Interleukine-10 in ovarian cancer
Introduction
Ovarian cancer (OC) is the leading cause of mortality from gynecologic malignancies, with an estimated 313,959 new cases and 207,252 deaths worldwide in 2020 (1). The majority ofOC are diagnosed at an advanced stage (III or IV) (2). Generally, OC may be dichotomised into a low-grade type and a high-grade type. While the high-grade OC accounts for the majority of cases and holds P53 mutations, the low-grade type have an indolent course, are genetically stable tumors which may develop from precursor lesions, as tumor of low malignant potential or endometriosis and have frequent mutations of the KRAS, BRAF, or ERBB2 genes (3). While platinum-based combinatorial chemotherapy coupled with surgical cytoreduction reach a clinical response in the majority of patients, recurrence of tumor in the peritoneal cavity remains a major challenge for improving survival in these patients. Importantly, BRCA1/2 germline mutations are found in 6–15% of epithelial high-grade OC. BRCA1/2 carriers have a better response than non-BRCA1/2 carriers to platinum-based chemotherapies. yielding longer survival (4). Accumulating evidence suggests that inflammation plays a critical role in the tumor microenvironment (TME) in progression of cancer. Among various cytokines, interleukin 10 (IL-10) has been suggested to be involved in immune suppression and tolerance. In this review, we summarize the roles of IL-10 in OC.
OC and the peritoneal cavity
Perhaps the most unique feature of OC is its frequent association with ascites (5,6). This peritoneal fluid contains a wide spectrum of cellular and protein components, which promote tumor survival by inducing immune-evasion and immunosuppression (7). The cellular component is composed of tumor cells and non-tumor cells, including immune cells, mesothelial and endothelial cells (7). The acellular component of ascites is composed of proteins as cytokines, growth factors and metabolites contributing to tumor metastasis and chemoresistance (8,9). Importantly, the phosphoinositide 3-kinase (PI3K) pathway, which is involved in various cellular processes, including cell growth, survival, and metabolism—is frequently upregulated in epithelial OC and plays a pivotal role in preservation of genomic stability and chemoresistance (10). BRCA1/2 mutated OC, and homologous recombination (HR) deficient OC which are wild-type BRCA1/2—present a higher neo-antigen load than HR-proficient cancers, which leads to more efficient anti-tumour immune response (11).
One of the cytokines is interleukin-10 (IL-10), which contributes to an anti-inflammatory TME, that is conducive to tumor growth and chemoresistance (12). IL-10 is an anti-inflammatory cytokine, first described as cytokine synthesis inhibitory factor (13), which has been shown to play a role in OC (14). IL-10 is known to be secreted by monocytes, dendritic cells (DCs), macrophages, natural killer cells, CD4+ and CD8+ T cells, Th17 cells, B cells and OC cells themselves. Importantly, the upregulation of IL-10 expression is most pronounced in OC patients at the stage in which immune check-points, depress the activity of T cells and DCs (15,16).
Interestingly, as opposed to endometrial and cervical cancer, immunotherapies have had limited efficacy in the treatment of OC in the clinical setting (17-19). This is mostly attributed to the immunosuppressive TME within the peritoneal cavity which inhibits cytotoxic T cells function and promotes inhibitory signaling by cells and other soluble factors (20,21).
Ascites in OC, a unique immunologic environment, contains high concentrations of IL-10 (22), which is significantly higher than in OC patients’ serum (23). The role of IL-10 in this ascites TME could be to promote immunosuppression, and has become an active area of research, because it could interfere with checkpoint inhibitors (24,25). IL-10 was demonstrated to promote the anti-apoptotic effect of malignant ascites (26), and its expression correlates with a predominantly Th2 (T helper 2) cells response in advanced stage OC (27). Elevated ascites IL-10 correlated with poor prognosis in OC patients.
IL-10 and the Janus kinase (JAK)/signal transducer and activator of transcription (STAT) pathway
IL-10 receptor dimerization activates JAK1 and TYK2 (Figure 1), which leads to autophosphorylation. This process leads to recruitment and activation, of the transcription factor STAT3 (STAT1 and STAT5 may also be activated). The JAK/STAT signaling pathway induces the expression of important mediators of inflammation and cancer, with mounting evidence that dysregulation of the JAK/STAT pathway is associated with various cancers (28). IL-10 affects the JAK-STAT5 pathway by decreasing the phosphorylation state of STAT5 protein. Decreasing the activity of STAT5 drives the conversion of natural killer cells from cytotoxic killers to tumor promoters (29). In addition, ascites IL-10 upregulates myeloid-derived suppressor cells, which play a key immunosuppressive role in various types of cancers and in OC patients (30). This upregulation of myeloid-derived suppressor cells is promoted by triggering the STAT3 signal (31). In addition, M2-polarized macrophages in ascites from advanced OC, secrete IL-10 in response to activation of STAT3.
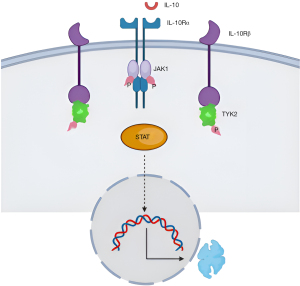
Factors contributing to secretion of IL-10
GATA3 pathway inducing IL-10
GATA3 is a transcription factor with a critical role in the regulation of both innate and adaptive immunity (32). GATA3 is highly expressed in the hematopoietic compartment, and is essential in the development and function of a variety of immune cells, especially T cells (33). ARID1A gene mutation is known to be present in almost half of clear cell and endometrioid OC patients (34). These mutations increase the expression of histone deacetylase 6 (HDAC6) (35) which activates proteins, as HSP90 and α-tubulin, that play pivotalrole in important cellular processes, such as cell adhesion, protein translocation, and formation of immune synapses (36). A recent study has demonstrated that GATA3 activation is increased by HDAC6 overexpression, and leads to IL-10 secretion by OC cells, affecting M2 macrophage polarization [anti-inflammatory macrophages (37)], thereby increasing immune evasion (38).
