Safety and tolerability of radiotherapy in combination with systemic targeted therapies for treatment of hepatocellular carcinoma: a narrative review
Introduction
Background
Hepatocellular carcinoma (HCC) is a leading cause of cancer morbidity and mortality, with rising incidence worldwide (1). A significant proportion of patients with HCC have advanced disease at diagnosis and may not be candidates for curative therapies. The first-line targeted therapy in advanced HCC is atezolizumab, a programmed cell death ligand-1 (PD-L1) antibody, and bevacizumab, a vascular endothelial growth factor (VEGF) inhibitor, or tyrosine kinase inhibitor (TKI) therapy such as sorafenib. A combination of monoclonal antibody bevacizumab and immune checkpoint inhibitor atezolizumab has been shown to improve survival outcomes in patients with advanced HCC compared to sorafenib with potentially greater tolerability (2-4). Small but significant improvements in overall survival have been demonstrated in randomised controlled trials of sorafenib vs. placebo; however a greater benefit is seen in patients with hepatitis C virus (HCV)-related cirrhosis compared to hepatitis B virus (HBV)-related and alcohol-related disease (5,6). Lenvatinib, is another option for first line therapy and is preferred by some clinicians because of better tolerability and longer time to tumour progression compared to sorafenib in the REFLECT trial (7). Second line therapy for HCC is typically regorafenib, which has been shown to improve median survival compared with placebo after progression on sorafenib (8). The role of other immune checkpoint inhibitors such as nivolumab and pembrolizumab in treating HCC is an area of active research, with some recent studies showing some encouraging results (9,10).
Radiotherapy (RT) is one of the cornerstones of modern cancer therapy and is commonly used to treat the primary tumour or metastases for symptomatic relief and local control. Common indications include symptomatic bone metastases or bulky nodal metastasis, malignant spinal cord compression or liver lesions causing abdominal pain.
Rationale for study
Modern RT techniques have enabled liver-directed RT to become an effective and safe treatment modality for many patients with HCC (11). Additionally, proliferation in systemic therapy options has led to a stepwise improvement in response rates and median survival. This has the effect of increasing the number of patients with advanced disease requiring RT for control of symptomatic disease in the palliative context. However, there have been minimal reports of the safety and tolerability of RT in the setting of concurrent systemic targeted and immunotherapy (12-14).
Objectives
We performed a review of the literature to assist the clinician in determining whether administering palliative RT is safe with contemporary systemic therapy. This review aims to summarise all currently available data about the toxicity of concurrent RT to the liver or other body sites with the targeted drugs and immunotherapeutic agents used to treat advanced HCC. Due to the paucity of data surrounding concurrent RT and systemic therapy in HCC, and that palliative RT is commonly applied to extra-hepatic disease, we expanded the review to include non-HCC cohorts. We present this article in accordance with the Narrative Review reporting checklist (available at https://cco.amegroups.com/article/view/10.21037/cco-23-140/rc).
Methods
A search of the available literature was performed, as described in Table 1. Combination therapy was defined as RT within 2 weeks of systemic targeted therapy. Due to the limited number of studies examining combination therapy in HCC, the inclusion criteria were expanded to include non-HCC cohorts treated with drugs commonly used in HCC. Where immune checkpoint blockade was used, only studies including intra-hepatic irradiation were included due to a large number of studies in other cancers and anatomical locations (15,16). Studies analysing the use of bevacizumab with intra-cranial RT were excluded, as it has been the subject of previous reviews (14,17). Other exclusion criteria are shown in Table 1.
Table 1
Items | Specification |
---|---|
Date of search | September 2023 |
Databases and other sources searched | PubMed, reference lists of included papers and relevant reviews |
Search terms used | Radiotherapy [MeSH terms and/or Title/Abstract (including SBRT, Hypofractionated Radiotherapy)] |
Hepatocellular Carcinoma (MeSH terms and/or Title/Abstract) | |
Immune Checkpoint Inhibitors (MeSH terms and/or Title/Abstract) or Immunotherapy (MeSH terms and/or Title/Abstract), or Immune Checkpoint Inhibitor (MeSH terms and/or Title/Abstract), or Immunological Antineoplastic Agents (MeSH terms) | |
Sorafenib (MeSH terms and/or Title/Abstract) or Bevacizumab (MeSH terms and/or Title/Abstract) or Lenvatinib (MeSH terms and/or Title/Abstract) | |
Timeframe | 2011–2023 |
Inclusion and exclusion criteria | Inclusion criteria |
• Clinical trials and case reports examining the use of combination therapy with radiotherapy and systemic therapy commonly used in HCC | |
• Published after 2011 | |
• Reports toxicities | |
Exclusion criteria | |
• Unrelated texts | |
• Vaccine studies | |
• Studies reporting bevacizumab in combination with intracranial-radiotherapy | |
• Studies reporting immunotherapy where no liver lesions were irradiated | |
• Studies with patients younger than 18 years old | |
• Studies reporting the use of selective internal radiotherapy | |
• Studies analysing drugs not commonly used in HCC | |
• Studies where chemoradiotherapy was neoadjuvant to surgical excision | |
Selection process | Selection conducted by members of the research team using a set of clearly defined inclusion and exclusion criteria (as outlined above), agreed upon by the team |
SBRT, stereotactic body radiotherapy; HCC, hepatocellular carcinoma.
Titles and abstracts were screened, and the full text reviewed where information in the abstract was insufficient. The following data were extracted: study type, year of publication, number of patients, tumour characteristics, radiation technique, radiation dose and fractionation, type and dose of systemic therapy and timing of systemic therapy compared to RT. Toxicities grade 3 or higher, according to the National Cancer Institute Common Terminology Criteria for Adverse Events (CTCAE), were included (18). Acute toxicities were defined as any adverse events occurring within three months of commencement of treatment, while late toxicities were defined as occurring after three months. Because of the high background rate of severe off-target toxicity with systemic therapy, we specified high-grade toxicities relevant to the organ treated with RT as most studies were conducted in the context of definitive treatment for other cancers with RT (e.g., liver: hepatic enzyme elevation, lung, bowel and skin complaints). All other toxicities not within the radiation field were listed as other.
Discussion
Our search of the literature yielded 67 articles included which are included in this review (N=1,313). There were forty prospective studies, 22 case reports, and 3 retrospective studies. Twenty-three studies reported combination RT and concurrent TKIs (22 sorafenib, 1 regorafenib) (Table 2) (19-41), 22 studies reported combined bevacizumab and RT (Table 3) (42-63), three studies reported combined lenvatinib and RT (Table 4) (64-66), and 19 reported combined RT and immune checkpoint inhibitors (Table 5) (67-85).
