Updates in the role of the tumor microenvironment cellular crosstalk and genetic signatures in diffuse large B-cell lymphoma: a narrative review
Introduction
Lymphoma is divided into two main groups: Hodgkin lymphomas and non-Hodgkin lymphomas (1). Non-Hodgkin lymphomas make up 70–80% of cases and have an incidence of 386,000 cases worldwide per year. They include both aggressive (high-grade) and less aggressive (low-grade) variants of B-lymphocyte and T-lymphocyte-derived lymphomas (2). The most common non-Hodgkin lymphoma is diffuse large B-cell lymphoma (DLBCL), which comprises 40% of all cases of newly diagnosed lymphoma, with an annual incidence of over 100,000 patients worldwide (3,4). Without treatment, DLBCL patients develop early symptomatic disease with death occurring within months (5). DLBCL shows a wide range of variation with regards to its genetics as well as clinical and histologic parameters (1). Lymphoma classification schemes since the 2017 WHO update have required subclassification by cell of origin (COO); although molecularly defined, most practices now use immunohistochemistry (IHC) as a validated surrogate for grouping into germinal center B cell (GCB) and non-GCB or activated B cell (ABC) subtypes (see Appendix 1 for definition of “cell of origin”). One of the most used algorithms is the Hans criteria, which stratifies DLBCL into GCB and non-GCB subtypes based on three widely available immunostains: CD10, BCL6, and MUM1. These subtypes are driven by different intracellular oncogenic signaling pathways (5). In addition, the classification of these tumors based on COO is important as there is a significant difference in the five-year survival following R-CHOP (rituximab, cyclophosphamide, doxorubicin hydrochloride, vincristine sulfate, and prednisone) chemotherapy (87–92% in GCB vs. 44% in ABC) (6).
Several recent studies have highlighted the role of the tumor microenvironment (TME) in DLBCL pathogenesis, based on the interactions between tumor cells, stromal elements (fibroblasts, endothelial cells), and immune cells [mast cells (MCs), macrophages, and lymphocytes] (see Appendix 1 for definition of “tumor microenvironment”) (6). Digital gene expression measurements have assessed the transcriptional features of DLBCL as related to survival prediction and have provided a pure TME-based prognosticator that has been added to the COO risk stratification (see Appendix 1 for definition of “digital gene expression measurement”) (7). Despite the differences in the TME composition of each DLBCL tumor, the majority of DLBCL cases are still being treated with the same treatment regimen (5), except for primary central nervous system tumors (1). Studying the genetic alterations in tumor cells and their microenvironment contributes greatly to developing individualized therapeutic treatment regimens (8).
While many studies have been done on the genetics of the TME in DLBCL, there is a lack of a comprehensive review of updated achievements in the field (9). Herein we review recent studies examining the extracellular TME and associated genetic alterations in DLBCL as well as the tumor cells’ reaction to the surrounding microenvironment. This includes a review of the features of each TME element and its interaction with tumor cells of DLBCL.
Objective
The present analysis is meant to provide an overview of specific features of key players in the TME of DLBCL and provide a concise review of the TME markers as they relate to classification of DLBCL to pave the road for potential targeted therapeutic regimens. We present this article in accordance with the Narrative Review reporting checklist (available at https://cco.amegroups.com/article/view/10.21037/cco-23-124/rc).
Methods
To identify updates on the genetic landscape of DLBCL TMEs, we systematically reviewed literature published in English in Medline for DLBCL-related articles on gene expression studies of TME. Forty-seven articles including original articles and systematic reviews were identified and included that were published between 2006 and 2023 (Table 1).
Table 1
Items | Specification |
---|---|
Date of search | 07.07.2022–10.17.2023 |
Databases | Medline |
Search terms used | Extracellular matrix of DLBCL, TME of the DLBCL, gene expression in DLBCL, endothelial cells in DLBCL, macrophages in DLBCL, lymphocytes in DLBCL, myofibroblasts in DLBCL, mast cells in DLBCL |
Timeframe | 04.2006–04.2023 |
Inclusion and exclusion criteria | Including criteria: original and review articles, articles published in English with full text available |
Excluding criteria: articles in non-English language, articles with no full text available online, book chapters, editorial comments | |
Selection process | The primary selection of the articles was performed by the first author and the manuscript was written based on those |
The other authors reviewed the manuscript, made comments with suggested set of complimentary of articles | |
The primary author finalized the manuscript based on the primary and complementary list of the articles |
DLBCL, diffuse large B cell lymphoma; TME, tumor microenvironment.