Nuclear factor kappa B (NF-κB) pathway inducing IL-10
IL-10 is transcriptionally controlled by NF-κB (39). Metadherin, a multifunctional gene associated with several tumor types, was found to be overexpressed in OC tissue (40), and to cause activation of NF-κB signaling, thereby elevating IL-10.
Pigment epithelium-derived factor (PEDF) inducing IL-10
PEDF is a 50-kDa glycoprotein of the serine protease inhibitors family (41). PEDF was found to induce production of IL-10 by immunosuppressive macrophages and supports OC cell survival in the peritoneal cavity of mice models (42).
Another study has demonstrated that Myeloid cells are the predominant producers of IL-10 in the ascites of ovarian tumor-bearing mice (43), mostly with phenotypic markers of monocytes, macrophages, and DCs. CD11b+ cells isolated from mice ascites, secreted IL-10 in significantly higher amounts than mice ID8 tumor cells.
Human OC tumor cells produce IL-10 but in limited amounts (44-46).
OC ascites IL-10 and oncological outcomes
In 1992, a seminal study by our group first identified the presence of IL-10 in the ascites of patients with OC, as compared to ascites of other causes (22), and these findings were confirmed since (42,47-50), also showing IL-10 in the serum of patients with OC (51) but at lower levels than the ascites. Later, IL-10 was found in OC tissue as compared to normal ovaries and OC cell lines (52). Monocytes which produce IL-10 in OC ascites, inhibit cytokine expression and proliferation of autologous T cells (53).
Higher concentration of IL-10 in OC ascites was associated with shorter progression free survival among OC patients (54). Patients with high levels of IL-10 in ascites showed a median progression free survival of 14 months versus 36 months for patients with low concentration of IL-10. IL-10 depletion from ascites by an IL-10 blocking antibody inhibits the survival-promoting activity of these ascites against tumor necrosis factor-related apoptosis-inducing ligand (TRAIL), whereas the IL-10 neutralizing antibody does not affect the survival-promoting activity of an ascites that does not contain IL-10 (54). Therefore, it is suggested that IL-10 contributes to ascites-mediated TRAIL induced apoptosis inhibition. Interestingly, the inhibitory effect of the IL-10 neutralizing antibody is selective for TRAIL as it does not alter the pro survival activity of ascites against cisplatin.
Interestingly, there is one study that did not find correlation between ascites IL-10 and malignant OC, however that study included in the OC cohort also tumors of low malignant potential, and non epithelial OC, such as sex-cord and germ cell tumors (55). Moreover, this study used peritoneal washings rather than ascites, thus significantly diluting the cytokines in the peritoneal cavity (55)
OC serum IL-10 and oncological outcomes
Serum IL-10 concentration was found to be elevated in patient with OC as compared to patients with benign endometriomas. This association was found to be independently associated with a diagnosis of OC, following adjustment for age, cancer antigen 125 (CA-125), IL-6 and IL-8 serum levels (56). Similarly, multiple studies evaluating patients with benign ovarian cysts, endometriomas, and ovarian malignancies, found that serum IL-10 levels were significantly higher in OC patients than in the other study groups (49,57-61). Similarly, serum IL-10 concentration in OC patients were significantly higher than in healthy control group. Moreover, the concentration of serum IL-10 was correlated with OC stage (61-63), grade (59), and survival (64). Treatment of OC cell lines with IL-10 receptor (IL-10R) antibody, resulted in a decrease in the level of matrix metalloproteinases-2, leading to a decrease in cancer proliferation and the invasion ability (61), and IL-10 was shown to have suppressive effects on angiogenesis, tumor growth, and peritoneal dissemination of VEGF-producing OC cells (65).
The level of IL-10 significantly decreased after surgical cytoreduction in the group of patients with advanced disease and reached the level found in serum of patients with early OC (66). Similarly, following cytoreductive surgery in OC patients, there is a decrease in Treg cell and a reduction of circulating IL-10 (67).
In another study, elevated levels of IL-10 were detected in the plasma and in the ascites of OC patients (68), with ascites IL-10 level being significantly higher than plasma.
Serum IL-10 levels decreased after chemotherapy in patients responding, while they remained elevated in non-responding to the treatment (69).
The controversial/ dual function of IL-10 in cancer
IL-10 is often referred to as having a dual role in cancer (70-89) (Table 1). Paradoxically, both IL-10-deficiency and IL-10-overexpression can promote anti-tumor immune responses in mice (90). This disparity probably reflects the significance of local TME in sculpting immune responses and the local variations in bioavailable IL-10. On the one hand, IL-10 is a potent suppressor of DC activation (91), but on the other hand, high concentrations of IL-10 can promote IL-2-dependent proliferation of CD8+ T cells (88). This has led to divergent approaches for targeting IL-10 as an anticancer therapeutic: blocking IL-10R to enhance myeloid cell function (89) versus injecting exogenous IL-10 to directly promote an anti-tumor T cell response through activation of intra-tumoral CD8+ T cells (92). Serum markers of activation of the immune system were detected when IL-10 was administered to patients (93). Pegylated IL-10 was also demonstrated to induce rejection of tumor masses in mice models, by increased CD8 + T cell-immune responses (94), and in a clinical trial on solid tumors, pegilated-IL-10 was demonstrated to be beneficial (95). CmAb-(IL-10)2, an anti-epidermal growth factor receptor (EGFR) antibody conjugated with IL-10 combined with programmed death 1 (PD-1) blockade, significantly improved antitumor effects in advanced tumors in mice (96). Furthermore, in a study among 111 patients with refractory advanced malignant solid tumors, pegylated IL-10 (Pegilodecakin) and anti-PD-1 treatment, showed significant treatment responses, suggesting that pegylated IL-10 plus anti-PD-1 monoclonal antibody may provide a new therapeutic path for previously heavily treated patients with non-small-cell carcinoma and renal cell carcinoma (95).