Table 2
Treated site | Study | Primary tumour | N | RT type | RT (Gy), med/Fx | Acute grade 3–4 toxicities (n) | Acute grade 5 toxicities (n) | Patients with severe toxicity, n | Late grade 3–4 toxicities (n) | Late grade 5 toxicities (n) | Patients experiencing late severe toxicity, n |
---|---|---|---|---|---|---|---|---|---|---|---|
Sorafenib | |||||||||||
Liver | Hsieh et al. (19) | HCC | 1 | SBRT | 48/6 | NR | NR | 0 | NR | NR | NR |
Brade et al. (20) | HCC | 4 | SBRT (low effective irradiated liver volume) | 51/6 | Thrombocytopaenia (n=1) | NR | 1 | NR | NR | NR | |
12 | SBRT (high veff) | 33/6 | Liver enzyme changes (n=3), small bowel obstruction (n=1), lower GI bleed (n=1), other (n=4) | Upper GI haemorrhage (n=1) | 8 | NR | NR | NR | |||
Goody et al. (21) | Various | 18 | SBRT | 45/6 | Other (n=6) | NR | 6 | NR | NR | NR | |
15 | Whole liver | 21.6/6 | Other (n=3) | Liver toxicity (n=2) | 5 | NR | NR | NR | |||
Brade et al. (22) | Various | 15 | SBRT | 42/6 | Chest wall pain (n=3) | NR | 3 | NR | NR | NR | |
11 | WLRT | 21.6/6 | Pneumonitis (n=1), chest wall pain (n=1) | Liver toxicity (n=2) | 4 | NR | NR | NR | |||
Chen et al. (23) | HCC | 40 | Technique NR | 50/20 | Liver enzyme changes (n=4), other (n=5) | NR | NR | Hepatic toxicity (n=6), gastric ulcer (n=1) | Hepatic toxicity (n=3) | NR | |
Kim et al. (24) | HCC | 34 | Low dose 3DCRT or IMRT | 60/20–25 | Hyperbilirubinaemia (n=5), abdominal pain (n=2), duodenal haemorrhage (n=1), other (n=2) | NR | 10 | NR | NR | NR | |
Kim et al. (25) | HCC | 1 | Technique NR | 35/14 | Other (n=1) | NR | 1 | NR | NR | NR | |
Murray et al. (26) | Various | 34 | Technique NR | 30/10 | Oesophagitis (n=1), hepatic toxicity (n=1), abdominal pain (n=1), diarrhoea (n=1), thrombocytopaenia (n=1), other (n=4) | Bowel perforation (n=1) | 9 | NR | NR | NR | |
Horgan et al. (27) | HCC | 1 | Conventional | 8/1 | Other (n=1) | NR | 1 | NR | NR | NR | |
1 | Conventional | 30/10 | NR | NR | 0 | NR | NR | NR | |||
Abdo/pelvis | Aparicio et al. (28) | Pancreatic Adenocarcinoma | 12 | Technique NR | 45/25 | Leukoencephalopathy (n=1), thrombocytopaenia (n=1), other (n=1) | NR | 3 | NR | NR | NR |
Chiorean et al. (29) | Pancreatic Adenocarcinoma | 25 | IMRT | 45/25 | Thrombocytopaenia (n=7), nausea (n=2), vomiting (n=1), other (n=5) | NR | NR | Thrombocytopaenia (n=7), GI bleed (n=6), GI ulcer (n=5), diarrhoea (n=1), abdominal pain (n=1), other (n=9) | GI bleed (n=1) | NR | |
Cai et al. (30) | HCC | 1 | IMRT | 54/28 | NR | NR | 0 | Duodenal perforation (n=1) occurred 17 months after RT | NR | 1 | |
Brain | Arneson et al. (31) | Various | 23 | SBRT | 20–77.5/1–5 | Other (n=7) | NR | 7 | NR | NR | NR |
Morikawa et al. (32) | Breast | 19 | Whole brain | 30/10 | Other (n=25) | NR | NR | NR | NR | NR | |
Hottinger et al. (33) | Glioma | 17 | 60/2 | Other (n=21) | NR | 11 | Other (n=5) | NR | 4 | ||
Den et al. (34) | Glioma | 18 | 3DCRT, fractionated | 35–60/10 | Rash (n=3), neuropathy (n=1), myelopathy (n=1), other (n=14) | NR | NR | NR | NR | NR | |
Other | Chen et al. (35) | HCC | 2 | Technique NR | 30–45/13–15 | Candida oesophagitis (n=2) | NR | 2 | NR | NR | NR |
Kitajima et al. (36) | HCC | 1 | EBRT | 45/18 | Other (n=3) | NR | 1 | NR | NR | NR | |
Stieb et al. (37) | HCC | 1 | Technique NR | 20–36/5–12 | Radiation recall dermatitis (n=1) | NR | 1 | NR | NR | NR | |
Inoue et al. (38) | RCC | 2 | Technique NR | 30/10 | Sigmoid colon perforation after 4 weeks of treatment (n=1) | Sigmoid colon perforation after 4 weeks of treatment (n=1) | 2 | NR | NR | NR | |
Kashihara et al. (39) | HCC | 1 | 3DCRT, fractionated | 30/10, 25/5 | GI haemorrhage (n=1), haematoma in right gluteus maximus (n=1) | NR | 1 | NR | NR | NR | |
Mehta et al. (40) | HCC | 1 | Technique NR | 30/10 | NR | NR | 0 | NR | NR | NR | |
Regorafenib | |||||||||||
Lung | Roberto et al. (41) | Colorectal | 1 | SBRT | 54 Gy | Other (n=1) | NR | 1 | NR | NR | NR |
TKI, tyrosine kinase inhibitor; RT, radiotherapy; med, median; Fx, fractions; HCC, hepatocellular carcinoma; SBRT, stereotactic body radiotherapy; NR, not recorded; GI, gastrointestinal; WLRT, whole liver radiotherapy; 3DCRT, 3D conformal radiotherapy; IMRT, intensity-modulated radiotherapy; EBRT, external beam radiotherapy; RCC, renal cell carcinoma.
Table 3
Treated site | Study | Primary tumour | N | RT type | RT (Gy), med/Fx | Acute grade 3–4 toxicities (n) | Acute grade 5 toxicities (n) | Patients who experienced acute severe toxicity, n | Late grade 3–4 toxicities (n) | Late grade 5 toxicities (n) | Patients who experienced late severe toxicity, n |
---|---|---|---|---|---|---|---|---|---|---|---|
Thorax | Lind et al. (42) | NSCLC | 6 | Technique NR | 66/33 | NR | NR | 0 | Radiation pneumonitis (n=2) | NR | 2 |
Socinski et al. (43) | NSCLC | 45 | Technique NR | 74/37 | Oesophagitis (n=13), haemorrhage (n=1), pneumonitis (n=1), other (n=14) | NR | NR | Tracheoesophageal fistula (n=1) | NR | 1 | |
Abdomen | Morganti et al. (44) | CRC | 18 | LDRT | 2.4/12 | Other (n=4) | NR | 2 | NR | NR | NR |
Chadha et al. (45) | Pancreatic adenocarcinoma | 17 | 3DCRT, IMRT | 50.4/28 | Diarrhoea (n=2), other (n=1) | NR | 3 | NR | NR | NR | |
Berlin et al. (46) | Pancreatic adenocarcinoma | 62 | 3DCRT, IMRT | 50.4/28 | Thrombocytopaenia (n=9), abdominal pain (n=4), thrombus/embolism (n=4), hypoalbuminuria (n=2), diarrhoea (n=1), elevated ALP (n=1), gastritis (n=1), SVT (n=1), enteritis (n=1), gastric ulcer (n=1), C-P arrest (n=1), other (n=105) | Colonic perforation (n=1) | NR | NR | NR | NR | |