TME
The behavior of DLBCL depends mainly on the interactions between lymphoma cells and their TME, which focus on immune-escape mechanisms and tumor growth (1). The TME in DLBCL consists of stromal elements and non-malignant immune cells including natural killer (NK) cells, dendritic cells (DCs), macrophages, CD4+ T cells, and CD8+ T cells. Study of the gene expression patterns of TME in DLBCL has proposed two distinct signatures. The first pattern is the stromal-1 signature in which the TME has high expression of extracellular matrix elements, such as osteonectin, collagen and laminin, fibronectin, thrombospondin, connective-tissue growth factor, and remodeling proteins (see Appendix 1 for definition of “extracellular matrix”). The second pattern is the stromal-2 signature which is highly vascularized with high expression of endothelial cell markers, vascular endothelial growth factor (VEGF) receptor, endothelial tyrosine kinase, components of caveolae, and genes expressed in adipocytes, like RBP4 and ADIPOQ (see Appendix 1 for definition of “components of caveolae”) (2). Studying the gene signatures of the TME and its correlation with the risk score obtained from the gene expression models has shown that the presence of CD8 + T-cells and naïve CD4 + T-cells conveys a favorable prognosis to the rituximab-containing standard regimen, while the presence of activated DCs is associated with a poor prognosis. Genes associated with a favorable molecular signature include those involved in cell differentiation and activation of Wnt signaling, as well as downregulation of adaptive immune response systems. Wnt signaling in conjunction with the TGF-pathway has therapeutic implications in DLBCL. Potential medications act by down regulating the β-catenin pathway (3). Genes associated with unfavorable molecular signatures tend to be involved in activation of the acute immune system response and angiogenesis (4). Study of immune receptor genes has shown that the proportion of CD4 memory-activated T cells and follicular helper T cells is relatively high in the patients known to have a better prognosis. In contrast, memory B cells and naïve B cells are relatively more abundant in patients with a worse prognosis (5). TME composition has a prominent role in the development, progression, and growth of DLBCL and has been added to conventional risk stratification factors such as the international prognostic index, COO, and molecular alterations (6). Pileri et al. explored 15 COO related genes, 6 oncogenic genes, 47 TME genes, and 5 housekeeping genes, and noted that overall less expression of TME genes had worse both overall and progression-free survival (see Appendix 1 for definition of “housekeeping genes”) (7). Another study with the same aim established seven prognostic immune gene markers (TIMP2, QKI, LCP2, LAMP2, ITGAM, CSF3R, and AAK1) that are differentially expressed in immune cells or stromal cells; and it was noted that these markers can robustly and reliably predict an individualized immunomolecular prognostic model for DLBCL patients (8). To clarify the reproducibility of the profiles, a NanoString-based GEP profiling study was performed by Plaça et al. They were able to reproduce 4 signatures on a panel of 117 genes, including the COO, the T-cell-mediated host response cluster, and the MYC activity signature (see Appendix 1 for definition of “NanoString-based GEP profiling study”). They were not able to reproduce the B cell receptor/proliferation-related or oxidative phosphorylation-related signatures (9).
In addition, alterations in the TME help explain the pathogenesis of Richter syndrome (RS). RS or Richter transformation, is defined as the development of an aggressive, high-grade B-cell lymphoma that evolves from a preexisting chronic lymphocytic leukemia/small cell leukemia (CLL/SLL) with a median overall survival of 10 months from the diagnosis. There are two variants of RS described: DLBCL-type and Hodgkin lymphoma-type RS. The pathogenesis of DLBCL-type RS is related to genetic changes, modifications of immune regulators, and B cell receptor pathway hyperactivation. The genetic changes of DLBCL-type RS include disruption of TP53, deletions of CDKN2A, gain-of-function mutations of NOTCH1, and hyperactivation of c-MYC. The B cell receptor pathway hyperactivation in DLBCL-type RS is shown by the analysis of the rearrangement of IGHV genes in 80% of the cases, which proves the occurrence of a transformation event from the previous indolent phase. Modifications of immune regulators in the microenvironment of DLBCL-type RS have been observed by the presence of higher levels of FOXP3+ Treg lymphocytes, immune checkpoint molecules LAG3, TIGIT, and CD163+ M2-like macrophages (see Appendix 1 for definition of “immune checkpoint molecules”) (10).
The various key components of the TME in DLBCL [endothelial cells, myofibroblasts (MF), MCs, lymphocytes, and macrophages], along with the features of their related genes are discussed in the following sections.