Table 1
Tumor progression effect | Anti tumor effect |
---|---|
Promote migration and invasion (70,71) | Stabilizing tumor apoptosis (72,73) |
Downregulation of MHC (74,75) | Anti-angiogenic (76,87) |
Suppression of NK cells (77,78) | Increased CD8+ cytotoxicity (79) |
Modulating inflammatory microenvironment (80,81,89) | Decreased macrophages infiltration (82) |
Signalling promoting tumor genesis (83,84,86) | Increased NK cell infiltration (85,88) |
IL, interleukin; MHC, major histocompatibility complex; NK, natural killer.
There is evidence that IL-10 is actually a growth-factor or has a cofactor activity, stimulating growth and differentiation in tumor cells, however this evidence is limited to B-cell tumors (97) and melanoma (86). Therefore, while IL-10 may contribute to proliferation of B cell tumors, there is little evidence supporting a general role for IL-10 as a tumor growth factor.
Overall, most evidence regarding IL-10 in the TME, indicates that IL-10 interferes with the induction or effector function of an antitumor immune response. IL-10 inhibits DC function, which may blunt induction of a response against cancer cells (98-100), and IL-10 significantly suppresses the activation of CD8 T cells in OC patients (16).
Manipulating the IL-10 signaling pathway
The TME develops in such a way that it prevents immune effector cells, mainly CD8+ T cells, from destroying tumor cells. This results in depletion of functional T cells (101,102). Immunotherapy on the other hand relies on sufficient functional T cells which are able to target the tumor, and a tumor which is immunogenic enough to attract T cells. Most immune cells express IL-10R on their membranes (103).
The first demonstration that IL-10R blockade had therapeutic efficacy in OC was provided in mice models (43). Mice that received the IL-10R blocking therapy showed significantly and substantially enhanced survival. This therapeutic efficacy of IL-10R blockade required the presence of T cells. One study analyzed the effects of standard chemotherapy coupled with IL-10 antagonists on the TME of mice harboring OC. They observed an immunosuppressive shift in the myeloid cells, with an increased expression of IL-10 shortly after chemotherapy treatment (104). Combined treatment with IL-10 antagonists significantly decreased the immunosuppressive myeloid cells population, and chemotherapy activated DCs. Coupled, combination treatment significantly increased the number of activated T and DCs as well as expression of cytotoxic factors. It was also concluded that anti IL-10 had to be administered concurrently with chemotherapy to reverse the immunosuppression caused by chemotherapy (104).
Systemic administration of IL-10R antibodies, for the purpose of blocking the IL-10 signaling pathway, triggers tumor cells to secrete more IL-10 and has as a minimal effect on tumor growth (105). Interestingly, when IL-10R antibody is administered with an intra-tumor injection of a toll-like receptor 9 ligand, there is a reversal of tumor antigen presenting cells and suppression of tumor growth in murine model (89).
Tumor vaccines necessitate an effective acute inflammation response in order the vaccine to be of benefit. Therefore, neutralizing the IL-10 produced by DCs or CD4+ T cells, generated better vaccine-mediated anti-tumor responses (106,107). Local tumor blockade of IL-10 through a nano-delivery agent, reduce the population of immunosuppressive cells, such as M2 macrophages, and programmed death ligand-1 (PD-L1)+ cells and has an anti-tumor effect (108).
However, IL-10, especially pegylated IL-10 (Pegilodecakin) suppresses cancer growth in both clinical trials and animal models, although high quality, controlled clinical trial results are yet to come (109). As most immune-associated hematopoietic cells express IL-10 receptor, respond to or secrete IL-10, the effect of IL-10 signaling manipulation on these cells needs to be further evaluated. It was shown that administration of PEG-IL-10 led to an increased infiltration of immune cell (110).
Immunization coupled with IL-10 blockade by IL-10R antibodies drastically—increase vaccine-induced antigen-specific CD8 + T cell responses (111,112). This blockade may be achieved via soluble IL-10R and IL-10R antagonist (113-115). For therapeutic antitumor vaccination, the source of IL-10 in the TME is of importance, because blockade of vaccine-induced IL-10 is more efficient than the blockade of tumor-associated IL-10 (106).
It is known that IL-10 and PD-1 effect their biological functions through various signaling pathways (116). Following a study showing that simultaneous dual blockade of IL-10 and PD-L1 is significantly more effective in restoring antiviral T cell responses in viral infection (116)—further studies in cancer were undertaken. In Melanoma patients, PD-1 blockade was shown to increase expression of IL-10R by CD8+ T cells, thereby increasing their sensitivity to the immunosuppressive effects of endogenous IL-10. IL-10 blockade strengthened the effects of PD-1 blockade in expanding CD8+ T cells and reinforcing their function. That paved the way to the rationale to block both IL-10 and PD-1 (117). Importantly, treatment of mice with ovarian tumor with PD-1 blocking antibody results in an increase in the levels of IL-10 in both ascites and serum. While monotherapy by PD-1 blockade or IL-10 neutralization was inefficient, a combination of these two led to significant improved survival and delayed tumor growth, accompanied by increased anti-tumor T cell responses (118).
In a seminal study, IL-10 was found to increase the expression of PD-1 and its ligand on OC tumor-infiltrating DCs and that this increase is STAT3 dependent (118). PD-1 blockade resulted in compensatory release of IL-10 by tumor-infiltrating DCs. Combination treatment with PD-1 blocking Ab and IL-10 neutralization/IL-10R blockade reduces tumor burden and enhances survival in tumor bearing mice. This combination blockade resulted in a robust increase in both the numbers of activated CD4+ and CD8+ T cells in the ascites of mice. Moreover, the combination blockade reduced the frequency of Myeloid derived suppressor cells in the ascites of tumor bearing mice.