Sohal et al. (47) | Pancreatic adenocarcinoma | 18 | Technique NR | 59.4/33 | Increased AST/ALT (n=1), hyperbilirubinaemia (n=1), pulmonary embolism (n=1), other (n=9) | NR | NR | NR | NR | NR | |
Small et al. (48) | Pancreatic adenocarcinoma | 29 | 3DCRT | 36/15 | Hyperbilirubinaemia (n=2), other (n=39) | NR | 23 | Other (n=10) | NR | 8 | |
Miyazawa et al. (49) | CRC | 1 | Technique NR | 50/25 | Other (n=1) | NR | 1 | NR | NR | NR | |
Barney et al. (50) | Various | 30 | SBRT | 50/5 | Gastric ulcer (n=3), gastric perforation (n=2), small bowel perforation (n=1), duodenal ulcer (n=1) | Duodenal perforation (n=1) | NR | NR | NR | NR | |
Pelvis | Vuky et al. (51) | Prostate | 18 | IMRT | 57–77.9/38 | Other (n=6) | NR | 6 | Proctitis (n=2), rectal bleeding (n=2), urinary retention (n=2), haematuria (n=1), prostatitis (n=1), cystitis (n=1), other (n=1) | NR | NR |
Head and neck | Fury et al. (52) | HNSCC | 30 | IMRT | 70/33–35 | Mucositis (n=18), dysphagia (n=10), pain in throat/pharynx/larynx (n=4), ALT elevation (n=2), thrombocytopaenia (n=1), other (n=78) | NR | NR | NR | NR | NR |
Ahn et al. (53) | Various | 13 | Technique NR | 70/30–36 | Mucositis (n=5), dysphagia (n=4), GI fistula/perforation (n=3), dermatitis/rash (n=3), thrombocytopaenia (n=1), other (n=14) | NR | NR | NR | NR | NR | |
Yoo et al. (54) | HNSCC | 28 | IMRT | 70/56 | Mucositis (n=14), dysphagia (n=8), thrombocytopaenia (n=2), radiation dermatitis (n=2), pneumonitis (n=2), cardiac ischaemia (n=1), other (n=29) | NR | NR | Osteoradionecrosis (n=4), soft tissue necrosis (n=1), fibrosis (n=2), parotiditis (n=1), dysphagia (n=2), other (n=6) | NR | NR | |
Yao et al. (55) | HNSCC | 30 | IMRT, conventional | IMRT 70/35; conventional 70.2/39 | Dysphagia (n=11), dermatitis (n=9), mucositis (n=7), other (n=41) | NR | NR | Pharyngoesophageal stenosis requiring dilatation (n=7), radiation necrosis of the larynx (n=1) | NR | NR | |
Argiris et al. (56) | HNSCC | 41 | 3DCRT, IMRT | 70–74/35 | Mucositis (n=17), dysphagia (n=12), radiation dermatitis (n=11), haemorrhage (n=3), osteonecrosis (n=1), GI perforation (n=1), wound complication (n=1), other (n=27) | NR | NR | NR | NR | NR | |
Salama et al. (57) | HNSCC | 17 | 3DCRT, IMRT | 73.8/50 | Mucositis (n=13), other (n=13) | Other (n=1) | NR | NR | NR | NR | |
Fury et al. (58) | HNSCC | 42 | IMRT | 70/33–35 | Functional mucositis (n=36), throat pain (n=31), clinical mucositis (n=9), ALT elevation (n=3), atrial fibrillation (n=2), AST elevation (n=2), dermatitis in radiation field (n=1), other (n=121) | NR | 42 | NR | NR | NR | |
Nyflot et al. (59) | HNSCC | 10 | Technique NR | 70/33 | Mucositis (n=8), dysphagia (n=8), skin reaction (n=1), oral pain (n=1), other (n=17) | NR | 10 | NR | NR | NR | |
Lee et al. (60) | Nasopharyngeal | 44 | IMRT | 70/33 | Radiation mucositis (n=34), dysphagia (n=24), mucositis/stomatitis (n=21), oral pain (n=8), pharyngolaryngeal pain (n=6), radiation recall syndrome (n=6), hearing impairment (n=4), neuralgia (n=4), increased ALT (n=4), increased AST (n=3), thrombocytopaenia (n=2), trismus (n=1), salivary gland disorder (n=1), osteonecrosis (n=1), oesophagitis (n=1), tinnitus (n=1), other (n=118) | NR | 44 | Dysphagia (n=5), hearing impairment (n=4), dry mouth (n=2), radiation mucositis (n=1), other (n=7) | NR | NR | |
Other | De Yao et al. (61) | Angiosarcoma | 1 | Technique NR | 60/30 | NR | NR | NR | NR | NR | NR |
Monk et al. (62) | Colonic adenocarcinoma | 1 | SBRT | 27/3 | NR | NR | 0 | NR | Oesophageal-meningeal fistula (n=1) | 1 | |
Pernin et al. (63) | Breast | 39 | Technique NR | 50/25 | Other (n=3) | NR | 3 | NR | NR | NR |
RT, radiotherapy; med, median; Fx, fractions; NSCLC, non-small cell lung cancer; NR, not recorded; CRC, colorectal cancer; LDRT, low dose radiotherapy; 3DCRT, 3D conformal radiotherapy; IMRT, intensity-modulated radiotherapy; ALP, alkaline phosphatase; SVT, supraventricular tachycardia; C-P arrest, cardiopulmonary; AST, aspartate transaminase; ALT, alanine transaminase; SBRT, stereotactic body radiotherapy; HNSCC, head and neck squamous cell carcinoma; HCC, hepatocellular carcinoma; GI, gastrointestinal.
Table 4
Treated site | Study | Primary tumour | N | RT type | RT (Gy), med/Fx | Acute grade 3–4 toxicities (n) | Acute grade 5 toxicities (n) | Patients who experienced acute severe toxicity (n) | Late grade 3–4 toxicities (n) | Late grade 5 toxicities, n | Patients who experienced late severe toxicity, n |
---|---|---|---|---|---|---|---|---|---|---|---|
Thyroid | Porcelli et al. (64) | Thyroid | 1 | 3DCRT | 38 Gy total | NR | NR | 0 | NR | NR | NR |
Liver | Wang et al. (65) | HCC | 35 | SBRT | 45–55/5–10 | Diarrhoea (n=1), abdominal pain (n=1), nausea and vomiting (n=1), ALT/AST elevation (n=1) | NR | NR | NR | NR | NR |
Liver | Yu et al. (66) | HCC | 28 | Photon-beam therapy, proton radiotherapy | 35/10 | Palmo-plantar erythema (n=1), abdominal pain (n=1) | NR | NR | Duodenal perforation requiring emergency surgery (n=1) | 0 | 1 |
RT, radiotherapy; med, median; Fx, fractions; 3DCRT, 3D conformal radiotherapy; NR, not recorded; HCC, hepatocellular carcinoma; SBRT, stereotactic body radiotherapy; ALT, alanine transaminase; AST, aspartate transaminase.
