assess their potential association towards thyroid malignancy a priori. The endpoint of interest was the postsurgically determined histopathological diagnosis for each nodule. Using a baseline model involving the ultrasonographic factor, other diagnostic factors including thyroid function serology, FNA cytopathology and molecular imprinting detection were then sequentially included in subsequent multivariate logistic regression models to evaluate the odds of developing thyroid malignancy. The area under the receiver operating characteristics curve (AUROC) and various goodness-of-fit assessment measures were determined to evaluate if the addition of each diagnostic step to the prior model resulted in a statistically significant improvement in thyroid malignancy discrimination and model fit, respectively.
Statistical analysis
Continuous variables such as age and thyroid function serological test measurements were reported as medians with interquartile ranges (IQR). Frequencies and proportions were used to describe categorical variables including sex and the various malignancy risk categories for ultrasonography, thyroid function serology, FNA cytopathology and molecular imprinting detection. Driven by the non-normal distributions determined using the Shapiro-Wilk test (43), differences between the benign and malignant groups for continuous clinical variables were evaluated using the Mann-Whitney U test. Categorical clinical variables for the benign and malignant cases were analyzed and compared using Chi-squared or Fisher exact tests, as applicable.
Odds ratios and their 95% confidence intervals (CIs) were reported for the univariate and multivariate logistic regression models. In analyzing the diagnostic contributions of each individual predictive factor in the stepwise regression models, likelihood ratio test was performed to measure the drop in residual deviance for the current model against the prior model, with the hypothesized significance evaluated using Chi-squared test. Akaike information criterion (AIC) and McFadden’s R2 (44) were used as additional model goodness-of-fit measures. The variance inflation factor (VIF) metric (45) was used to assess for potential multicollinearity among the predictor variables in the diagnostic models.
The discrimination performance of the diagnostic models was assessed using the AUROC metric with 95% CI determined using the DeLong method. Optimism-adjusted AUROC values were estimated to correct against potential overfitting using a 500-cycle internal bootstrap validation method (46).
All hypothesis tests conducted were two-sided, with computed P<0.05 considered to be statistically significant. All statistical analyses and visualizations were performed using R software (version 3.5.0) (47).
Results
Patient characteristics
A total of 326 consecutive cases of thyroid nodules with complete medical records for clinical, ultrasonographic and thyroid function serological examinations which were assessed for FNA cytopathology were recruited for the study. Each thyroid FNA specimen was divided for simultaneous cytopathology evaluation and blinded QCIGISH testing. From this initial number, 212 cases were subsequently excluded including 1 Bethesda I case, 137 Bethesda VI cases, 6 with poor RNA quality or inadequate cell quantity for QCIGISH testing, 66 non-surgical cases and 2 with indeterminate cytopathology—with details illustrated under Figure 1. The remaining 114 thyroid nodules (34.97%) which had valid and complete results in FNA cytopathology, molecular imprinting detection and thyroid postsurgical histopathology were considered eligible for the analysis. 69.29% (79/114) of the cases were evaluated as cytopathologically indeterminate which were classified under Bethesda III to V, with the remaining 30.70% (35/114) having been risk-stratified as benign under Bethesda II. The clinicopathological characteristics of the patient cohort are summarized in Tables 1,2 with supplementary exploratory analysis results provided in Figures S2,S3.
Table 1
Patient information | Overall (n=114) | Benign (n=50) | Malignant (n=64) | P |
---|---|---|---|---|
Clinical characteristics | ||||
Sex, n (%) | 0.30† | |||
Male | 33 (28.95) | 12 (24.00) | 21 (32.81) | |
Female | 81 (71.05) | 38 (76.00) | 43 (67.19) | |
Age (years), median [IQR] | 50 [36–62] | 58 [47–64] | 41 [33–52] | <0.001‡ |
Ultrasonographic assessment | ||||
ACR TI-RADS, n (%) | <0.001† | |||
Category 2 | 14 (12.28) | 13 (26.00) | 1 (1.56) | |
Category 3 | 20 (17.54) | 18 (36.00) | 2 (3.12) | |
Category 4 | 22 (19.30) | 12 (24.00) | 10 (15.63) | |
Category 5 | 58 (50.88) | 7 (14.00) | 51 (79.69) | |
Serological assessment | ||||