Conclusions
The role of IL-10 in OC is complex. Its impact on tumor growth, immune modulation, and therapeutic responses underscores its importance as a potential target for OC treatment. A deeper understanding of IL-10’s role within the dynamic TME will aid in developing personalized and effective therapeutic strategies that leverage its dual immunosuppressive and antitumor properties. Further research and clinical trials are essential to harness the potential of IL-10 modulation in improving OC outcomes.
Acknowledgments
Funding: None.
Footnote
Peer Review File: Available at https://cco.amegroups.com/article/view/10.21037/cco-23-135/prf
Conflicts of Interest: Both authors have completed the ICMJE uniform disclosure form (available at https://cco.amegroups.com/article/view/10.21037/cco-23-135/coif). The authors have no conflicts of interest to declare.
Ethical Statement: The authors are accountable for all aspects of the work in ensuring that questions related to the accuracy or integrity of any part of the work are appropriately investigated and resolved.
Open Access Statement: This is an Open Access article distributed in accordance with the Creative Commons Attribution-NonCommercial-NoDerivs 4.0 International License (CC BY-NC-ND 4.0), which permits the non-commercial replication and distribution of the article with the strict proviso that no changes or edits are made and the original work is properly cited (including links to both the formal publication through the relevant DOI and the license). See: https://creativecommons.org/licenses/by-nc-nd/4.0/.
References
- Sung H, Ferlay J, Siegel RL, et al. Global Cancer Statistics 2020: GLOBOCAN Estimates of Incidence and Mortality Worldwide for 36 Cancers in 185 Countries. CA Cancer J Clin 2021;71:209-49. [Crossref] [PubMed]
- Lheureux S, Gourley C, Vergote I, et al. Epithelial ovarian cancer. Lancet 2019;393:1240-53. [Crossref] [PubMed]
- Pavlidis N, Rassy E, Vermorken JB, et al. The outcome of patients with serous papillary peritoneal cancer, fallopian tube cancer, and epithelial ovarian cancer by treatment eras: 27 years data from the SEER registry. Cancer Epidemiol 2021;75:102045. [Crossref] [PubMed]
- Shah S, Cheung A, Kutka M, et al. Epithelial Ovarian Cancer: Providing Evidence of Predisposition Genes. Int J Environ Res Public Health 2022;19:8113. [Crossref] [PubMed]
- Kietpeerakool C, Rattanakanokchai S, Jampathong N, et al. Management of drainage for malignant ascites in gynaecological cancer. Cochrane Database Syst Rev 2019;12:CD007794. [Crossref] [PubMed]
- Krugmann J, Schwarz CL, Melcher B, et al. Malignant ascites occurs most often in patients with high-grade serous papillary ovarian cancer at initial diagnosis: a retrospective analysis of 191 women treated at Bayreuth Hospital, 2006-2015. Arch Gynecol Obstet 2019;299:515-23. [Crossref] [PubMed]
- Ahmed N, Stenvers KL. Getting to know ovarian cancer ascites: opportunities for targeted therapy-based translational research. Front Oncol 2013;3:256. [Crossref] [PubMed]
- Kim S, Kim B, Song YS. Ascites modulates cancer cell behavior, contributing to tumor heterogeneity in ovarian cancer. Cancer Sci 2016;107:1173-8. [Crossref] [PubMed]
- Carduner L, Leroy-Dudal J, Picot CR, et al. Ascites-induced shift along epithelial-mesenchymal spectrum in ovarian cancer cells: enhancement of their invasive behavior partly dependant on αv integrins. Clin Exp Metastasis 2014;31:675-88. [Crossref] [PubMed]
- Aliyuda F, Moschetta M, Ghose A, et al. Advances in Ovarian Cancer Treatment Beyond PARP Inhibitors. Curr Cancer Drug Targets 2023;23:433-46. [Crossref] [PubMed]
- Revythis A, Limbu A, Mikropoulos C, et al. Recent Insights into PARP and Immuno-Checkpoint Inhibitors in Epithelial Ovarian Cancer. Int J Environ Res Public Health 2022;19:8577. [Crossref] [PubMed]
- Chang CM, Lam HYP, Hsu HJ, et al. Interleukin-10: A double-edged sword in breast cancer. Tzu Chi Med J 2021;33:203-11. [Crossref] [PubMed]
- Moore KW, Vieira P, Fiorentino DF, et al. Homology of cytokine synthesis inhibitory factor (IL-10) to the Epstein-Barr virus gene BCRFI. Science 1990;248:1230-4. [Crossref] [PubMed]
- Lane D, Matte I, Garde-Granger P, et al. Ascites IL-10 Promotes Ovarian Cancer Cell Migration. Cancer Microenviron 2018;11:115-24. [Crossref] [PubMed]
- Doo DW, Norian LA, Arend RC. Checkpoint inhibitors in ovarian cancer: A review of preclinical data. Gynecol Oncol Rep 2019;29:48-54. [Crossref] [PubMed]
- Li L, Ma Y, Xu Y. Follicular regulatory T cells infiltrated the ovarian carcinoma and resulted in CD8 T cell dysfunction dependent on IL-10 pathway. Int Immunopharmacol 2019;68:81-7. [Crossref] [PubMed]
- Monk BJ, Colombo N, Oza AM, et al. Chemotherapy with or without avelumab followed by avelumab maintenance versus chemotherapy alone in patients with previously untreated epithelial ovarian cancer (JAVELIN Ovarian 100): an open-label, randomised, phase 3 trial. Lancet Oncol 2021;22:1275-89. [Crossref] [PubMed]