Table 5
Radiotherapy technique | Study | Primary tumour | Treated site | N/N (N) | Drug | RT type (n) | RT dose (Gy), med/Fx | Acute grade 3–4 toxicities (n) | Acute grade 5 toxicities (n) | Patients with severe toxicity, n |
---|---|---|---|---|---|---|---|---|---|---|
SBRT | Hiniker et al. (67) | Melanoma | Liver | 1 | Ipilimumab | SABR | 54/3 | Other (n=1) | NR | 1 |
Gutkin et al. (68) | Melanoma | Liver | 1 | Ipilimumab | SBRT | 54/3 | Other (n=1) (stage not specified) | NR | 1 | |
Chiang et al. (69) | HCC | Liver | 5 | Nivolumab (1 switched to pembrolizumab) | SBRT | 27.5–35/5 | Pneumonitis (n=1, occurring on pembrolizumab), other (n=1) | NR | 2 | |
Hermel et al. (70) | HCC | Porta-hepatic LN | 1 | Nivolumab | SBRT | NR | NR | NR | 0 | |
Xie et al. (71) | Pancreatic ductal adenocarcinoma | Various abdominal | 59 | Durvalumab or durvalumab and tremelimumab | SBRT | 8/1 or 25/5 | Colitis (n=3), other (n=20) | NR | 18 | |
Welsh et al. (72) | Various | Liver, lung | 106 | Ipilimumab | SABR | 50/4 or 60/10 | ALT/AST elevation (n=5), bilirubin elevation (n=2), colitis (n=2), pancreatitis/lipase elevation (n=1), pneumonitis (n=1), cholecystitis (n=1), chest pain (n=1), other (n=21) | NR | NR | |
McBride et al. (73) | HNSCC | Various | 32 (n=47) | Nivolumab | SBRT | 27/3 | n=3 not specified | NR | 3 | |
Theelen et al. (74) | NSCLC | Various | 36 | Pembrolizumab | SBRT | 24/3 | Other (n=16) | NR | 12 | |
Louvel et al. (75) | Melanoma, CRC | Spleen, liver, lung | 2 (n=3) | Pembrolizumab or atezolizumab | SBRT | 24/6; 45/3 | Radiation pneumonitis (n=2) | NR | 2 | |
Luke et al. (76) | Various | Various | 62 | Pembrolizumab | SBRT | 30–50/3–5 | Pneumonitis (n=3), colitis (n=2), hepatic toxicity (n=1), other (n=42) | NR | NR | |
HFRT | Welsh et al. (77) | NSCLC | Liver, lung | 20 | Pembrolizumab | SBRT (n=10), HFRT (n=10) | SBRT 50/4, HFRT 45/15 | Pneumonitis (n=1), other (n=5) | NR | 6 |
19 | SBRT | 50/4 | Pneumonitis (n=1), ventricular tachycardia (n=1), RV dysfunction (n=1), myocardial infarction (n=1) | NR | 3 | |||||
21 | HFRT | 45/15 | Pneumonitis (n=2), pleural effusion (n=1), atelectasis (n=1), pericardial effusion (n=1), other (n=2) | NR | 6 | |||||
Maity et al. (78) | Various | Various | 23 | Pembrolizumab | Various | 8/3 or 17/1 | Other (n=1) | NR | 1 | |
Monjazeb et al. (79) | CRC | Liver | 10 | Durvalumab and tremelimumab | HFRT | 24/3 | Other (n=5) | NR | 3 | |
Various/other RT technique | Monjazeb et al. (79) | CRC | Liver | 9 | Durvalumab and tremelimumab | LDFRT | 8/16 | Liver enzyme elevation (n=1), other (n=8) | NR | 5 |
Hiniker et al. (80) | Melanoma | Various | 22 (n=27) | Ipilimumab | IMRT (n=6), 3DCRT (n=9), SBRT (n=8) | IMRT 40/10–15, 3DCRT 33/5–15, SBRT 24.5/1–5 | Colitis (n=2), other (n=1) | NR | 3 | |
Zhong et al. (81) | HCC | Various | 16 | Various | HFRT (n=7), SBRT (n=6), conventional (n=3) | 30–60 HFRT (n=7), SBRT (n=6), conventional (n=3) | ALT elevation (n=1), AST elevation (n=1), GI haemorrhage (n=1), other (n=1) | NR | 4 | |
Tang et al. (82) | Various | Liver, lung | 38 | Ipilimumab | HFRT | HFRT 24/3, SABR 50/4 or 60/10 | Colitis (n=4), ALT/AST elevation (n=1), other (n=16) | NR | 12 | |
Levy et al. (83) | Various | Various | 10 (n=15) | Durvalumab | 3DCRT (n=12), SBRT (n=3) | Various | NR | NR | 0 | |
Amagai et al. (84) | Melanoma | Various abdominopelvic | 3 | Nivolumab, ipilimumab | IMRT | 37.5/15; 60/15; 30/10 | Other (n=1) | NR | 1 | |
Golden et al. (85) | NSCLC | Liver | 1 | Ipilimumab | Technique NR | 30/5 | NR | NR | 0 |
RT, radiotherapy; med, median; Fx, fractions; SBRT, stereotactic body radiotherapy; SABR, stereotactic ablative radiotherapy; NR, not recorded; HCC, hepatocellular carcinoma; ALT, alanine transaminase; AST, aspartate transaminase; HNSCC, head and neck squamous cell carcinoma; NSCLC, non-small cell lung cancer; CRC, colorectal cancer; HFRT, hypofractionated radiotherapy; RV, right ventricle; LDFRT, low dose fractionated radiotherapy; IMRT, intensity-modulated radiotherapy; 3DCRT, 3D conformal radiotherapy; GI, gastrointestinal.
Mechanisms of RT activity
RT is a key therapeutic for a multitude of tumour types, both as a key therapy for newly diagnosed cancer patients, as an adjuvant or neoadjuvant for surgery or as a palliative modality. The development of new image guidance technologies and radiation delivery techniques has allowed for increased precision and an evolving capability to maximise dose conformity (86).
Conventional fractionation external beam radiotherapy (EBRT) typically involves delivering a series of low fractions of 1–5 Gy per fraction. The rationale for this is based on the premise that lower fraction sizes are associated with lower toxicity, necessitating the need for extended courses of small fraction size to achieve therapeutic efficacy (87). However as can be expected, applying the same regime to all patients with all tumour types does not result in the same outcome due to the differences in pathological types, differentiation and radiobiological behaviour of tumours (88). The responses of tumour and normal tissues to conventional RT is governed by the five Rs of radiobiology (89), the first four of which were initially described by Withers, including “repair of sublethal cellular damage”, “redistribution of cells within the cell cycle”, “reoxygenation of the surviving cells”, and “repopulation of cells after radiation” (90). In conventionally fractionated EBRT, radiosensitivity of tumour cells is increased by “reoxygenation” and “redistribution”, while “repair” and “repopulation” of tumour cells contributes to decreased radiosensitivity (91), with “radiosensitivity” becoming the fifth ‘R’ (89).
The advent of new radiation delivery techniques has resulted in a major shift in RT practice. In contrast to conventionally fractionated EBRT, stereotactic radiosurgery (SRS) and stereotactic body radiotherapy (SBRT) allow for precision, high dose-per-fraction RT to be delivered to tumours while reducing the damage to surrounding tissues (87). With SBRT, higher dose-per fraction of radiation means that the framework of the 4 Rs insufficient, as the majority of the tumour cells are ablated (91). Additional radiobiological alterations induced by SBRT, such as vascular endothelial injury and immune cell activation enhance the anti-tumour effect, and could potentiate the efficacy of targeted therapies (88).
Sorafenib and RT
Sorafenib is a small molecule TKI which targets Raf kinase and the vascular endothelial growth factor receptor (VEGFR) intracellular kinase pathway (Figure 1) (92). The main toxicities associated with sorafenib are hand-foot skin reaction (also known as palmo-plantar erythrodysaesthesia, or PPE), diarrhoea, hypertension, rash, fatigue, abdominal pain and nausea. Severe toxicity such as liver failure and myocardial infarction are uncommon (93). The biological half-life of around 20–27 hours, and is metabolised by the liver (94). The proposed mechanism of sorafenib interaction with RT are inhibition are two-fold. Firstly, inhibition of pro-growth RAS-RAF-MAPK pathway can increase radiation sensitivity, and secondarily, VEGFR pathway inhibition leads to normalisation of tumour vasculature leading to increased oxygenation (95,96).
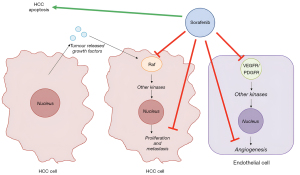
Toxicity of combination sorafenib and RT
A search of the literature found 22 studies where sorafenib was combined with RT (19-40). There were three cohort studies and two case reports where sorafenib was combined with liver SBRT (Table 2), within which the rate of grade ≥3 toxicity ranged from 20–67%. A number of trials noted that combination sorafenib and liver RT was a difficult regime for patients due to high rates of toxicity (20,21). Additionally, there were five cohort studies and two case reports reporting conventionally fractionated liver RT or liver intensity-modulated radiotherapy (IMRT) with sorafenib (Table 2). In these studies, the toxicity rate ranged from 29–36%. As will be discussed below, the most concerning adverse effects in these studies with liver RT were the high rate of liver and gastrointestinal (GI) toxicity. There were four acute grade 5 hepatic toxicities reported with combination sorafenib and whole liver radiotherapy (WLRT) (21,22), and two grade 5 GI toxicities with combination sorafenib and SBRT, including one bowel perforation (26), and one GI haemorrhage (20).