FT4 (pmol/L), median [IQR] | 16.7 [15.4–18.6] | 15.9 [14.7–17.2] | 17.3 [16.2–19.7] | <0.001‡ |
FT3 (pmol/L), median [IQR] | 5.1 [4.6–5.5] | 5.0 [4.6–5.4] | 5.1 [4.6–5.6] | 0.40‡ |
FT4/FT3 ratio, median [IQR] | 3.3 [3.0–3.7] | 3.2 [2.9–3.5] | 3.5 [3.1–3.8] | 0.01‡ |
Cytopathologic assessment | ||||
Bethesda classification, n (%) | <0.001† | |||
Category II | 35 (30.70) | 33 (66.00) | 2 (3.12) | |
Category III | 24 (21.05) | 10 (20.00) | 14 (21.88) | |
Category IV | 11 (9.65) | 3 (6.00) | 8 (12.50) | |
Category V | 44 (38.60) | 4 (8.00) | 40 (62.50) | |
Imprinting assessment | ||||
QCIGISH classification, n (%) | <0.001† | |||
Grade 0 | 20 (17.54) | 20 (40.00) | 0 | |
Grade I | 24 (21.05) | 23 (46.00) | 1 (1.56) | |
Grade II | 8 (7.02) | 2 (4.00) | 6 (9.38) | |
Grade III | 40 (35.09) | 5 (10.00) | 35 (54.69) | |
Grade IV | 22 (19.30) | 0 | 22 (34.38) | |
Postsurgical histopathologic diagnosis | <0.001† | |||
Benign, n (%) | ||||
Nodular goiter | 27 (23.68) | 27 (54.00) | ||
Follicular thyroid adenoma | 7 (6.14) | 7 (14.00) | ||
Adenomatous goiter | 6 (5.26) | 6 (12.00) | ||
Hashimoto’s thyroiditis | 5 (4.39) | 5 (10.00) | ||
Adenoma | 2 (1.75) | 2 (4.00) | ||
Thyroiditis | 2 (1.75) | 2 (4.00) | ||
Goiter with adenoma | 1 (0.88) | 1 (2.00) | ||
Malignant, n (%) | ||||
Papillary thyroid carcinoma | 47 (41.23) | 47 (73.44) | ||
Papillary thyroid microcarcinoma | 12 (10.53) | 12 (18.75) | ||
Follicular thyroid carcinoma | 3 (2.63) | 3 (4.69) | ||
Medullary thyroid carcinoma | 1 (0.88) | 1 (1.56) | ||
Papillary/follicular thyroid carcinoma | 1 (0.88) | 1 (1.56) |
Baseline clinical characteristics and diagnostic information of the patient cohort (n=114). †, categorical variables were compared using Chi-squared or Fisher’s exact tests, as applicable. ‡, continuous variables were compared between the benign and malignant cases using the Mann-Whitney U test. All hypothesis tests applied were two-tailed. Computed P values of less than 0.05 were considered as significant. IQR, interquartile ranges; FT4, free thyroxine; FT3, free triiodothyronine; ACR TI-RADS, American College of Radiology Thyroid Imaging, Reporting and Data System; QCIGISH, quantitative chromogenic imprinted gene in-situ hybridization.
Table 2
Diagnostic modality | Overall (n=114) | Benign (n=50) | Malignant (n=64) | P |
---|---|---|---|---|
Ultrasonographic assessment | ||||
ACR TI-RADS, n (%) | <0.001†,‡ | |||
Categories 2, 3 | 34 (29.82) | 31 (62.00) | 3 (4.69) | |
Categories 4, 5 | 80 (70.18) | 19 (38.00) | 61 (95.31) | |
Serological assessment | ||||
FT4/FT3 level, n (%) | 0.02†,§ | |||
Low | 54 (47.37) | 30 (60.00) | 24 (37.50) | |
High | 60 (52.63) | 20 (40.00) | 40 (62.50) | |
Cytopathologic assessment | ||||
Bethesda classification, n (%) | <0.001†,¶ | |||
Categories II, III, IV | 70 (61.40) | 46 (92.00) | 24 (37.50) | |
Category V | 44 (38.60) | 4 (8.00) | 40 (62.50) | |
Imprinting assessment | ||||
QCIGISH classification, n (%) | <0.001†,†† | |||
Grades 0, I | 44 (38.60) | 43 (86.00) | 1 (1.56) | |
Grades II, III, IV | 70 (61.40) | 7 (14.00) | 63 (98.44) |
Proportions of benign and malignant cases across the different diagnostic modalities evaluated for the patient cohort (n=114). †, categorical variables were compared using Chi-squared or Fisher’s exact tests, as applicable. Computed P values of less than 0.05 were considered as significant. ‡, ACR TI-RADS categories were combined prior to hypothesis testing (combined Categories 2 and 3 were tested against combined Categories 4 and 5). §, FT4/FT3 ratio values were dichotomized into low (≤3.3) and high (>3.3) levels. ¶, Bethesda categories were combined prior to hypothesis testing (combined Categories II, III and IV were tested against Category V). ††, QCIGISH grades were combined prior to hypothesis testing (combined Grades 0 and I were tested against combined Grades III, IV and V). FT4, free thyroxine; FT3, free triiodothyronine; ACR TI-RADS, American College of Radiology Thyroid Imaging, Reporting and Data System; QCIGISH, quantitative chromogenic imprinted gene in-situ hybridization.
The median age at diagnosis was 50 years (IQR, 36–62 years) with female predominance (71.05% vs. 28.95%; female to male ratio, 2.5:1). Overall, 50 patients were diagnosed with histopathologically benign nodules [including 27 nodular goiter (NG), 7 follicular thyroid adenoma (FTA), 6 adenomatous goiter, 5 Hashimoto’s thyroiditis (HT), 2 adenoma, 2 thyroiditis and 1 goiter with adenoma], and 64 patients were diagnosed with thyroid cancer [including 47 papillary thyroid carcinoma (PTC), 12 papillary thyroid microcarcinoma (PTMC), 3 follicular thyroid carcinoma (FTC), 1 medullary thyroid carcinoma (MTC) and 1 combined PTC and FTC]. A malignancy rate of 56.14% was noted from the study.