- Pujade-Lauraine E, Fujiwara K, Ledermann JA, et al. Avelumab alone or in combination with chemotherapy versus chemotherapy alone in platinum-resistant or platinum-refractory ovarian cancer (JAVELIN Ovarian 200): an open-label, three-arm, randomised, phase 3 study. Lancet Oncol 2021;22:1034-46. [Crossref] [PubMed]
- Moore KN, Bookman M, Sehouli J, et al. Atezolizumab, Bevacizumab, and Chemotherapy for Newly Diagnosed Stage III or IV Ovarian Cancer: Placebo-Controlled Randomized Phase III Trial (IMagyn050/GOG 3015/ENGOT-OV39). J Clin Oncol 2021;39:1842-55. [Crossref] [PubMed]
- Jammal MP, Martins-Filho A, Silveira TP, et al. Cytokines and Prognostic Factors in Epithelial Ovarian Cancer. Clin Med Insights Oncol 2016;10:71-6. [Crossref] [PubMed]
- Wang W, Kryczek I, Dostál L, et al. Effector T Cells Abrogate Stroma-Mediated Chemoresistance in Ovarian Cancer. Cell 2016;165:1092-105. [Crossref] [PubMed]
- Gotlieb WH, Abrams JS, Watson JM, et al. Presence of interleukin 10 (IL-10) in the ascites of patients with ovarian and other intra-abdominal cancers. Cytokine 1992;4:385-90. [Crossref] [PubMed]
- Tonetti CR, de Souza-Araújo CN, Yoshida A, et al. Ovarian Cancer-Associated Ascites Have High Proportions of Cytokine-Responsive CD56bright NK Cells. Cells 2021;10:1702. [Crossref] [PubMed]
- Schwab CL, English DP, Roque DM, et al. Past, present and future targets for immunotherapy in ovarian cancer. Immunotherapy 2014;6:1279-93. [Crossref] [PubMed]
- Wang DH, Guo L, Wu XH. Checkpoint inhibitors in immunotherapy of ovarian cancer. Tumour Biol 2015;36:33-9. [Crossref] [PubMed]
- Hagemann T, Robinson SC, Thompson RG, et al. Ovarian cancer cell-derived migration inhibitory factor enhances tumor growth, progression, and angiogenesis. Mol Cancer Ther 2007;6:1993-2002. [Crossref] [PubMed]
- Dobrzanski MJ, Rewers-Felkins KA, Samad KA, et al. Immunotherapy with IL-10- and IFN-γ-producing CD4 effector cells modulate "Natural" and "Inducible" CD4 TReg cell subpopulation levels: observations in four cases of patients with ovarian cancer. Cancer Immunol Immunother 2012;61:839-54. [Crossref] [PubMed]
- Hu X, Li J, Fu M, et al. The JAK/STAT signaling pathway: from bench to clinic. Signal Transduct Target Ther 2021;6:402. [Crossref] [PubMed]
- Gotthardt D, Putz EM, Grundschober E, et al. STAT5 Is a Key Regulator in NK Cells and Acts as a Molecular Switch from Tumor Surveillance to Tumor Promotion. Cancer Discov 2016;6:414-29. [Crossref] [PubMed]
- Wu L, Deng Z, Peng Y, et al. Ascites-derived IL-6 and IL-10 synergistically expand CD14(+)HLA-DR(-/low) myeloid-derived suppressor cells in ovarian cancer patients. Oncotarget 2017;8:76843-56. [Crossref] [PubMed]
- Ouyang W, Rutz S, Crellin NK, et al. Regulation and functions of the IL-10 family of cytokines in inflammation and disease. Annu Rev Immunol 2011;29:71-109. [Crossref] [PubMed]
- Wan YY. GATA3: a master of many trades in immune regulation. Trends Immunol 2014;35:233-42. [Crossref] [PubMed]
- Ku CJ, Hosoya T, Maillard I, et al. GATA-3 regulates hematopoietic stem cell maintenance and cell-cycle entry. Blood 2012;119:2242-51. [Crossref] [PubMed]
- Kuroda Y, Chiyoda T, Kawaida M, et al. ARID1A mutation/ARID1A loss is associated with a high immunogenic profile in clear cell ovarian cancer. Gynecol Oncol 2021;162:679-85. [Crossref] [PubMed]
- Bitler BG, Wu S, Park PH, et al. ARID1A-mutated ovarian cancers depend on HDAC6 activity. Nat Cell Biol 2017;19:962-73. [Crossref] [PubMed]
- Zhang X, Yuan Z, Zhang Y, et al. HDAC6 modulates cell motility by altering the acetylation level of cortactin. Mol Cell 2007;27:197-213. [Crossref] [PubMed]
- Viola A, Munari F, Sánchez-Rodríguez R, et al. The Metabolic Signature of Macrophage Responses. Front Immunol 2019;10:1462. [Crossref] [PubMed]
- Hsieh TH, Hsu CY, Wu CW, et al. Vorinostat decrease M2 macrophage polarization through ARID1A(6488delG)/HDAC6/IL-10 signaling pathway in endometriosis-associated ovarian carcinoma. Biomed Pharmacother 2023;161:114500. [Crossref] [PubMed]
- Hoesel B, Schmid JA. The complexity of NF-κB signaling in inflammation and cancer. Mol Cancer 2013;12:86. [Crossref] [PubMed]
- Rong C, Shi Y, Huang J, et al. The Effect of Metadherin on NF-κB Activation and Downstream Genes in Ovarian Cancer. Cell Transplant 2020;29:963689720905506. [Crossref] [PubMed]
- Becerra SP, Notario V. The effects of PEDF on cancer biology: mechanisms of action and therapeutic potential. Nat Rev Cancer 2013;13:258-71. [Crossref] [PubMed]
- Ueno S, Sudo T, Saya H, et al. Pigment epithelium-derived factor promotes peritoneal dissemination of ovarian cancer through induction of immunosuppressive macrophages. Commun Biol 2022;5:904. [Crossref] [PubMed]