Endothelial cells
Angiogenesis is an important prognostic factor for lymphoma and is a target for next-generation therapeutics. Tumor-associated blood vessels have different morphology, gene expression, and function compared with normal blood vessels. The capillaries in DLBCL are made up of two endothelial cell layers with abundant cytoplasm that are configured parallel to each other and create a slit-like lumen (11,12). The signal transducer and activator of transcription-3 (STAT3) factor and the genes that it regulates are involved in promoting tumor cell proliferation, survival, angiogenesis, and metastasis through the expression of high levels of CD163+ macrophages and CD8+ T cells. Activation of STAT3 results in hypoxia-inducible factor 1 alpha and VEGF expression in tumor cells, while VEGF, in turn, activates STAT3 in endothelial cells. STAT3 inhibits the expression of the anti-angiogenesis transcription factor p53. In addition, overexpression of the isozyme SPHK1, responsible for the production of sphingosine-1 phosphate, acts as a potent inducer of angiogenesis in DLBCL (Figure 1) (13,14). However, it should be noted that follow up of patients treated with anti-angiogenic agents such as anti-VEGF-A antibodies, bevacizumab, and R-CHOP has shown that increased vascularity in DLBCL patients may have only minor importance for the disease course as these regimens did not show increased therapeutic efficacy on patients (15).
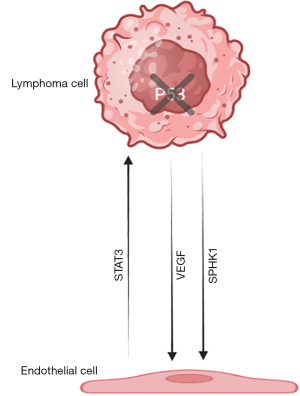
MF
MF are stromal cells that also play an integral role in lymphoma TME interactions. These cells are related to fibroblastic reticular cells (FRC), an immunologically specialized stromal element (16). These stromal cells are regulated in part by transforming growth factor-beta 1 (TGF-β1) whose function includes regulation of myofibroblastic differentiation, fibrosis, and differentiation of regulatory T cells that suppress lymphocyte activation, resulting in immune evasion by malignant lymphoma cells. However, TGF-β1 facilitates the generation of pro-inflammatory CD4+ Th17+ T helper lymphocytes (Figure 2) (17). A higher density of MF is present in tumors with the ‘stromal-1’ signature as well as in tumors that express FRC-related genes that are associated with better outcomes. This has been shown by comparing TME panels containing various expression patterns of MF, DCs, and CD4+ T-cell transcripts (16). Similar results were seen in a gene expression profiling study performed with a 1,028-gene panel that included 13 immune signatures and 4 stromal cytotypes, in which the higher expression of myofibroblast, DC, and CD4+ T-cell-related genes was correlated with better outcomes in DLBCL (18).
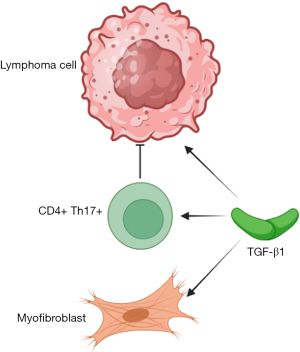
MCs
MCs are attracted to TME by tumor-secreted stem cell factors (c-KIT ligand) and produce several angiogenic factors as well as matrix metalloproteinases (MMPs) and histamine, which promote tumor vascularization. They also secrete tumor necrosis factor alpha-α and IL-10, which promote the immune tolerance mediated by regulatory T (Treg) cells, and therefore tumor progression (Figure 3) (19).
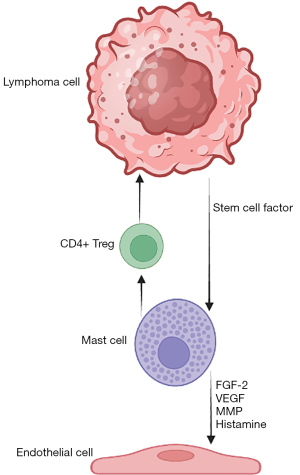
Tryptase, a serine proteinase secreted by MC, increases the levels of VEGF and fibroblast growth factor-2. Higher levels of tryptase, as well as increased microvascular density, have been shown in patients with suboptimal response to R-CHOP therapy compared to patients who have completely recovered. This supports the angiogenic potential of MCs in DLBCL (20). Another study in contrast, showed that a higher number of MC independently predicts better lymphoma-specific survival in DLBCL (21).
Genetic changes in immune cells
Lymphocytes
In 1863, Rudolf Virchow first described leukocyte infiltration in tumor tissues (19). In B-cell lymphomas, both antigen-presenting cells and B cells play a role in the antitumor response by cytotoxic T cells, whereas the tumor cells are dependent on T helper cells for survival. This generates a complex interaction between tumor cells and their surroundings (22). Each DLBCL has its own unique number of tumor-infiltrating T cells (TILs), ratio of CD8+ to CD4+ T cells, and T cell receptor repertoire that results in different behaviors (see Appendix 1 for definition of “tumor-infiltrating lymphocytes”). Although a higher T-cell percentage (≥20%) is significantly correlated with better patient survival, there is still controversy regarding the ratio of CD4:CD8 T-cells and DLBCL prognosis (23).