Malignancy rates across ultrasonographic, serological, cytopathologic and imprinting assessments for thyroid malignancy risk evaluation
Increasing malignancy rates were observed across the transformed binary categorical predictors for the ultrasonographic, serological, cytopathologic and imprinting factors prior to logistic regression model development (Table 1, Figure S2). 8.82% (3/34) of the combined ACR TI-RADS categories 2 and 3 were confirmed malignant, while that determined for the combined categories 4 and 5 was 76.25% (61/80). Malignancy rates for the low and high FT4/FT3 ratio levels were 44.44% (24/54) and 66.67% (40/60), respectively. Increasing proportions of histopathologically malignant cases were similarly observed for the combined Bethesda categories II, III and IV at 34.28% (24/70) and Bethesda category V at 90.91% (40/44). QCIGISH classification demonstrated malignancy rates of 2.27% (1/44) for combined Grades 0 and I, and 90.00% (63/70) for combined Grades II, III and IV. Since these grades already represent the actual QCIGISH-positive and QCIGISH-negative categories, diagnostic performance estimates were determined as follows: 98.44% sensitivity (95% CI: 95.40–100.00%), 86.00% specificity (95% CI: 76.38–95.62%), 90.00% positive predictive value (PPV) (95% CI: 82.97–97.03%) and 97.72% negative predictive value (NPV) (95% CI: 93.32–100.00%).
Additive stepwise contributions of ultrasonographic, serological, cytopathologic and imprinting assessments for thyroid malignancy risk evaluation
Univariate logistic regression applied on the clinical diagnostic evaluation factors showed that imprinting (AUROC: 0.92; 95% CI: 0.87–0.97; optimism-adjusted AUROC: 0.91), cytopathologic (AUROC: 0.77; 95% CI: 0.70–0.84); optimism-adjusted AUROC: 0.76) and ultrasonographic (AUROC: 0.79; 95% CI: 0.71–0.86; optimism-adjusted AUROC: 0.78) factors demonstrated superior discrimination performance as compared to the serological (AUROC: 0.61; 95% CI: 0.52–0.70; optimism-adjusted AUROC: 0.60) factor (P<0.001 to P=0.003) (Table 2 and Table S1, Figure 2). All modalities were however independent predictors of thyroid malignancy with statistically significant odds ratios as determined for the ultrasonographic [odds ratio (OR): 33.18; 95% CI: 10.42–149.44; P<0.001], serological (OR: 2.50; 95% CI: 1.18–5.41; P=0.02), cytopathologic (OR: 19.17; 95% CI: 6.76–69.58; P<0.001) and imprinting (OR: 387.00; 95% CI: 68.66–7,413.33; P<0.001) factors (Table 3).
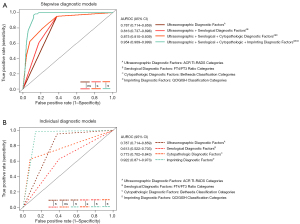
Table 3
Diagnostic modality | Reference category | Univariate analysis (n=114) | Stepwise multivariate analysis (n=114) | ||||||||||||
---|---|---|---|---|---|---|---|---|---|---|---|---|---|---|---|
Model 1‡ | Model A§ | Model B¶ | Model C†† | Model D‡‡ | |||||||||||
OR (95% CI) | P† | OR (95% CI) | P† | OR (95% CI) | P† | OR (95% CI) | P† | OR (95% CI) | P† | ||||||
Ultrasonographic assessment | |||||||||||||||
ACR TI-RADS | |||||||||||||||
Categories 4, 5 | Categories 2, 3 | 33.18 (10.42–149.44) | <0.001 | 33.18 (10.42–149.44) | <0.001 | 30.37 (9.45–137.43) | <0.001 | 13.97 (4.03–66.23) | <0.001 | 2.45 (0.25–20.21) | 0.40 | ||||
Serological assessment | |||||||||||||||
FT4/FT3 ratio | |||||||||||||||
High (>3.3) | Low (≤3.3) | 2.50 (1.18–5.41) | 0.02 | – | – | 1.75 (0.67–4.57) | 0.25 | 1.38 (0.48–3.83) | 0.54 | 0.74 (0.13–3.50) | 0.72 | ||||
Cytopathologic assessment | |||||||||||||||
Bethesda classification | |||||||||||||||
Category V | Categories II, III, IV | 19.17 (6.76–69.58) | <0.001 | – | – | – | – | 6.79 (2.13–26.43) | 0.002 | 6.13 (1.09–52.24) | 0.06 | ||||
Imprinting assessment | |||||||||||||||
QCIGISH classification | |||||||||||||||
Grades II, III, IV | Grades 0, I | 387.00 (68.66–7,413.33) | <0.001 | – | – | – | – | – | – | 195.45 (29.63–4,098.60) | <0.001 |
Univariate and stepwise multivariate logistic regression of malignancies in thyroid nodules using ultrasonographic, serological, cytopathologic and imprinting assessments. †, Wald’s test was applied to test the significance of the individual regression coefficients. ‡, univariate logistic regression was developed separately using ultrasonographic, serological, cytopathologic, and imprinting assessments. §, base univariate logistic regression was developed using ultrasonographic assessment. ¶, stepwise multivariate logistic regression was developed using ultrasonographic and serological assessments. ††, stepwise multivariate logistic regression was developed using ultrasonographic, serological, and cytopathologic assessments. ‡‡, stepwise multivariate logistic regression was developed using ultrasonographic, serological, cytopathologic, and imprinting assessments. OR, odds ratio; CI, confidence interval; FT4, free thyroxine; FT3, free triiodothyronine; ACR TI-RADS, American College of Radiology Thyroid Imaging, Reporting and Data System; QCIGISH, quantitative chromogenic imprinted gene in-situ hybridization.