- Hart KM, Byrne KT, Molloy MJ, et al. IL-10 immunomodulation of myeloid cells regulates a murine model of ovarian cancer. Front Immunol 2011;2:29. [Crossref] [PubMed]
- Nash MA, Lenzi R, Edwards CL, et al. Differential expression of cytokine transcripts in human epithelial ovarian carcinoma by solid tumour specimens, peritoneal exudate cells containing tumour, tumour-infiltrating lymphocyte (TIL)-derived T cell lines and established tumour cell lines. Clin Exp Immunol 1998;112:172-80. [Crossref] [PubMed]
- Berger S, Siegert A, Denkert C, et al. Interleukin-10 in serous ovarian carcinoma cell lines. Cancer Immunol Immunother 2001;50:328-33. [Crossref] [PubMed]
- Carr TM, Adair SJ, Fink MJ, et al. Immunological profiling of a panel of human ovarian cancer cell lines. Cancer Immunol Immunother 2008;57:31-42. [Crossref] [PubMed]
- Zhu XL, Wang HJ, Wang XR, et al. IL-6 secretion of CD4(+) T cells stimulated by LC3-positive extracellular vesicles in human epithelial ovarian cancer. Clin Transl Oncol 2022;24:2222-30. [Crossref] [PubMed]
- Radke J, Schmidt D, Böhme M, et al. -Cytokine level in malignant ascites and peripheral blood of patients with advanced ovarian carcinoma-. Geburtshilfe Frauenheilkd 1996;56:83-7. [Crossref] [PubMed]
- Mustea A, Könsgen D, Braicu EI, et al. Expression of IL-10 in patients with ovarian carcinoma. Anticancer Res 2006;26:1715-8. [PubMed]
- Lane D, Matte I, Garde-Granger P, et al. Inflammation-regulating factors in ascites as predictive biomarkers of drug resistance and progression-free survival in serous epithelial ovarian cancers. BMC Cancer 2015;15:492. [Crossref] [PubMed]
- Giuntoli RL 2nd, Webb TJ, Zoso A, et al. Ovarian cancer-associated ascites demonstrates altered immune environment: implications for antitumor immunity. Anticancer Res 2009;29:2875-84. [PubMed]
- Pisa P, Halapi E, Pisa EK, et al. Selective expression of interleukin 10, interferon gamma, and granulocyte-macrophage colony-stimulating factor in ovarian cancer biopsies. Proc Natl Acad Sci U S A 1992;89:7708-12. [Crossref] [PubMed]
- Loercher AE, Nash MA, Kavanagh JJ, et al. Identification of an IL-10-producing HLA-DR-negative monocyte subset in the malignant ascites of patients with ovarian carcinoma that inhibits cytokine protein expression and proliferation of autologous T cells. J Immunol 1999;163:6251-60. [Crossref] [PubMed]
- Matte I, Lane D, Laplante C, et al. Profiling of cytokines in human epithelial ovarian cancer ascites. Am J Cancer Res 2012;2:566-80. [PubMed]
- de Lima CA, Silva Rodrigues IS, Martins-Filho A, et al. Cytokines in peritoneal fluid of ovarian neoplasms. J Obstet Gynaecol 2020;40:401-5. [Crossref] [PubMed]
- Crispim PCA, Jammal MP, Antão PKA, et al. IL6, IL8, and IL10 in the distinction of malignant ovarian neoplasms and endometriomas. Am J Reprod Immunol 2020;84:e13309. [Crossref] [PubMed]
- Sipak-Szmigiel O, Włodarski P, Ronin-Walknowska E, et al. Serum and peritoneal fluid concentrations of soluble human leukocyte antigen, tumor necrosis factor alpha and interleukin 10 in patients with selected ovarian pathologies. J Ovarian Res 2017;10:25. [Crossref] [PubMed]
- Micheli DC, Jammal MP, Martins-Filho A, et al. Serum cytokines and CXCR2: potential tumour markers in ovarian neoplasms. Biomarkers 2020;25:474-82. [Crossref] [PubMed]
- Wertel I, Polak G, Tarkowski R, et al. Evaluation of IL-10 and TGF-β levels and myeloid and lymphoid dendritic cells in ovarian cancer patients. Ginekol Pol 2011;82:414-20. [PubMed]
- Lambeck AJ, Crijns AP, Leffers N, et al. Serum cytokine profiling as a diagnostic and prognostic tool in ovarian cancer: a potential role for interleukin 7. Clin Cancer Res 2007;13:2385-91. [Crossref] [PubMed]
- Ke X, Shen L. Targeting cytokines secreted by CD4(+) CD25(high) CD127(low) regulatory T cells inhibits ovarian cancer progression. Scand J Immunol 2019;89:e12736. [Crossref] [PubMed]
- Zhang L, Liu W, Wang X, et al. Prognostic value of serum IL-8 and IL-10 in patients with ovarian cancer undergoing chemotherapy. Oncol Lett 2019;17:2365-9. [PubMed]
- Nowak M, Glowacka E, Szpakowski M, et al. Proinflammatory and immunosuppressive serum, ascites and cyst fluid cytokines in patients with early and advanced ovarian cancer and benign ovarian tumors. Neuro Endocrinol Lett 2010;31:375-83. [PubMed]
- Zhu X, Ying LS, Xu SH, et al. Clinicopathologic and prognostic significance of serum levels of cytokines in patients with advanced serous ovarian cancer prior to surgery. Zhonghua Bing Li Xue Za Zhi 2010;39:666-70. [PubMed]
- Kohno T, Mizukami H, Suzuki M, et al. Interleukin-10-mediated inhibition of angiogenesis and tumor growth in mice bearing VEGF-producing ovarian cancer. Cancer Res 2003;63:5091-4. [PubMed]
- Nowak M, Głowacka E, Lewkowicz P, et al. Sub-optimal primary surgery leads to unfavorable immunological changes in ovarian cancer patients. Immunobiology 2018;223:1-7. [Crossref] [PubMed]