Sorafenib was combined with extrahepatic RT in six cohort studies and eight case reports (Table 2), with rates of toxicity ranging between 0–28% across fourteen cohort studies. The most common adverse effect seen with sorafenib in combination with extra-hepatic radiation was thrombocytopaenia.
Four studies (Table 2) reported late toxicities, all with combination sorafenib and conventionally fractionated RT. There were 32 late grade ≥3 toxicities possibly attributable to RT (0–84%) (Table 2). Most common were severe GI toxicity (including bleeding, ulcers and perforations) (0–48%), and hepatotoxicity, occurring at a rate of 25% in one study (23). There were four late grade 5 toxicities (three hepatotoxicities, one GI bleed).
The most commonly observed side effect of TKIs with RT was thrombocytopenia (0–28%). It was found at a rate of 4% with sorafenib alone in the Sorafenib Hepatocellular Carcinoma Assessment Randomized Protocol (SHARP) trial (3). Thrombocytopenia is an uncommon side effect of SBRT to the liver, and has not been found in other studies incorporating those with normal liver function (91-93). In those with primary liver cancer, the rate varies based upon baseline liver fibrosis, from 2–9% Charles-Pugh A (CPA) cirrhosis (94,95) to 11–14% for those with Charles-Pugh B (CPB) cirrhosis (96,97). Therefore, we attributed this primarily to the systemic therapy.
Hepatotoxicity with sorafenib and RT
Of great concern is the high rate of severe liver toxicity reported in trials combining sorafenib with liver RT. In a phase 2 study of combined sorafenib and RT in patients with HCC, the authors made particular note that hepatic toxicities would be a major determinant of safety in combination therapy (23). This seems to be reflected across multiple different trials, with rates of liver toxicity ranging from 0–19% in liver SBRT cohorts and 3–18% with conventionally fractionated liver RT cohorts (20-24,26). These rates appear to be higher than in sorafenib alone. Neither the SHARP trial nor Asia-Pacific studies of sorafenib in patients with underlying liver dysfunction reported deaths from liver failure (5,6). There have been case reports of grade 3–5 sorafenib-induced liver toxicity (97-99) and one death from liver failure in a phase II trial examining the use of sorafenib in thyroid cancer (100); however the incidences seen in the combination setting appear higher. The rate of hepatotoxicity also appears higher compared with radiation alone, where the risk of radiation induced liver disease is 5–10% when the whole liver is irradiated up to 30–35 Gy (101). In comparison, rates of 13.3% and 18.2% were seen with combination therapy when the whole liver was irradiated to 21.6 Gy (21,22). Interestingly, the volume of liver irradiated seems to play a role in the level of toxicity. In one study, significant toxicity was observed when higher volumes of liver tissue were irradiated [Response Evaluation Criteria in Solid Tumours (RECIST) target size 87 vs. 35 mm], including three grade 3–4 liver enzyme elevations (20). WLRT was compared to liver SBRT in two studies and was associated with four deaths due to hepatotoxicity between the two studies (13–18% of patients), compared to no severe hepatotoxicity in the SBRT groups (21,22), suggesting a volume effect when combining liver RT with sorafenib. It may be that sorafenib alone is associated with hepatotoxicity which is increased by RT in a dose dependent way. The concept of RT dose-volume tolerance for hepatotoxicity is well elucidated, with multivariate analysis showing that mean liver dose is an important predictor of radiation induced liver disease (102,103). The results of these combination studies suggest an augmentation of that effect when combined with TKI.
GI toxicity with sorafenib and RT
Another concern was the high occurrence of GI bleeding and perforation. The rate of severe GI toxicity ranged from 0–19% in the acute setting and 0–48% in the late setting, as well as multiple grade 5 bowel perforations. Significant GI toxicities are not a known side effect of sorafenib alone. In the SHARP trial, sorafenib caused no significant increase in tumour bleeding compared to the placebo (5). The rates seen in combination trials also appear higher than attributable to RT alone, where a severe form of radiation-induced bowel injury has been reported in 4–8% of patients who undergo abdominal or pelvic RT within 5–10 years (104). It is possible that dose per fraction may play a role in GI toxicity, as shown in previous trials of liver SBRT alone where luminal toxicities have been observed in 2 of 102 patients (105). This would explain why the highest rate of acute GI toxicity was seen in a study reporting sorafenib with liver SBRT (20). In contrast, other studies with conventionally fractionated liver RT observed no severe GI toxicity (21-23). However, it is important to note that severe GI toxicity was also observed in studies where palliative RT doses were used, including grade 5 toxicities (24,26,38). One case study reported sigmoid colon perforation in two patients after 4 weeks of combination sorafenib and palliative RT, one grade 3 and one grade 5 (38). This implies that the dose per fraction of RT may not be the only contributing factor in GI toxicity, and that sorafenib may have a radio-sensitising effect increasing the risk of GI perforation.
Regorafenib and RT
Second line therapy for HCC includes regorafenib, a TKI and VEGFR inhibitor which has been shown to improve median survival compared with placebo after progression on sorafenib (8). Combination regorafenib and RT was examined in only one case study (41), which reported no severe toxicities (41). We maintain there is insufficient data to draw meaningful conclusions regarding this combination.
RT and bevacizumab
Bevacizumab is a humanized anti-VEGF monoclonal IgG1 antibody that inhibits the binding of circulating VEGF to its receptor, thereby reducing tumour microvascular growth (Figure 2) (106). Bevacizumab has an in vivo half-life of approximately 19 days and is eliminated by proteolytic catabolism rather than hepatic or renal clearance. Common side effects seen are hypertension, proteinuria, thromboembolic events, GI perforation, and wound healing complications. Inhibition of VEGF has been shown to potentiate the effects of RT in pre-clinical studies in an additive or synergistic fashion (107-110). Mechanisms of interaction are incompletely understood but relate to normalisation of tumour vasculature leading to increased oxygenation, or maximising tumour microvascular damage in irradiated tissues (107). Severe toxicity may occur in the context of impaired wound healing associated with bevacizumab.
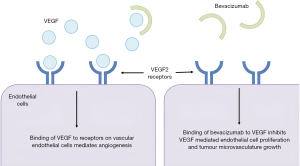
Toxicity of combination bevacizumab and RT
We found 22 studies reporting combination bevacizumab and RT (Table 3) (42-63), and found that this combination was associated with a high rate of severe adverse events occurring in many studies, ranging from 0–27% in the acute setting and 0–23% in the late setting. While most of these adverse effects occurred outside the radiation field and were likely not related to combination therapy, there was a high rate of severe thrombocytopaenia, GI and intra-luminal toxicities. In the two cohort studies where bevacizumab was combined with thoracic RT, the most common adverse effect was oesophagitis (0–29%). With abdominal RT and bevacizumab (six cohort studies, one case report), the most common toxicities were thrombocytopaenia (0–15%) and peptic ulcer (0–23%). There were two grade 5 toxicities, one colonic perforation (46), and one duodenal perforation (50). In one study, pelvic RT combined with bevacizumab was associated with six acute grade 3–4 toxicities, none within the radiation field (51).
Head and neck RT with bevacizumab (nine studies, N=255) was associated with 371 grade ≥3 adverse events within the radiation field, most commonly mucositis (23–98%) and dysphagia (0–80%) (Table 3). There was one case of grade 5 sepsis not attributed to RT. This incidence of mucositis and dysphagia appears consistent with known incidences with RT alone. Mucositis has been observed in 34% of patients receiving conventionally fractionated RT (111). In the Sydney Swallow Questionnaire, 59% of patients who received head and neck RT experienced persistent dysphagia post-RT (112).