With the ultrasonographic factor used as the baseline model [AUROC: 0.79 (95% CI: 0.71–0.86), optimism-adjusted AUROC: 0.78], multivariate logistic regression for predicting thyroid malignancy was formulated and stepwise regressed on the serological, cytopathologic and imprinting factors, respectively, reflecting the actual sequential clinical utility of these diagnostic evaluations (Table 3). Each added factor improved the stepwise model’s AUROC to 0.82 (95% CI: 0.74–0.90; P=0.23; optimism-adjusted AUROC: 0.80), 0.88 (95% CI: 0.81–0.94; P=0.02; optimism-adjusted AUROC: 0.86) and 0.95 (95% CI: 0.91–1.00; P=0.007; optimism-adjusted AUROC: 0.93), respectively (Table S1, Figure 2).
Different sets of factors were determined as independent predictors of thyroid malignancy for each stepwise regression implemented in the study as shown on Table 3. However, for the final model which combined all four factors simultaneously into the model, only imprinting remained statistically significant (OR: 195.45; 95% CI: 29.63–4,098.60; P<0.001).
Goodness-of-fit measures applied on all four stepwise models showed generally decreasing AIC (112.00, 112.69, 103.63 and 58.54, respectively) and increasing McFadden’s R2 (0.31, 0.32, 0.39 and 0.69, respectively) with the best fit identified for the final model (Table 4).
Table 4
Diagnostic model | AIC†† | McFadden’s R2‡‡ | Residual deviance | LRT§§ | P¶¶ |
---|---|---|---|---|---|
Ultrasonographic† | 112.00 | 0.31 | 108.00 | NA | NA |
Ultrasonographic† + serological‡ | 112.69 | 0.32 | 106.69 | 1.31 | 0.25 |
Ultrasonographic† + serological‡ + cytopathologic§ | 103.63 | 0.39 | 95.63 | 11.06 | <0.001 |
Ultrasonographic† + serological‡ + cytopathologic§ + imprinting¶ | 58.54 | 0.69 | 48.54 | 47.09 | <0.001 |
Evaluation of the goodness of fit for the stepwise logistic regression models involving ultrasonographic, serological, cytopathologic and imprinting diagnoses. †, ACR TI-RADS categories used as ultrasonographic diagnostic factors. ‡, FT4/FT3 ratio categories used as serological diagnostic factors. §, Bethesda classification categories used as cytopathologic diagnostic factors. ¶, QCIGISH classification categories used as imprinting diagnostic factors. ††, lower computed AIC values indicate a better model fit. ‡‡, higher computed McFadden’s R2 values indicate a better model fit. §§, LRT values represent the drop in residual deviance for the current model with respect to the prior model. ¶¶, Chi-squared test was used to test the significance between the likelihood under the current model against the prior model. AIC, Akaike information criterion; LRT, likelihood ratio test; FT4, free thyroxine; FT3, free triiodothyronine; ACR TI-RADS, American College of Radiology Thyroid Imaging, Reporting and Data System; QCIGISH, quantitative chromogenic imprinted gene in-situ hybridization.
These findings demonstrated the significant stepwise diagnostic contributions of standard clinical assessments in the malignancy risk stratification of thyroid nodules. However, the addition of molecular imprinting detection may further enable a more accurate and definitive preoperative evaluation to improve clinical management.
Adoption of molecular imprinting detection findings into ultrasonographic, serological and cytopathologic results collectively improved thyroid malignancy risk assessment
To illustrate, four representative cases were presented on Figure 3. Case 1 involved moderately suspicious findings for thyroid cancer from ultrasound examination (ACR TI-RADS category 4) with normal serum FT4/FT3 ratio level and benign FNA cytopathology (Bethesda II)—representing discordant assessments without molecular imprinting detection. However, thyroid malignancy risk was identified for this patient based on imprinting signatures (QCIGISH-positive). With the imprinting diagnostic information added, this case had an increased malignancy risk, which was confirmed as PTC from postsurgical histopathology. Considering the preceding modalities which involved all diagnostic factors except imprinting might have indicated a lower malignancy risk.
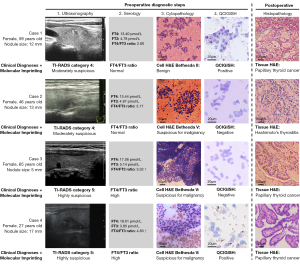
Case 2 was examined as moderately suspicious for thyroid cancer (ACR TI-RADS category 4) with normal serum FT4/FT3 ratio level but presurgically diagnosed as suspicious for malignancy (Bethesda V)—resulting in a high malignancy risk evaluation using only these factors in the multivariate logistic regression model. This patient was QCIGISH-negative who was identified with low malignancy risk based from molecular imprinting detection. Combining all these factors including the imprinting findings resulted to a low-risk assessment which was confirmed by postsurgical histopathology as HT. In the absence of the imprinting factor in the model, this particular case would have been mistakenly evaluated as potentially malignant.