- Napoletano C, Bellati F, Landi R, et al. Ovarian cancer cytoreduction induces changes in T cell population subsets reducing immunosuppression. J Cell Mol Med 2010;14:2748-59. [Crossref] [PubMed]
- Santin AD, Bellone S, Ravaggi A, et al. Increased levels of interleukin-10 and transforming growth factor-beta in the plasma and ascitic fluid of patients with advanced ovarian cancer. BJOG 2001;108:804-8. [Crossref] [PubMed]
- Zakrzewska I, Poznański J. Serum levels of interleukin-10 in patients with ovarian carcinoma in response to chemotherapy. Przegl Lek 2003;60:18-20. [PubMed]
- Chen L, Shi Y, Zhu X, et al. IL‑10 secreted by cancer‑associated macrophages regulates proliferation and invasion in gastric cancer cells via c‑Met/STAT3 signaling. Oncol Rep 2019;42:595-604. [Crossref] [PubMed]
- Sung WW, Wang YC, Lin PL, et al. IL-10 promotes tumor aggressiveness via upregulation of CIP2A transcription in lung adenocarcinoma. Clin Cancer Res 2013;19:4092-103. [Crossref] [PubMed]
- Fluckiger AC, Durand I, Banchereau J. Interleukin 10 induces apoptotic cell death of B-chronic lymphocytic leukemia cells. J Exp Med 1994;179:91-9. [Crossref] [PubMed]
- Roberts AW, Davids MS, Pagel JM, et al. Targeting BCL2 with Venetoclax in Relapsed Chronic Lymphocytic Leukemia. N Engl J Med 2016;374:311-22. [Crossref] [PubMed]
- Steinbrink K, Wölfl M, Jonuleit H, et al. Induction of tolerance by IL-10-treated dendritic cells. J Immunol 1997;159:4772-80. [Crossref] [PubMed]
- Steinbrink K, Jonuleit H, Müller G, et al. Interleukin-10-treated human dendritic cells induce a melanoma-antigen-specific anergy in CD8(+) T cells resulting in a failure to lyse tumor cells. Blood 1999;93:1634-42. [Crossref] [PubMed]
- Gazzinelli R, Xu Y, Hieny S, et al. Simultaneous depletion of CD4+ and CD8+ T lymphocytes is required to reactivate chronic infection with Toxoplasma gondii. J Immunol 1992;149:175-80. [Crossref] [PubMed]
- Lin A, Yan WH. Human Leukocyte Antigen-G (HLA-G) Expression in Cancers: Roles in Immune Evasion, Metastasis and Target for Therapy. Mol Med 2015;21:782-91. [Crossref] [PubMed]
- Serrano AE, Menares-Castillo E, Garrido-Tapia M, et al. Interleukin 10 decreases MICA expression on melanoma cell surface. Immunol Cell Biol 2011;89:447-57. [Crossref] [PubMed]
- Liu Y, Zhou N, Zhou L, et al. IL-2 regulates tumor-reactive CD8(+) T cell exhaustion by activating the aryl hydrocarbon receptor. Nat Immunol 2021;22:358-69. [Crossref] [PubMed]
- Oft M. IL-10: master switch from tumor-promoting inflammation to antitumor immunity. Cancer Immunol Res 2014;2:194-9. [Crossref] [PubMed]
- Sato T, Terai M, Tamura Y, et al. Interleukin 10 in the tumor microenvironment: a target for anticancer immunotherapy. Immunol Res 2011;51:170-82. [Crossref] [PubMed]
- Zheng LM, Ojcius DM, Garaud F, et al. Interleukin-10 inhibits tumor metastasis through an NK cell-dependent mechanism. J Exp Med 1996;184:579-84. [Crossref] [PubMed]
- Levy Y, Brouet JC. Interleukin-10 prevents spontaneous death of germinal center B cells by induction of the bcl-2 protein. J Clin Invest 1994;93:424-8. [Crossref] [PubMed]
- Rousset F, Peyrol S, Garcia E, et al. Long-term cultured CD40-activated B lymphocytes differentiate into plasma cells in response to IL-10 but not IL-4. Int Immunol 1995;7:1243-53. [Crossref] [PubMed]
- Mocellin S, Panelli M, Wang E, et al. IL-10 stimulatory effects on human NK cells explored by gene profile analysis. Genes Immun 2004;5:621-30. [Crossref] [PubMed]
- Yue FY, Dummer R, Geertsen R, et al. Interleukin-10 is a growth factor for human melanoma cells and down-regulates HLA class-I, HLA class-II and ICAM-1 molecules. Int J Cancer 1997;71:630-7. [Crossref] [PubMed]
- Gallo O, Masini E, Morbidelli L, et al. Role of nitric oxide in angiogenesis and tumor progression in head and neck cancer. J Natl Cancer Inst 1998;90:587-96. [Crossref] [PubMed]
- Groux H, Bigler M, de Vries JE, et al. Inhibitory and stimulatory effects of IL-10 on human CD8+ T cells. J Immunol 1998;160:3188-93. [Crossref] [PubMed]
- Vicari AP, Chiodoni C, Vaure C, et al. Reversal of tumor-induced dendritic cell paralysis by CpG immunostimulatory oligonucleotide and anti-interleukin 10 receptor antibody. J Exp Med 2002;196:541-9. [Crossref] [PubMed]
- O'Garra A, Barrat FJ, Castro AG, et al. Strategies for use of IL-10 or its antagonists in human disease. Immunol Rev 2008;223:114-31. [Crossref] [PubMed]
- Allavena P, Piemonti L, Longoni D, et al. IL-10 prevents the differentiation of monocytes to dendritic cells but promotes their maturation to macrophages. Eur J Immunol 1998;28:359-69. [Crossref] [PubMed]
- Emmerich J, Mumm JB, Chan IH, et al. IL-10 directly activates and expands tumor-resident CD8(+) T cells without de novo infiltration from secondary lymphoid organs. Cancer Res 2012;72:3570-81. [Crossref] [PubMed]
- Lauw FN, Pajkrt D, Hack CE, et al. Proinflammatory effects of IL-10 during human endotoxemia. J Immunol 2000;165:2783-9. [Crossref] [PubMed]