GI toxicity with bevacizumab and RT
The rate of GI toxicity, including perforation and bleeding, associated with combination bevacizumab and RT is of great concern. We observed a higher-than-expected level of severe GI toxicity with combination bevacizumab and RT, ranging from 0–27% in the acute setting and 0–23% in the late setting. Bevacizumab is associated with GI perforation, with the incidence ranging between 0–3.9% in a published meta-analysis (113). This is a rare but serious side effect of RT that is dependent on the peak dose received by the GI lumen, the organ irradiated, and the volume. However, most common protocols are designed to accept less than a 5% risk of grade 3 adverse toxicity (114). Concerningly, the rates with combination therapy were higher than with monotherapy, suggesting a synergistic effect leading to increased luminal toxicity.
Interestingly, the highest rate of severe GI toxicity was seen in a retrospective analysis where 27% of patients who received bevacizumab with abdominal SBRT experienced a severe luminal adverse event (50). In comparison, the rates of acute GI toxicity seen in studies using conventionally fractionated abdominal RT was lower (0–3%, Table 3), suggesting a role for dose-per-fraction in severe GI toxicity. One possible explanation is that RT induced bowel injury is mediated by microvascular damage (115), which may be more significant when larger fractions are employed (116). This would also explain the increased toxicity compared to monotherapy, as inhibition of VEGF pathways would impair recovery of radiation-induced vascular damage. This synergistic effect of VEGF inhibition and RT damage to bowel microvasculature by RT results in increased risk of severe GI toxicity with combined anti-angiogenic therapy with RT.
RT and lenvatinib
Lenvatinib is an inhibitor of VEGFR 1 to 3 as well as fibroblast growth factor receptors (FGFRs) 1 to 4, platelet-derived growth factor receptor (PDGFR) alpha, RET and KIT, and is an alternate first line therapy option for HCC (Figure 3) (7). Similar to sorafenib, lenvatinib increases radiosensitivity by inducing tumour vessel normalisation (117). Compared to sorafenib, lenvatinib has shown to have a stronger inhibitory effect on VEGF and FGFR signalling (118), however there is limited data related to the safety and efficacy of lenvatinib when in combination with RT. Lenvatinib has an in vivo half-life of approximately 28 hours, and is eliminated primarily through enzymatic metabolism through Cytochrome P450 3A (CYP3A) and aldehyde oxidase. Common adverse effects include hypertension, peripheral oedema, proteinuria, fatigue, palmo-plantar erythema, hyper and hypothyroidism, hepatotoxicity, haemorrhage and GI toxicities including nausea, vomiting, diarrhoea, and less commonly, fistula formation and perforation.
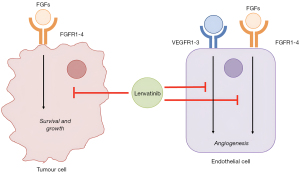
Toxicity of combination levatinib and RT
Our review of the literature revealed two small retrospective cohort studies where lenvatinib was combined with RT for HCC (Table 4) (65,66). In one study, lenvatinib with liver SBRT (n=35) was associated with four grade 3–4 toxicities (11%) including diarrhoea, nausea and vomiting, abdominal pain, and hepatotoxicity (65). In a retrospective study which examined combination lenvatinib and RT in HCC with macroscopic tumour thrombosis, combination therapy (n=28) was associated with just two grade 3–4 adverse effects (7.1% of patients), palmo-plantar erythema and abdominal pain. Additionally, there was one patient who experienced a duodenal perforation 4 months after completion of combination therapy (66). No grade 5 toxicities were seen in either cohort study.
In these two cohort studies, combination RT and lenvatinib seems to be well tolerated. Due to the paucity of clinical data about combination lenvatinib and RT and the small sample sizes in retrospective studies, minimal meaningful conclusions can be drawn about its safety profile in combination therapy. There was also one case report where lenvatinib was combined with thyroid RT with no severe adverse events (64).
RT and immune checkpoint lockade
Immune checkpoint inhibitors such as atezolizumab and nivolumab enhance systemic immune responses against cancer cells through blockade of immune modulators such as PD-L1 or programmed cell death 1 (PD-1) (Figure 4). Monoclonal antibodies are eliminated via proteolytic catabolism, and have long half-lives, with atezolizumab having an in vivo half-life of 27 days, and nivolumab 25 days (119). Therefore, ceasing atezolizumab or other immune checkpoint inhibitors prior to RT may not prevent any interactions between the two agents if the clinical imperative to give RT is urgent. The postulated method of interaction with RT and immunotherapy is to increase antigen presentation to the immune system, as well as increase local immune responses within the irradiated volume. In favourable cases this results in a prolonged and durable anti-tumour immune response that is associated with sustained tumour regression (120).
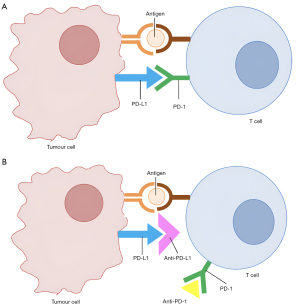
Toxicity of combination immune checkpoint inhibition and RT
Nineteen studies were identified in which immunotherapy was given in combination with intrahepatic RT in at least one patient (Table 5) (67-85), however given that the majority of the studies reported RT to multiple anatomical sites it is difficult to analyse toxicities according to treatment site. Combination therapy with immune checkpoint blockade and RT was well tolerated, with multiple studies reporting that the safety profile of combination therapy was consistent with that of monotherapy. No grade 5 toxicities were recorded (Table 5). The most common grade 3–4 toxicities possibly associated with RT were elevation of liver enzymes (0–14%), colitis (0–11%) and pneumonitis (0–10%). Outside the radiation field, the most common severe toxicities were GI and haematological in nature. were eight cases of pituitary hypophysitis. Colitis and pneumonitis occurred at similar rates seen in monotherapy with RT or immune checkpoint blockade (121-124). This is in contrast to the PACIFIC trial where rates of pneumonitis were higher when durvalumab was given after chemoradiotherapy (125). However, the pneumonitis was primarily low grade, which may explain the discrepancy with this review (125). Pituitary hypophysitis is a well-known adverse event of immune checkpoint blockade, particularly with ipilimumab and to a lesser extent in nivolumab and pembrolizumab (126,127).
Among the included cohort studies, durvalumab and tremelimumab with RT was associated with the greatest frequency of severe adverse events, with 0–56% of patients experiencing grade 3–4 toxicity, followed by 4–33% of patients treated with pembrolizumab and RT, 14–32% of patients treated with ipilimumab and RT and 9% of patients treated with nivolumab and RT. Atezolizumab with RT was only reported in one patient. The toxicity rates of SBRT and hypofractionated radiotherapy (HFRT) with immune checkpoint inhibitors were similar, however, as many of the studies included multiple different RT techniques used in a variety of different sites, and mostly for the treatment of metastases, minimal conclusions can be drawn about the differences in safety profile between RT techniques, or between the different immunotherapeutic agents.
It should be noted that immuno-oncological agents are known to cause delayed immune-related adverse events, including following discontinuation of the drug (128,129). While none of the included studies combining immunotherapy and RT reported any late onset adverse events, they may occur in the future as more patients are treated with combination therapy.
The retrospective case analysis by Zhong et al. should be considered independently, as it examined the safety and outcomes of immune checkpoint blockade when combined with both RT and various target anti-angiogenic agents. It reported triple therapy to be well tolerated, with no significant increase in toxicity compared to monotherapy with immune checkpoint blockade or anti-angiogenic agents alone (81). Grade 3–4 adverse events occurred in 4 patients (25%), and included rash, aspartate transaminase (AST) elevation, alanine transaminase (ALT) elevation and GI haemorrhage (81). The incidence of severe toxicity is consistent, if not somewhat lower than the incidence of adverse events occurring on combination therapy with immunotherapy and RT.