Lymphocytes have a key role in tumor immune evasion mechanisms, as ineffective tumor antigen presentation eliminates anti-tumor responses. Tumor immune evasion mechanisms include the lack of tumor cell recognition, and the dysfunction of effector T lymphocytes or NK cells. Lack of tumor cell recognition can occur if the tumor cell does not express human leukocyte antigen class I (HLA-I) or if the beta2-microglobulin gene is inactivated. Although the lack of MHC class I expression helps facilitate escape from CD8+ cytotoxic T cells, tumor cells can still be recognized by NK cells (2). CD58, the receptor of the CD2+ NK cells or T cells, also has an important antigen-presenting role. In DLBCL, inactivation or loss of the CD58 gene is implied by the loss of recognition of tumor cells by cytotoxic T cells and NK cells and is therefore associated with an unfavorable prognosis in de novo DLBCL (24,25).
The activity of TILs is hampered by impaired actin dynamics, and the expression of inhibitory molecules, such as CD200, programmed cell death protein-1 ligand (PD-L1), and herpes virus infection. The hampered TILs in turn cause the effector T lymphocyte dysfunction. The receptors on TILs that respond to these inhibitory ligands are T cell immunoglobulin and mucin domain-containing protein-3 (TIM-3), lymphocyte activation gene-3 (LAG-3), programmed cell death protein-1 (PD-1), and CD70. The expression of immune inhibitory receptors is induced by IL-12 and TGF-β, which are secreted by lymphoma cells (1). Finally, the tissue inhibitor metalloproteinase is an antiapoptotic protein produced by both TILs and TME elements, and is an inhibitor of GC B-cell apoptosis, which contributes to the poor prognosis of aggressive B-cell neoplasms (25).
Immune inhibitory receptors are signaling molecules that regulate the immune system by inducing tolerance through T-cell exhaustion. Cancer cells hijack this mechanism to escape from the immune system. DLBCL cells express PD-1 and thereby dysregulate T cells through the same mechanism. They also induce the expression of TIM-3 on CD4+ Th1 and CD8+ cytotoxic T cells. LAG-3 upregulation can also be found in CD4+, CD8+, and NK cells and induces PD-1 expression on TILs. LAG-3 also negatively affects T cell response to tumor cells by binding to MHC class II (26).
In general, the high proportion of inhibitory T-cells translates to unfavorable survival in DLBCL (27). On the other hand, T cells themselves have a defensive mechanism by providing diverse receptors which can confuse tumor cells. TMEs of aggressive B cell tumors have been shown to contain less diverse T cell receptors (28).
CD4+ lymphocytes
CD4+ T cells have an important role in generating tumor immunity through the activation of antigen-presenting DCs, which can then prime CD8+ cells (29). These CD4+ TILs are known to be mostly CD4+CD45RO+ memory T cells. CD4+ T cells could stimulate antigen presentation through CD40L, resulting in lymphoma-specific T-cell responses and longer overall patient survival (2,30).
Both a high percentage (>6%) of tumor-infiltrating CD8+ T cells and CD4+ T-cell infiltration have been correlated with increased survival. Regulatory T (Treg) cells are a subset of CD4+ T cells that express CD25 and transcription factor forkhead box P3 (FoxP3) (2,30). Treg cells are subdivided into naive-like central Treg (cTreg) cells and activated effector Treg (eTreg) cells. The eTreg cells exert a strong immunosuppressive effect by secreting cytokines, T cell immunoglobulin and mucin-domain containing-3 (Tim-3). Zhong et al. showed for the first time that Tim-3+Foxp3+Treg cells are remarkably enriched in the TME of DLBCL patients, and that is predictive of a poor prognosis for DLBCL patients. These findings have resulted in anti-Tim-3 antibodies as a new therapeutic option (31).
Treg cells regulate immune responses through four major mechanisms: metabolic regulation, direct cytolysis, regulation of antigen-presenting cells, and secretion of inhibitory cytokines. The migratory capacity of Treg cells due to chemokine receptors (CCR4, CCR2, and CCR8) is a key factor in regulating tissue restricted inflammation. Inhibitory receptors such as cytotoxic T-lymphocyte-associated protein 4 (CTLA-4), PD-1, LAG-3, T cell immunoreceptor with Ig and ITIM domains (TIGIT), and TIM-3 have been studied in Treg cells. CTLA-4 blockade inhibits CTLA4+ Treg mediated inhibition of T cell activation. TIGIT is a selective immune receptor that represses effector T cell function while enhancing Treg cell stability and function. Further investigation of the role of PD-1, LAG-3, and TIM3 is warranted (30).