- Mumm JB, Oft M. Pegylated IL-10 induces cancer immunity: the surprising role of IL-10 as a potent inducer of IFN-γ-mediated CD8(+) T cell cytotoxicity. Bioessays 2013;35:623-31. [Crossref] [PubMed]
- Naing A, Papadopoulos KP, Autio KA, et al. Safety, Antitumor Activity, and Immune Activation of Pegylated Recombinant Human Interleukin-10 (AM0010) in Patients With Advanced Solid Tumors. J Clin Oncol 2016;34:3562-9. [Crossref] [PubMed]
- Qiao J, Liu Z, Dong C, et al. Targeting Tumors with IL-10 Prevents Dendritic Cell-Mediated CD8(+) T Cell Apoptosis. Cancer Cell 2019;35:901-915.e4. [Crossref] [PubMed]
- Rousset F, Garcia E, Defrance T, et al. Interleukin 10 is a potent growth and differentiation factor for activated human B lymphocytes. Proc Natl Acad Sci U S A 1992;89:1890-3. [Crossref] [PubMed]
- Caux C, Massacrier C, Vanbervliet B, et al. Interleukin 10 inhibits T cell alloreaction induced by human dendritic cells. Int Immunol 1994;6:1177-85. [Crossref] [PubMed]
- Zeller JC, Panoskaltsis-Mortari A, Murphy WJ, et al. Induction of CD4+ T cell alloantigen-specific hyporesponsiveness by IL-10 and TGF-beta. J Immunol 1999;163:3684-91. [Crossref] [PubMed]
- Gao JX, Madrenas J, Zeng W, et al. CD40-deficient dendritic cells producing interleukin-10, but not interleukin-12, induce T-cell hyporesponsiveness in vitro and prevent acute allograft rejection. Immunology 1999;98:159-70. [Crossref] [PubMed]
- Blank CU, Haining WN, Held W, et al. Defining 'T cell exhaustion'. Nat Rev Immunol 2019;19:665-74. [Crossref] [PubMed]
- Gasser S, Lim LHK, Cheung FSG. The role of the tumour microenvironment in immunotherapy. Endocr Relat Cancer 2017;24:T283-95. [Crossref] [PubMed]
- Saraiva M, O'Garra A. The regulation of IL-10 production by immune cells. Nat Rev Immunol 2010;10:170-81. [Crossref] [PubMed]
- Hartl CA, Bertschi A, Puerto RB, et al. Combination therapy targeting both innate and adaptive immunity improves survival in a pre-clinical model of ovarian cancer. J Immunother Cancer 2019;7:199. [Crossref] [PubMed]
- Llopiz D, Ruiz M, Infante S, et al. IL-10 expression defines an immunosuppressive dendritic cell population induced by antitumor therapeutic vaccination. Oncotarget 2017;8:2659-71. [Crossref] [PubMed]
- Llopiz D, Aranda F, Díaz-Valdés N, et al. Vaccine-induced but not tumor-derived Interleukin-10 dictates the efficacy of Interleukin-10 blockade in therapeutic vaccination. Oncoimmunology 2015;5:e1075113. [Crossref] [PubMed]
- Liu XS, Dyer J, Leggatt GR, et al. Overcoming original antigenic sin to generate new CD8 T cell IFN-gamma responses in an antigen-experienced host. J Immunol 2006;177:2873-9. [Crossref] [PubMed]
- Shen L, Li J, Liu Q, et al. Local Blockade of Interleukin 10 and C-X-C Motif Chemokine Ligand 12 with Nano-Delivery Promotes Antitumor Response in Murine Cancers. ACS Nano 2018;12:9830-41. [Crossref] [PubMed]
- Autio K, Oft M. Pegylated Interleukin-10: Clinical Development of an Immunoregulatory Cytokine for Use in Cancer Therapeutics. Curr Oncol Rep 2019;21:19. [Crossref] [PubMed]
- Mumm JB, Emmerich J, Zhang X, et al. IL-10 elicits IFNγ-dependent tumor immune surveillance. Cancer Cell 2011;20:781-96. [Crossref] [PubMed]
- Brooks DG, Lee AM, Elsaesser H, et al. IL-10 blockade facilitates DNA vaccine-induced T cell responses and enhances clearance of persistent virus infection. J Exp Med 2008;205:533-41. [Crossref] [PubMed]
- Chen S, Ni G, Wu X, et al. Blocking IL-10 signalling at the time of immunization renders the tumour more accessible to T cell infiltration in mice. Cell Immunol 2016;300:9-17. [Crossref] [PubMed]
- Silva JR, Sales NS, Silva MO, et al. Expression of a soluble IL-10 receptor enhances the therapeutic effects of a papillomavirus-associated antitumor vaccine in a murine model. Cancer Immunol Immunother 2019;68:753-63. [Crossref] [PubMed]
- Ni G, Wang Y, Cummins S, et al. Inhibitory mechanism of peptides with a repeating hydrophobic and hydrophilic residue pattern on interleukin-10. Hum Vaccin Immunother 2017;13:518-27. [Crossref] [PubMed]
- Berezhnoy A, Stewart CA, Mcnamara JO 2nd, et al. Isolation and optimization of murine IL-10 receptor blocking oligonucleotide aptamers using high-throughput sequencing. Mol Ther 2012;20:1242-50. [Crossref] [PubMed]
- Brooks DG, Ha SJ, Elsaesser H, et al. IL-10 and PD-L1 operate through distinct pathways to suppress T-cell activity during persistent viral infection. Proc Natl Acad Sci U S A 2008;105:20428-33. [Crossref] [PubMed]
- Sun Z, Fourcade J, Pagliano O, et al. IL10 and PD-1 Cooperate to Limit the Activity of Tumor-Specific CD8+ T Cells. Cancer Res 2015;75:1635-44. [Crossref] [PubMed]
- Lamichhane P, Karyampudi L, Shreeder B, et al. IL10 Release upon PD-1 Blockade Sustains Immunosuppression in Ovarian Cancer. Cancer Res 2017;77:6667-78. [Crossref] [PubMed]