Case 3’s findings were isolated which involved a patient with ACR TI-RADS category V under ultrasound examination (highly suspicious for thyroid cancer), elevated FT4/FT3 ratio levels and Bethesda V under FNA cytopathology (highly suspicious for thyroid cancer). QCIGISH detection was however negative. This case was confirmed PTC by surgical histopathology. Despite the discordant results from QCIGISH, the physicians were able to give a definitive malignant diagnosis based on the consistently high malignancy risk findings from ultrasonography, serology and cytopathology. For instance, when preoperative assessments using standard modalities remain coherent, the malignancy risk evaluation becomes more reliable making adjunctive molecular tests like QCIGISH optional.
Case 4 showed a patient with ACR TI-RADS category V under ultrasound examination (highly suspicious for thyroid cancer), elevated FT4/FT3 ratio levels, Bethesda V under FNA cytopathology (highly suspicious for thyroid cancer) and positive QCIGISH detection. Despite the concordant results from QCIGISH to the assessments made using ultrasonography, serology and cytopathology, the consistent preoperative evaluation using standard modalities were sufficient to stratify the malignancy risk for this case which may not require further confirmation from an adjunctive molecular test based on clinical guidelines.
Discussion
A thorough and accurate preoperative assessment of thyroid nodules which enables the ability to effectively distinguish between malignancy and benignity is critical in achieving the best therapeutic implications for patients. Despite the collective risk information gathered from standard thyroid diagnostic tests including ultrasonography, serology and FNA biopsy, the management of approximately 20–30% of thyroid nodules which are cytopathologically indeterminate with highly varying malignancy risks ranging from 5% to 75%, or cases with discordant preoperative assessment using standard diagnostic modalities, remains a clinical challenge (21,22). Avoiding overtreatment in low-risk patients while informing effective diagnostic interventions for high-risk patients often require incorporating additional risk factors into clinical decision-making including a more definitive guidance from molecular markers, among others (4,5). Molecular profiling, such as imprinting detection in particular, therefore has the potential to drive better clinical decisions in the setting of indeterminate thyroid nodules by guiding both the need for and extent of thyroid surgery.
In the present study, we particularly investigated a set of predominantly cytopathologically indeterminate thyroid nodules which continue to be a diagnostic challenge in terms of interpretation and clinical management. FNAB examination, which is solely based on morphological features, may be unable to optimally provide definitive diagnoses in most of these cases. We consolidated all related diagnostic information predictive of thyroid malignancy using the ultrasonographic, thyroid function serological, FNA cytopathologic and imprinting detection factors, which were all sequentially applied based in the particular order of clinical practice. With postsurgical histopathology applied as the diagnostic gold standard, multivariate regression models were formulated from a stepwise combination of all these predictive factors. Our results showed that each diagnostic step has additively contributed in the accurate prediction of thyroid malignancy. The final diagnostic model incorporating all factors demonstrated high discrimination power with an AUROC of 0.95. Although the inclusion of the ultrasonographic, serological and cytopathologic assessments have already significantly improved model performance, QCIGISH—an imprinting-based molecular test further elevated malignancy discrimination demonstrating its clinical value in effectively differentiating benign lesions from thyroid cancers, especially in indeterminate cases.
Thyroid cancer, being an indolent and slow progressing type of cancer, poses a different set of challenges during clinical management as both under-treatment and over-treatment have implications (4). Although a considerable number of protein biomarkers have been discovered to further improve the accuracy of diagnosing thyroid nodules (48-52), these markers are usually only detected through immunohistochemistry on tissue resections obtained postsurgically. For preoperative risk assessment, several molecular tests on FNA cytology samples have been developed, including BRAF V600E mutation which is specific for papillary thyroid cancer (24); RNA sequencing-based Afirma GSCTM gene expression classifier as a good rule-out test (28); and ThyroseqTM genomic classifier and mir-THYtypeTM miRNA classifiers for both rule-in and rule-out testing (27,29), despite several noted limitations. In PTCs which account for 80–85% of all thyroid cancers, the frequency of BRAF V600E mutation varies from 29% to 83% among different cohorts, sample sizes and detection methods (24,53-56). Considering other subtypes of thyroid cancer with rare BRAF V600E mutation, more than 30% of thyroid cancer patients who may not harbor BRAF V600E mutation will not be effectively diagnosed with malignancy using this particular molecular test. In addition, next generation sequencing methods including Afirma GSCTM, ThyroseqTM and mir-THYtypeTM, have yet to improve their technical feasibility (29) to optimize their continued clinical application.
Conceptually different from these aforementioned molecular tests, QCIGISH uses an epigenetics-based approach. Instead of detecting methylation and lncRNAs which regulate the expression of imprinted genes, a previously reported ISH-based methodology targeting the intronic regions of nascent RNAs was applied to detect the gene expression sites in the nuclei (30). Particularly using this experimental method, the allelic expression status of imprinted genes in individual cells can be visually assessed and quantified. Based from the imprinting signatures, a predictive model which effectively differentiated thyroid malignancy from benign lesions using five grades (Grades 0, I, II, III and IV) was previously developed with increasing malignancy risks (30). The QCIGISH grades were subsequently grouped into two categories to facilitate diagnostic performance assessment—QCIGISH-negative (Grades 0 and I) and QCIGISH-positive (Grades II, III and IV), representing benign and malignant predictions, respectively. As epigenetic alterations usually occur at early stages of carcinogenesis and precede cellular morphological changes (57), QCIGISH showed excellent diagnostic sensitivity and specificity from a previous study (35). In an independent prospective validation, QCIGISH detection demonstrated 100.00% sensitivity, 91.45% specificity, 100.00% NPV and 96.52% PPV for presurgical FNA specimens (35). Particularly for the Bethesda III, IV and V cases with indeterminate FNA cytopathology, QCIGISH also showed high NPV of 100.00%, PPV of 96.55% and overall accuracy 97.53% (35). With high NPV and PPV, QCIGISH can be used as an excellent rule-in and rule-out diagnostic tool and could help the diagnosis of the indeterminate nodules.