T helper (Th) 17 cells, a subset of CD4+ T cells, secrete IL-17 and IL-21. Their differentiation is inhibited by TGF-β, and retinoid-related orphan receptor γt (ROR-γt) is one of the key transcription factors in their differentiation. The gene fusion between immunoglobulin heavy chain and interferon regulatory factor 8 (IRF8) has been shown to lead to IRF8 overexpression in tumor cells. IRF8 upregulation inhibits the generation of Th17 cells by suppressing RORγt and predicts unfavorable clinical outcomes in DLBCL patients (32).
Th9 cells are another subset of CD4+ T cells and were first discovered in 2008 by Dardalhon et al. (33). Th9 T cells are characterized by the secretion of the IL-9 cytokine. Naive T cells can differentiate into Th9 cells in the presence of IL-4 and TGF-β. IL-9 is significantly increased in the serum of patients with DLBCL, and there is a correlation between IL-9 and high international prognostic index scores. They participate in tumor immunity by activating MCs and recruiting DCs into tumor sites (34).
T follicular helper (Tfh) cells are a specialized subset of CD4+ cells that depend on GC B cells for their maintenance. Tfh cells are defined by their ability to support GC formation, select GC B cells, and determine their differentiation into memory B cells and plasma cells through CD40L-mediated binding of CD40. As expected, GC-DLBCL maintains a CD4+ T cell and Tfh cell signature, in contrast to ABC-DLBCL (35). Tfh cells are divided into the T follicular regulatory (Tfr)-like subset (CD25+CD4+CXCR5+) and the CD25− Tfh subset (CD25−CD4+CXCR5+). Patients with DLBCL have a high level of Tfr-like cells in both blood and TME which can suppress the CD8+ T-cell response and promote tumor cell proliferation (36).
CD8+ lymphocytes
CD8+ T cells directly kill tumor cells and maintain immune surveillance (see Appendix 1 for definition of “immune surveillance”). They lose their function in the presence of chronic antigen exposure, which causes T cell exhaustion (37). Both CD4+ T cells and CD8+ T cells in DLBCL patients have been shown to have upregulation of TIM-3-associated with T cell exhaustion and tumor progression (38). CD8+ T cells in DLBCL express higher levels of immune inhibitory receptors including PD-1, TIM-3, and LAG-3, compared to CD8+ T cells in follicular and Hodgkin lymphomas; this is correlated with an increased frequency of cells with terminal exhaustion markers including PD-1high CD39high T cells, as well as increased transcription factors T-bet and Eomesodermin (EOMES)high (39). Adenosine receptors are another immune checkpoint on CD8+ T cells in DLBCL, which regulate antitumor immunity and are correlated with shorter survival (40).
CD8+ T cells can generally easily approach tumor cells without T cell receptor specificity through the CXCR5 receptor. In DLBCL, not only is it the clonal expansion of the T cell receptor but also the inhibitors of CXCR5 receptors that result in tumor progression. Inhibitors of CXCR5+ CD8+ T cell differentiation dysregulate transcription factors including Bcl6, Blimp1, and Id2 (41).
Macrophages
Tumor-associated macrophages (TAMs) are a key link between inflammation and cancer. The M1 and M2 categories of TAM identify the two extremes of the broad spectrum of macrophage function. Depending on their environment, the microbial component lipopolysaccharide can lead to the M1 polarization of the macrophages while interleukin 4 (IL-4) can induce M2 macrophage polarization. Activated M1 TAMs overexpress CD80, CD86, and CD16/32 and have a high capacity to present antigen and elicit a strong Th1 response; alternatively, activated M2 TAMs express arginase-1, mannose receptor (CD206), and high levels of anti-inflammatory molecules such as IL-10 and scavenger (CD163) receptors (42-44). CD163 is relatively specific to M2 TAMs and is preferred over the pan-macrophage marker CD68. High expression of both CD68 and CD163 is shown to be significantly associated with poorer overall survival in DLBCL (45,46).
TAMs have negative prognostic effects in DLBCL related to an increased number of M2-TAMs as the higher number of M2-TAMs is frequently found in the stromal-2 signature. M2-TAMs down-regulate MHC class II antigen expression including HLA-DR (43). M2-TAMs with CD14+ HLA-DRlow CD120blow can suppress T cell proliferation, and also directly remodel the extracellular matrix in DLBCL, leading to increased angiogenesis (42). Another study reported the same results in DLBCL patients receiving R-CHOP therapy, and additionally demonstrated that DLBCL patients with TAMs showing weak HLA-DR and strong CD163 expression had a worse prognosis (43).