Strengths and limitations
This review provides a comprehensive summary of the currently available data about the toxicity of with RT to the liver and other body sites when combined with the systemic targeted therapies that are used to treat advanced HCC. By expanding the literature search to non-HCC patients receiving RT in combination with systemic therapies commonly used for the treatment of advanced HCC we have been able to begin to examine the toxicity associated with combination therapy despite the paucity of studies with HCC patients. However, while the data can be extrapolated, more studies are needed in the setting of HCC to better understand the safety profile of combination therapy in HCC.
This review has a number of other limitations including a lack of comparators with monotherapy, meaning no quantitative analysis could be reliably performed regarding differences in safety profiles between combination and monotherapy can be drawn. Another limitation is the quality of some of the included articles, while the majority of included articles were cohort studies, due to the scarcity of published data about combination therapy a number of case studies were also included. As a consequence, significant adverse events may be over-represented. Finally, given that there was a great variation in the included articles in timings of treatment regimens and dosing strategies, minimal conclusions can be drawn about the effects of changing timing or dose of therapy. Not all the included articles used palliative RT dosing/fractionation, or did not report the volume of liver receiving high doses of RT, so the toxicity profile of palliative RT may vary from what has been reported here.
Conclusions
While a number of the adverse effects seen in combination therapy appear to be similar to those that are known to occur in monotherapy, we also identified a number of severe toxicities that appear to occur with greater frequency in combination therapy, including severe hepatotoxicity where sorafenib is combined with RT, and GI toxicity with combination RT and bevacizumab. Therefore, caution should be taken when combining sorafenib and bevacizumab with RT given the potential increased risk of severe hepatotoxicity and GI toxicity respectively. While lenvatinib seems to be well tolerated in combination with RT, there is insufficient evidence to draw any meaningful conclusions about its safety profile.
If combination therapy is used, the volume of irradiated tissue should be minimised, and where possible techniques such as SBRT should be used, although it is important to be aware of the possible increased GI toxicity with larger radiation doses per fraction. Additionally, the biological half-life of the systemic agent should be considered when administering RT so as to further reduce the risk of significant adverse events. RT was mostly well tolerated when combined with immunotherapy, with no severe toxicities increased risk compared to monotherapy. It must also be noted that late onset toxicities can be observed with systemic and immunotherapeutic agents in combination with RT as they can be with systemic therapy alone, so caution is advised at all time points during combination therapy. This review summarises much of the current knowledge on toxicity of RT when combined with targeted agents, however further research reporting safety data is required in this rapidly growing field.
Acknowledgments
The authors would like to thank Associate Professor Richard Khor, the Department of Radiation Oncology at Austin Health, the Austin Health Clinical School, and the MD Research Project team at the Melbourne Medical School for their guidance in the synthesis of this review.
Funding: None.
Footnote
Reporting Checklist: The authors have completed the Narrative Review reporting checklist. Available at https://cco.amegroups.com/article/view/10.21037/cco-23-140/rc
Peer Review File: Available at https://cco.amegroups.com/article/view/10.21037/cco-23-140/prf
Conflicts of Interest: All authors have completed the ICMJE uniform disclosure form (available at https://cco.amegroups.com/article/view/10.21037/cco-23-140/coif). The authors have no conflicts of interest to declare.
Ethical Statement: The authors are accountable for all aspects of the work in ensuring that questions related to the accuracy or integrity of any part of the work are appropriately investigated and resolved.
Open Access Statement: This is an Open Access article distributed in accordance with the Creative Commons Attribution-NonCommercial-NoDerivs 4.0 International License (CC BY-NC-ND 4.0), which permits the non-commercial replication and distribution of the article with the strict proviso that no changes or edits are made and the original work is properly cited (including links to both the formal publication through the relevant DOI and the license). See: https://creativecommons.org/licenses/by-nc-nd/4.0/.
References
- McGlynn KA, Petrick JL, El-Serag HB. Epidemiology of Hepatocellular Carcinoma. Hepatology 2021;73:4-13. [Crossref] [PubMed]
- Lee MS, Ryoo BY, Hsu CH, et al. Atezolizumab with or without bevacizumab in unresectable hepatocellular carcinoma (GO30140): an open-label, multicentre, phase 1b study. Lancet Oncol 2020;21:808-20. [Crossref] [PubMed]
- Finn RS, Qin S, Ikeda M, et al. Atezolizumab plus Bevacizumab in Unresectable Hepatocellular Carcinoma. N Engl J Med 2020;382:1894-905. [Crossref] [PubMed]
- Galle PR, Finn RS, Qin S, et al. Patient-reported outcomes (PROs) from the Phase III IMbrave150 trial of atezolizumab (atezo) + bevacizumab (bev) vs sorafenib (sor) as first-line treatment (tx) for patients (pts) with unresectable hepatocellular carcinoma (HCC). J Clin Oncol 2020;38:476. [Crossref]
- Llovet JM, Ricci S, Mazzaferro V, et al. Sorafenib in advanced hepatocellular carcinoma. N Engl J Med 2008;359:378-90. [Crossref] [PubMed]
- Cheng AL, Kang YK, Chen Z, et al. Efficacy and safety of sorafenib in patients in the Asia-Pacific region with advanced hepatocellular carcinoma: a phase III randomised, double-blind, placebo-controlled trial. Lancet Oncol 2009;10:25-34. [Crossref] [PubMed]
- Kudo M, Finn RS, Qin S, et al. Lenvatinib versus sorafenib in first-line treatment of patients with unresectable hepatocellular carcinoma: a randomised phase 3 non-inferiority trial. Lancet 2018;391:1163-73. [Crossref] [PubMed]
- Bruix J, Qin S, Merle P, et al. Regorafenib for patients with hepatocellular carcinoma who progressed on sorafenib treatment (RESORCE): a randomised, double-blind, placebo-controlled, phase 3 trial. Lancet 2017;389:56-66. [Crossref] [PubMed]
- El-Khoueiry AB, Sangro B, Yau T, et al. Nivolumab in patients with advanced hepatocellular carcinoma (CheckMate 040): an open-label, non-comparative, phase 1/2 dose escalation and expansion trial. Lancet 2017;389:2492-502. [Crossref] [PubMed]
- Zhu AX, Finn RS, Edeline J, et al. Pembrolizumab in patients with advanced hepatocellular carcinoma previously treated with sorafenib (KEYNOTE-224): a non-randomised, open-label phase 2 trial. Lancet Oncol 2018;19:940-52. [Crossref] [PubMed]
- Chen CP. Role of Radiotherapy in the Treatment of Hepatocellular Carcinoma. J Clin Transl Hepatol 2019;7:183-90. [Crossref] [PubMed]