From the stepwise model developed in this study, we accumulatively added the QCIGISH classification categories with the results of prior standard clinical diagnostic evaluations for thyroid nodules. QCIGISH can provide an additional risk stratification method with high diagnostic accuracy to supplement standard diagnostic modalities in thyroid clinical assessment. As an adjunctive molecular test, QCIGISH will be most helpful in further evaluating the likelihood of malignancy particularly for cases with either an indeterminate cytopathology or had discordant assessment results among different modalities which commonly cause a diagnostic dilemma for physicians during clinical management. A collective clinical malignancy risk assessment using QCIGISH in addition to standard diagnostic modalities including ultrasonography, serology and cytopathology may prove useful for improving the overall preoperative evaluation of thyroid nodules, and their subsequent therapeutic management. Powered by ISH-based technology, QCIGISH also presents an accurate, functional and immediately feasible thyroid molecular diagnostic solution for clinical applications as compared to sequencing-based methods.
We recognize that this study had several limitations. The study was limited by a small sampling of patients who have chosen to undergo thyroid surgery in a major referral cancer center. The inclusion of a purely surgical cohort introduces a selection bias as reflected in the high malignancy rate of 56.14% in the study. This likely limits the use of our results within a community setting with a potentially lesser proportion of malignant cases. Additional insights and a more conclusive validation on the utility of the study could also be obtained from a prospective large-scale evaluation involving more medical centers and higher patient case numbers with more diverse clinical characteristics and disease subtypes.
Conclusions
Our study demonstrated the significant stepwise diagnostic contributions of ultrasonography, thyroid function serology and FNA cytopathology in the malignancy risk assessment of thyroid nodules. However, the addition of molecular imprinting detection through QCIGISH complemented and further improved the collective diagnostic contributions obtained from standard clinical risk-assessment procedures, further enabling a more accurate and definitive preoperative evaluation and medical management, especially for morphologically indeterminate thyroid nodules and cases with potentially discordant results among standard modalities.
Acknowledgments
Funding: This work was supported by
Footnote
Reporting Checklist: The authors have completed the STROBE reporting checklist. Available at https://cco.amegroups.com/article/view/10.21037/cco-23-89/rc
Data Sharing Statement: Available at https://cco.amegroups.com/article/view/10.21037/cco-23-89/dss
Peer Review File: Available at https://cco.amegroups.com/article/view/10.21037/cco-23-89/prf
Conflicts of Interest: All authors have completed the ICMJE uniform disclosure form (available at https://cco.amegroups.com/article/view/10.21037/cco-23-89/coif). The authors have no conflicts of interest to declare.
Ethical Statement:
Open Access Statement: This is an Open Access article distributed in accordance with the Creative Commons Attribution-NonCommercial-NoDerivs 4.0 International License (CC BY-NC-ND 4.0), which permits the non-commercial replication and distribution of the article with the strict proviso that no changes or edits are made and the original work is properly cited (including links to both the formal publication through the relevant DOI and the license). See: https://creativecommons.org/licenses/by-nc-nd/4.0/.
References
- Guth S, Theune U, Aberle J, et al. Very high prevalence of thyroid nodules detected by high frequency (13 MHz) ultrasound examination. Eur J Clin Invest 2009;39:699-706. [Crossref] [PubMed]
- Yeung MJ, Serpell JW. Management of the solitary thyroid nodule. Oncologist 2008;13:105-12. [Crossref] [PubMed]
- Ambrosi F, Righi A, Ricci C, et al. Hobnail Variant of Papillary Thyroid Carcinoma: a Literature Review. Endocr Pathol 2017;28:293-301. [Crossref] [PubMed]
- Haugen BR, Alexander EK, Bible KC, et al. 2015 American Thyroid Association Management Guidelines for Adult Patients with Thyroid Nodules and Differentiated Thyroid Cancer: The American Thyroid Association Guidelines Task Force on Thyroid Nodules and Differentiated Thyroid Cancer. Thyroid 2016;26:1-133. [Crossref] [PubMed]
- Patel KN, Yip L, Lubitz CC, et al. The American Association of Endocrine Surgeons Guidelines for the Definitive Surgical Management of Thyroid Disease in Adults. Ann Surg 2020;271:e21-93. [Crossref] [PubMed]