The understanding of the molecular pathogenesis of DLBCL has prompted the targeting of several molecular pathways, including new small-molecule inhibitors such as Bruton’s tyrosine kinase inhibitors, phosphatidylinositol-3-kinase δ inhibitors, Exportin-1 inhibitors, cereblon modulators, EZH2 gene inhibitors, dual-acting inhibitors of both class I and II histone deacetylase and class I PI3Ks, and RNA polymerase I inhibitors (see Appendix 1 for definition of “small-molecule inhibitors”). Monoclonal antibodies with an anti-CD20 effect are also routinely used for treatment. Other antibodies with different mechanisms of action and chimeric antigen receptors (CAR)-T cells are also under development for treating DLBCL, with the majority targeting CD19, CD20, CD22, and CD79b on the cell surface. Bispecific antibodies, on the other hand, recognize two different epitopes or antigens on the autologous patient’s cytotoxic T cells and result in redirection against DLBCL. Cellular therapies for DLBCL with monospecific CAR-T cells, which activate the autologous immune system against the microenvironment of the DLBCL, are already available for clinical use (see Appendix 1 for definition of “CAR-T therapy”). However, clinical trials have shown that these novel treatments are sometimes efficacious only in a subgroup of patients who cannot be classified by standard molecular and histochemical classification categories (47).
Conclusions
DLBCL shows a high degree of heterogeneity with regards to its underlying TME composition and genetics. Studies have demonstrated that evaluation of the TME and associated gene signatures can be used to help improve prognostication in DBLCL. Combining different risk stratification and prognostication systems such as COO and TME could provide new rationales for using new targeted therapeutic regiments. Evaluation of the TME in DLBCL may open additional avenues for treatments that are specifically targeted at the TME. Evaluation of the TME through immunohistochemical or molecular methods, in addition to the classification of tumors by COO, could potentially lead to more comprehensive treatments for DLBCL that target different pathways in tumorigenesis.
Acknowledgments
Funding: None.
Footnote
Reporting Checklist: The authors have completed the Narrative Review reporting checklist. Available at https://cco.amegroups.com/article/view/10.21037/cco-23-124/rc
Peer Review File: Available at https://cco.amegroups.com/article/view/10.21037/cco-23-124/prf
Conflicts of Interest: All authors have completed the ICMJE uniform disclosure form (available at https://cco.amegroups.com/article/view/10.21037/cco-23-124/coif). The authors have no conflicts of interest to declare.
Ethical Statement: The authors are accountable for all aspects of the work in ensuring that questions related to the accuracy or integrity of any part of the work are appropriately investigated and resolved.
Open Access Statement: This is an Open Access article distributed in accordance with the Creative Commons Attribution-NonCommercial-NoDerivs 4.0 International License (CC BY-NC-ND 4.0), which permits the non-commercial replication and distribution of the article with the strict proviso that no changes or edits are made and the original work is properly cited (including links to both the formal publication through the relevant DOI and the license). See: https://creativecommons.org/licenses/by-nc-nd/4.0/.
References
- Höpken UE, Rehm A. Targeting the Tumor Microenvironment of Leukemia and Lymphoma. Trends Cancer 2019;5:351-64. [Crossref] [PubMed]
- Mulder TA, Wahlin BE, Österborg A, et al. Targeting the Immune Microenvironment in Lymphomas of B-Cell Origin: From Biology to Clinical Application. Cancers (Basel) 2019;11:915. [Crossref] [PubMed]
- Dharanipragada P, Parekh N. In Silico Identification and Functional Characterization of Genetic Variations across DLBCL Cell Lines. Cells 2023;12:596. [Crossref] [PubMed]
- Merdan S, Subramanian K, Ayer T, et al. Gene expression profiling-based risk prediction and profiles of immune infiltration in diffuse large B-cell lymphoma. Blood Cancer J 2021;11:2. [Crossref] [PubMed]
- Liang XJ, Fu RY, Wang HN, et al. An Immune-Related Prognostic Classifier Is Associated with Diffuse Large B Cell Lymphoma Microenvironment. J Immunol Res 2021;2021:5564568. [Crossref] [PubMed]
- Koya J, Kataoka K. More accurate prognostic prediction in diffuse large B-cell lymphoma: beyond cell-of-origin. Ann Oncol 2018;29:2284-6. [Crossref] [PubMed]
- Pileri S, Mazzara S, Derenzini E, et al. Integrating tumor- and microenvironment-reflecting genes in a unique and routine-applicable assay for accurate risk prediction in DLBCL. Hematological Oncology 2019;37:139-40. [Crossref]
- Pan T, He Y, Chen H, et al. Identification and Validation of a Prognostic Gene Signature for Diffuse Large B-Cell Lymphoma Based on Tumor Microenvironment-Related Genes. Front Oncol 2021;11:614211. [Crossref] [PubMed]