- Gharib H, Papini E, Paschke R, et al. American Association of Clinical Endocrinologists, Associazione Medici Endocrinologi, and European Thyroid Association Medical guidelines for clinical practice for the diagnosis and management of thyroid nodules: executive summary of recommendations. Endocr Pract 2010;16:468-75. [Crossref] [PubMed]
- Tessler FN, Middleton WD, Grant EG, et al. ACR Thyroid Imaging, Reporting and Data System (TI-RADS): White Paper of the ACR TI-RADS Committee. J Am Coll Radiol 2017;14:587-95. [Crossref] [PubMed]
- Studer H, Peter HJ, Gerber H. Natural heterogeneity of thyroid cells: the basis for understanding thyroid function and nodular goiter growth. Endocr Rev 1989;10:125-35. [Crossref] [PubMed]
- Yildirim Simsir I, Cetinkalp S, Kabalak T. Review of Factors Contributing to Nodular Goiter and Thyroid Carcinoma. Med Princ Pract 2020;29:1-5. [Crossref] [PubMed]
- Cho YA, Kong SY, Shin A, et al. Biomarkers of thyroid function and autoimmunity for predicting high-risk groups of thyroid cancer: a nested case-control study. BMC Cancer 2014;14:873. [Crossref] [PubMed]
- Haymart MR, Repplinger DJ, Leverson GE, et al. Higher serum thyroid stimulating hormone level in thyroid nodule patients is associated with greater risks of differentiated thyroid cancer and advanced tumor stage. J Clin Endocrinol Metab 2008;93:809-14. [Crossref] [PubMed]
- Kim HK, Yoon JH, Kim SJ, et al. Higher TSH level is a risk factor for differentiated thyroid cancer. Clin Endocrinol (Oxf) 2013;78:472-7. [Crossref] [PubMed]
- Jonklaas J, Nsouli-Maktabi H, Soldin SJ. Endogenous thyrotropin and triiodothyronine concentrations in individuals with thyroid cancer. Thyroid 2008;18:943-52. [Crossref] [PubMed]
- Hu MJ, Zhang C, Liang L, et al. Fasting serum glucose, thyroid-stimulating hormone, and thyroid hormones and risk of papillary thyroid cancer: A case-control study. Head Neck 2019;41:2277-84. [Crossref] [PubMed]
- Suzuki S, Nakamura I, Suzuki S, et al. Inappropriate Suppression of Thyrotropin Concentrations in Young Patients with Thyroid Nodules Including Thyroid Cancer: The Fukushima Health Management Survey. Thyroid 2016;26:717-25. [Crossref] [PubMed]
- Guo X, Chen X, Zhang C, et al. Hyperinsulinemia and thyroid peroxidase antibody in Chinese patients with papillary thyroid cancer. Endocr J 2019;66:731-7. [Crossref] [PubMed]
- Sasson M, Kay-Rivest E, Shoukrun R, et al. The T4/T3 quotient as a risk factor for differentiated thyroid cancer: a case control study. J Otolaryngol Head Neck Surg 2017;46:28. [Crossref] [PubMed]
- Wang Z, Lin Y, Jiang Y, et al. The associations between thyroid-related hormones and the risk of thyroid cancer: An overall and dose-response meta-analysis. Front Endocrinol (Lausanne) 2022;13:992566. [Crossref] [PubMed]
- Sun J, Liu J, Wu TT, et al. Sensitivity to thyroid hormone indices are associated with papillary thyroid carcinoma in Chinese patients with thyroid nodules. BMC Endocr Disord 2023;23:126. [Crossref] [PubMed]
- Cibas ES, Ali SZ. The 2017 Bethesda System for Reporting Thyroid Cytopathology. Thyroid 2017;27:1341-6. [Crossref] [PubMed]
- Wang CC, Friedman L, Kennedy GC, et al. A large multicenter correlation study of thyroid nodule cytopathology and histopathology. Thyroid 2011;21:243-51. [Crossref] [PubMed]
- Bongiovanni M, Spitale A, Faquin WC, et al. The Bethesda System for Reporting Thyroid Cytopathology: a meta-analysis. Acta Cytol 2012;56:333-9. [Crossref] [PubMed]
- Thyroid Cancer. An evaluation of the molecular marker tests for thyroid cancer. American Thyroid Association; 2019. Available online: https://www.thyroid.org/patient-thyroid-information/ct-for-patients/august-2019/vol-12-issue-8-p-13-14/
- Xing M. BRAF mutation in thyroid cancer. Endocr Relat Cancer 2005;12:245-62. [Crossref] [PubMed]
- Xing M, Alzahrani AS, Carson KA, et al. Association between BRAF V600E mutation and mortality in patients with papillary thyroid cancer. JAMA 2013;309:1493-501. [Crossref] [PubMed]
- Steward DL, Carty SE, Sippel RS, et al. Performance of a Multigene Genomic Classifier in Thyroid Nodules With Indeterminate Cytology: A Prospective Blinded Multicenter Study. JAMA Oncol 2019;5:204-12. [Crossref] [PubMed]
- Nikiforova MN, Mercurio S, Wald AI, et al. Analytical performance of the ThyroSeq v3 genomic classifier for cancer diagnosis in thyroid nodules. Cancer 2018;124:1682-90. [Crossref] [PubMed]
- Harrell RM, Eyerly-Webb SA, Golding AC, et al. Statistical comparison of afirma gsc and afirma gec outcomes in a community endocrine surgical practice: early findings. Endocr Pract 2019;25:161-4. [Crossref] [PubMed]
- Santos MTD, Buzolin AL, Gama RR, et al. Molecular Classification of Thyroid Nodules with Indeterminate Cytology: Development and Validation of a Highly Sensitive and Specific New miRNA-Based Classifier Test Using Fine-Needle Aspiration Smear Slides. Thyroid 2018;28:1618-26. [Crossref] [PubMed]
- Shen R, Cheng T, Xu C, et al. Novel visualized quantitative epigenetic imprinted gene biomarkers diagnose the malignancy of ten cancer types. Clin Epigenetics 2020;12:71. [Crossref] [PubMed]
- Ishida M, Moore GE. The role of imprinted genes in humans. Mol Aspects Med 2013;34:826-40. [Crossref] [PubMed]
- Barlow DP, Bartolomei MS. Genomic imprinting in mammals. Cold Spring Harb Perspect Biol 2014;6:a018382. [Crossref] [PubMed]
- Murrell A. Genomic imprinting and cancer: from primordial germ cells to somatic cells. ScientificWorldJournal 2006;6:1888-910. [Crossref] [PubMed]
- Zhou J, Cheng T, Li X, et al. Epigenetic imprinting alterations as effective diagnostic biomarkers for early-stage lung cancer and small pulmonary nodules. Clin Epigenetics 2021;13:220. [Crossref] [PubMed]
- Xu H, Zhang Y, Wu H, et al. High Diagnostic Accuracy of Epigenetic Imprinting Biomarkers in Thyroid Nodules. J Clin Oncol 2023;41:1296-306. [Crossref] [PubMed]
- Ferraz C, Ward LS. Could a novel category of molecular testing be emerging? Chin Clin Oncol 2023;12:48. [Crossref] [PubMed]
- Nixon IJ, Ganly I, Hann LE, et al. Nomogram for predicting malignancy in thyroid nodules using clinical, biochemical, ultrasonographic, and cytologic features. Surgery 2010;148:1120-7; discussion 1127-8. [Crossref] [PubMed]
- Huang BL, Chabot JA, Lee JA, et al. A stepwise analysis of the diagnostic algorithm for the prediction of malignancy in thyroid nodules. Surgery 2020;167:28-33. [Crossref] [PubMed]
- Durante C, Grani G, Lamartina L, et al. The Diagnosis and Management of Thyroid Nodules: A Review. JAMA 2018;319:914-24. [Crossref] [PubMed]
- Roth MY, Witt RL, Steward DL. Molecular testing for thyroid nodules: Review and current state. Cancer 2018;124:888-98. [Crossref] [PubMed]
- Rossi ED, Pantanowitz L, Faquin WC. The Role of Molecular Testing for the Indeterminate Thyroid FNA. Genes (Basel) 2019;10:736. [Crossref] [PubMed]
- Obuchowski NA, McClish DK. Sample size determination for diagnostic accuracy studies involving binormal ROC curve indices. Stat Med 1997;16:1529-42. [Crossref] [PubMed]
- Shapiro SS, Wilk MB. An analysis of variance test for normality (complete samples)†. Biometrika 1965;52:591-611. [Crossref]
- McFadden D. Conditional Logit Analysis of Qualitative Choice Behaviour. In: Zarembka P, editor. Frontiers in Econometrics. New York: Academic Press; 1974:105-42.
- Mela CF, Kopalle PK. The impact of collinearity on regression analysis: the asymmetric effect of negative and positive correlations. Applied Economics 2002;34:667-77. [Crossref]
- Steyerberg E. Clinical Prediction Models: A Practical Approach to Development, Validation, and Updating. 2nd ed. New York: Springer; 2009.
- Chan BKC. Applied Statistics for Human Genetics Using R. Adv Exp Med Biol 2018;1082:123-44. [Crossref] [PubMed]
- Abouhashem NS, Talaat SM. Diagnostic utility of CK19 and CD56 in the differentiation of thyroid papillary carcinoma from its mimics. Pathol Res Pract 2017;213:509-17. [Crossref] [PubMed]
- Chiu CG, Strugnell SS, Griffith OL, et al. Diagnostic utility of galectin-3 in thyroid cancer. Am J Pathol 2010;176:2067-81. [Crossref] [PubMed]
- Liu Z, Yu P, Xiong Y, et al. Significance of CK19, TPO, and HBME-1 expression for diagnosis of papillary thyroid carcinoma. Int J Clin Exp Med 2015;8:4369-74. [PubMed]
- Arcolia V, Journe F, Wattier A, et al. Galectin-1 is a diagnostic marker involved in thyroid cancer progression. Int J Oncol 2017;51:760-70. [Crossref] [PubMed]
- Bartolazzi A, Gasbarri A, Papotti M, et al. Application of an immunodiagnostic method for improving preoperative diagnosis of nodular thyroid lesions. Lancet 2001;357:1644-50. [Crossref] [PubMed]
- Dralle H, Machens A, Basa J, et al. Follicular cell-derived thyroid cancer. Nat Rev Dis Primers 2015;1:15077. [Crossref] [PubMed]
- Rashid FA, Munkhdelger J, Fukuoka J, et al. Prevalence of BRAF(V600E) mutation in Asian series of papillary thyroid carcinoma-a contemporary systematic review. Gland Surg 2020;9:1878-900. [Crossref] [PubMed]
- Kim HS, Kim JO, Lee DH, et al. Factors influencing the detection of the BRAF T1799A mutation in papillary thyroid carcinoma. Oncol Rep 2011;25:1639-44. [Crossref] [PubMed]
- Fu G, Chazen RS, Monteiro E, et al. Facilitation of Definitive Cancer Diagnosis With Quantitative Molecular Assays of BRAF V600E and TERT Promoter Variants in Patients With Thyroid Nodules. JAMA Netw Open 2023;6:e2323500. [Crossref] [PubMed]
- Feinberg AP. The Key Role of Epigenetics in Human Disease Prevention and Mitigation. N Engl J Med 2018;378:1323-34. [Crossref] [PubMed]