- Plaça JR, Diepstra A, Los T, et al. Reproducibility of Gene Expression Signatures in Diffuse Large B-Cell Lymphoma. Cancers (Basel) 2022;14:1346. [Crossref] [PubMed]
- Mahmoud AM, Gaidano G, Mouhssine S. Immunological Aspects of Richter Syndrome: From Immune Dysfunction to Immunotherapy. Cancers (Basel) 2023;15:1015. [Crossref] [PubMed]
- Ribatti D, Tamma R, Annese T, et al. Vascular Growth in Lymphomas: Angiogenesis and Alternative Ways. Cancers (Basel) 2023;15:3262. [Crossref] [PubMed]
- Huang X, Bai X, Cao Y, et al. Lymphoma endothelium preferentially expresses Tim-3 and facilitates the progression of lymphoma by mediating immune evasion. J Exp Med 2010;207:505-20. [Crossref] [PubMed]
- Solimando AG, Annese T, Tamma R, et al. New Insights into Diffuse Large B-Cell Lymphoma Pathobiology. Cancers (Basel) 2020;12:1869. [Crossref] [PubMed]
- Tamma R, Ingravallo G, Gaudio F, et al. STAT3, tumor microenvironment, and microvessel density in diffuse large B cell lymphomas. Leuk Lymphoma 2020;61:567-74. [Crossref] [PubMed]
- Menzel L, Höpken UE, Rehm A. Angiogenesis in Lymph Nodes Is a Critical Regulator of Immune Response and Lymphoma Growth. Front Immunol 2020;11:591741. [Crossref] [PubMed]
- Ciavarella S, Vegliante MC, Fabbri M, et al. Dissection of DLBCL microenvironment provides a gene expression-based predictor of survival applicable to formalin-fixed paraffin-embedded tissue. Ann Oncol 2018;29:2363-70. [Crossref] [PubMed]
- Kis K, Liu X, Hagood JS. Myofibroblast differentiation and survival in fibrotic disease. Expert Rev Mol Med 2011;13:e27. [Crossref] [PubMed]
- Pileri SA, Vegliante MC, Ciavarella S. Diffuse large B-cell lymphoma: the stuff of cell-of-origin and microenvironment. Oncotarget 2019;10:3991-3. [Crossref] [PubMed]
- Ribatti D. Mast cells and macrophages exert beneficial and detrimental effects on tumor progression and angiogenesis. Immunol Lett 2013;152:83-8. [Crossref] [PubMed]
- Marinaccio C, Ingravallo G, Gaudio F, et al. Microvascular density, CD68 and tryptase expression in human diffuse large B-cell lymphoma. Leuk Res 2014;38:1374-7. [Crossref] [PubMed]
- Hedström G, Berglund M, Molin D, et al. Mast cell infiltration is a favourable prognostic factor in diffuse large B-cell lymphoma. Br J Haematol 2007;138:68-71. [Crossref] [PubMed]
- Booman M, Douwes J, Glas AM, et al. Mechanisms and effects of loss of human leukocyte antigen class II expression in immune-privileged site-associated B-cell lymphoma. Clin Cancer Res 2006;12:2698-705. [Crossref] [PubMed]
- Younes S, Natkunam Y. FOXes at play in the lymphoma landscape. Leuk Lymphoma 2021;62:5-7. [Crossref] [PubMed]
- Younes S, Zhao S, Bharadwaj S, et al. Detection of Aberrant CD58 Expression in a Wide Spectrum of Lymphoma Subtypes: Implications for Treatment Resistance. Mod Pathol 2023;36:100256. [Crossref] [PubMed]
- Cioroianu AI, Stinga PI, Sticlaru L, et al. Tumor Microenvironment in Diffuse Large B-Cell Lymphoma: Role and Prognosis. Anal Cell Pathol (Amst) 2019;2019:8586354. [Crossref] [PubMed]
- Chen BJ, Dashnamoorthy R, Galera P, et al. The immune checkpoint molecules PD-1, PD-L1, TIM-3 and LAG-3 in diffuse large B-cell lymphoma. Oncotarget 2019;10:2030-40. [Crossref] [PubMed]
- Autio M, Leivonen SK, Brück O, et al. Immune cell constitution in the tumor microenvironment predicts the outcome in diffuse large B-cell lymphoma. Haematologica 2021;106:718-29. [Crossref] [PubMed]
- Keane C, Gould C, Jones K, et al. The T-cell Receptor Repertoire Influences the Tumor Microenvironment and Is Associated with Survival in Aggressive B-cell Lymphoma. Clin Cancer Res 2017;23:1820-8. [Crossref] [PubMed]
- Judd J, Dulaimi E, Li T, et al. Low Level of Blood CD4(+) T Cells Is an Independent Predictor of Inferior Progression-free Survival in Diffuse Large B-cell Lymphoma. Clin Lymphoma Myeloma Leuk 2017;17:83-8. [Crossref] [PubMed]
- Yano H, Andrews LP, Workman CJ, et al. Intratumoral regulatory T cells: markers, subsets and their impact on anti-tumor immunity. Immunology 2019;157:232-47. [Crossref] [PubMed]
- Zhong W, Liu X, Zhu Z, et al. High levels of Tim-3(+)Foxp3(+)Treg cells in the tumor microenvironment is a prognostic indicator of poor survival of diffuse large B cell lymphoma patients. Int Immunopharmacol 2021;96:107662. [Crossref] [PubMed]
- Zhong W, Xu X, Zhu Z, et al. Increased expression of IRF8 in tumor cells inhibits the generation of Th17 cells and predicts unfavorable survival of diffuse large B cell lymphoma patients. Oncotarget 2017;8:49757-72. [Crossref] [PubMed]
- Dardalhon V, Awasthi A, Kwon H, et al. IL-4 inhibits TGF-beta-induced Foxp3+ T cells and, together with TGF-beta, generates IL-9+ IL-10+ Foxp3(-) effector T cells. Nat Immunol 2008;9:1347-55. Correction appears in Nat Immunol 2009;10:551.
- Wan J, Wu Y, Ji X, et al. IL-9 and IL-9-producing cells in tumor immunity. Cell Commun Signal 2020;18:50. [Crossref] [PubMed]
- Mintz MA, Cyster JG. T follicular helper cells in germinal center B cell selection and lymphomagenesis. Immunol Rev 2020;296:48-61. [Crossref] [PubMed]
- Ma X, Zha J, He J, et al. T follicular helper cell-mediated IL-21 production suppresses FOXP3 expression of T follicular regulatory-like cells in diffuse large B cell lymphoma patients. Hum Immunol 2020;81:452-9. [Crossref] [PubMed]
- Zhang T, Ren T, Song Z, et al. Genetic Mutations of Tim-3 Ligand and Exhausted Tim-3(+) CD8(+) T Cells and Survival in Diffuse Large B Cell Lymphoma. J Immunol Res 2020;2020:6968595. [Crossref] [PubMed]
- Xiao T, Zhang L, Chen L, et al. Tim-3 expression is increased on peripheral T cells from diffuse large B cell lymphoma. Tumour Biol 2014;35:7951-6. [Crossref] [PubMed]
- Vallée A, Shourian M, Johnson N, et al. Evaluation of T-Cell Compartment By Complex Multiparameter Flow Cytometry Reveals Distinct Patterns of T-Cell Exhaustion in DLBCL, FL and HL Patients. Blood;134:2806.
- Wang X, Zhang T, Song Z, et al. Tumor CD73/A2aR adenosine immunosuppressive axis and tumor-infiltrating lymphocytes in diffuse large B-cell lymphoma: correlations with clinicopathological characteristics and clinical outcome. Int J Cancer 2019;145:1414-22. [Crossref] [PubMed]
- Tang J, Zha J, Guo X, et al. CXCR5(+)CD8(+) T cells present elevated capacity in mediating cytotoxicity toward autologous tumor cells through interleukin 10 in diffuse large B-cell lymphoma. Int Immunopharmacol 2017;50:146-51. [Crossref] [PubMed]
- Petty AJ, Yang Y. Tumor-Associated Macrophages in Hematologic Malignancies: New Insights and Targeted Therapies. Cells 2019;8:1526. [Crossref] [PubMed]
- Yamamoto W, Nakamura N, Tomita N, et al. Human leukocyte antigen-DR expression on flow cytometry and tumor-associated macrophages in diffuse large B-cell lymphoma treated by rituximab, cyclophosphamide, doxorubicin, vincristine and prednisone therapy: retrospective cohort study. Leuk Lymphoma 2014;55:2721-7. [Crossref] [PubMed]
- Yunna C, Mengru H, Lei W, et al. Macrophage M1/M2 polarization. Eur J Pharmacol 2020;877:173090. [Crossref] [PubMed]
- Li YL, Shi ZH, Wang X, et al. Tumor-associated macrophages predict prognosis in diffuse large B-cell lymphoma and correlation with peripheral absolute monocyte count. BMC Cancer 2019;19:1049. [Crossref] [PubMed]
- Kridel R, Steidl C, Gascoyne RD. Tumor-associated macrophages in diffuse large B-cell lymphoma. Haematologica 2015;100:143-5. [Crossref] [PubMed]
- Patriarca A, Gaidano G. Investigational drugs for the treatment of diffuse large B-cell lymphoma. Expert Opin Investig Drugs 2021;30:25-38. [Crossref] [PubMed]