- Hecht M, Zimmer L, Loquai C, et al. Radiosensitization by BRAF inhibitor therapy-mechanism and frequency of toxicity in melanoma patients. Ann Oncol 2015;26:1238-44. [Crossref] [PubMed]
- Ko EC, Raben D, Formenti SC. The Integration of Radiotherapy with Immunotherapy for the Treatment of Non-Small Cell Lung Cancer. Clin Cancer Res 2018;24:5792-806. [Crossref] [PubMed]
- Kroeze SG, Fritz C, Hoyer M, et al. Toxicity of concurrent stereotactic radiotherapy and targeted therapy or immunotherapy: A systematic review. Cancer Treat Rev 2017;53:25-37. [Crossref] [PubMed]
- Guan H, Zhou Z, Hou X, et al. Safety and potential increased risk of toxicity of radiotherapy combined immunotherapy strategy. Asia Pac J Clin Oncol 2023;19:35-50. [Crossref] [PubMed]
- Trapani S, Manicone M, Sikokis A, et al. Effectiveness and safety of “real” concurrent stereotactic radiotherapy and immunotherapy in metastatic solid tumors: a systematic review. Critical Reviews in Oncology/Hematology 2019;142:9-15. [Crossref] [PubMed]
- Niyazi M, Harter PN, Hattingen E, et al. Bevacizumab and radiotherapy for the treatment of glioblastoma: brothers in arms or unholy alliance? Oncotarget 2016;7:2313-28. [Crossref] [PubMed]
- National Cancer Institute. Common Terminology Criteria for Adverse Events (CTCAE) v5.0. National Cancer Institute; 2017. Available online: https://ctep.cancer.gov/protocolDevelopment/electronic_applications/ctc.htm#ctc_50
- Hsieh CH, Lin SC, Shueng PW, et al. Recall radiation dermatitis by sorafenib following stereotactic body radiation therapy. Onco Targets Ther 2014;7:1111-4. [Crossref] [PubMed]
- Brade AM, Ng S, Brierley J, et al. Phase 1 Trial of Sorafenib and Stereotactic Body Radiation Therapy for Hepatocellular Carcinoma. Int J Radiat Oncol Biol Phys 2016;94:580-7. [Crossref] [PubMed]
- Goody RB, Brade AM, Wang L, et al. Phase I trial of radiation therapy and sorafenib in unresectable liver metastases. Radiother Oncol 2017;123:234-9. [Crossref] [PubMed]
- Brade AM, Kim J, Brierley J, et al. Phase I Study of Sorafenib and Whole-liver Radiation Therapy (WLRT) or Stereotactic Body Radiation Therapy (SBRT) for Liver Metastases. Int J Radiat Oncol Biol Phys 2012;84:S11-2. [Crossref]
- Chen SW, Lin LC, Kuo YC, et al. Phase 2 study of combined sorafenib and radiation therapy in patients with advanced hepatocellular carcinoma. Int J Radiat Oncol Biol Phys 2014;88:1041-7. [Crossref] [PubMed]
- Kim BK, Kim DY, Byun HK, et al. Efficacy and Safety of Liver-Directed Concurrent Chemoradiotherapy and Sequential Sorafenib for Advanced Hepatocellular Carcinoma: A Prospective Phase 2 Trial. Int J Radiat Oncol Biol Phys 2020;107:106-15. [Crossref] [PubMed]
- Kim DH, Cho E, Cho SB, et al. Complete response of hepatocellular carcinoma with right atrium and pulmonary metastases treated by combined treatments (a possible treatment effect of natural killer cell): A case report and literature review. Medicine (Baltimore) 2018;97:e12866. [Crossref] [PubMed]
- Murray L, Longo J, Wan J, et al. Phase I dose escalation study of concurrent palliative radiation therapy with sorafenib in three anatomical cohorts (Thorax, Abdomen, Pelvis): The TAP study. Radiother Oncol 2017;124:74-9. [Crossref] [PubMed]
- Horgan AM, Dawson LA, Swaminath A, et al. Sorafenib and radiation therapy for the treatment of advanced hepatocellular carcinoma. J Gastrointest Cancer 2012;43:344-8. [Crossref] [PubMed]
- Aparicio J, García-Mora C, Martín M, et al. A phase I, dose-finding study of sorafenib in combination with gemcitabine and radiation therapy in patients with unresectable pancreatic adenocarcinoma: a Grupo Español Multidisciplinario en Cáncer Digestivo (GEMCAD) study. PLoS One 2014;9:e82209. [Crossref] [PubMed]
- Chiorean EG, Schneider BP, Akisik FM, et al. Phase 1 pharmacogenetic and pharmacodynamic study of sorafenib with concurrent radiation therapy and gemcitabine in locally advanced unresectable pancreatic cancer. Int J Radiat Oncol Biol Phys 2014;89:284-91. [Crossref] [PubMed]
- Cai ZS, Chen MJ, Tang TY, et al. Duodenum perforated after combination with sorafenib and radiotherapy for retroperitoneal lymph node metastasis of hepatocellular carcinoma. J Formos Med Assoc 2020;119:760-2. [Crossref] [PubMed]
- Arneson K, Mondschein J, Stavas M, et al. A phase I trial of concurrent sorafenib and stereotactic radiosurgery for patients with brain metastases. J Neurooncol 2017;133:435-42. [Crossref] [PubMed]
- Morikawa A, Grkovski M, Patil S, et al. A phase I trial of sorafenib with whole brain radiotherapy (WBRT) in breast cancer patients with brain metastases and a correlative study of FLT-PET brain imaging. Breast Cancer Res Treat 2021;188:415-25. [Crossref] [PubMed]
- Hottinger AF, Ben Aissa A, Espeli V, et al. Phase I study of sorafenib combined with radiation therapy and temozolomide as first-line treatment of high-grade glioma. Br J Cancer 2014;110:2655-61. [Crossref] [PubMed]
- Den RB, Kamrava M, Sheng Z, et al. A phase I study of the combination of sorafenib with temozolomide and radiation therapy for the treatment of primary and recurrent high-grade gliomas. Int J Radiat Oncol Biol Phys 2013;85:321-8. [Crossref] [PubMed]
- Chen KH, Weng MT, Chou YH, et al. Epigastric Distress Caused by Esophageal Candidiasis in 2 Patients Who Received Sorafenib Plus Radiotherapy for Hepatocellular Carcinoma: Case Report. Medicine (Baltimore) 2016;95:e3133. [Crossref] [PubMed]
- Kitajima T, Hatano E, Mitsunori Y, et al. Complete pathological response induced by sorafenib for advanced hepatocellular carcinoma with multiple lung metastases and venous tumor thrombosis allowing for curative resection. Clin J Gastroenterol 2015;8:300-5. [Crossref] [PubMed]
- Stieb S, Riesterer O, Brüssow C, et al. Radiation recall dermatitis induced by sorafenib : A case study and review of the literature. Strahlenther Onkol 2016;192:342-8. [Crossref] [PubMed]
- Inoue T, Kinoshita H, Komai Y, et al. Two cases of gastrointestinal perforation after radiotherapy in patients receiving tyrosine kinase inhibitor for advanced renal cell carcinoma. World J Surg Oncol 2012;10:167. [Crossref] [PubMed]
- Kashihara T, Murakami N, Iizumi S, et al. Hemorrhage from Ascending Colon and Gluteal Muscle Associated with Sorafenib and Radiation Therapy: Radiation Dose Distribution Corresponded with Colonoscopy Findings and Computed Tomography Images. Pract Radiat Oncol 2019;9:214-9. [Crossref] [PubMed]
- Mehta K, Kaubisch A, Tang J, et al. Radiation Recall Dermatitis in Patients Treated with Sorafenib. Case Rep Oncol Med 2018;2018:2171062. [Crossref] [PubMed]
- Roberto M, Falcone R, Mazzuca F, et al. The role of stereotactic body radiation therapy in oligometastatic colorectal cancer: Clinical case report of a long-responder patient treated with regorafenib beyond progression. Medicine (Baltimore) 2017;96:e9023. [Crossref] [PubMed]
- Lind JS, Senan S, Smit EF. Pulmonary toxicity after bevacizumab and concurrent thoracic radiotherapy observed in a phase I study for inoperable stage III non-small-cell lung cancer. J Clin Oncol 2012;30:e104-8. [Crossref] [PubMed]
- Socinski MA, Stinchcombe TE, Moore DT, et al. Incorporating bevacizumab and erlotinib in the combined-modality treatment of stage III non-small-cell lung cancer: results of a phase I/II trial. J Clin Oncol 2012;30:3953-9. [Crossref] [PubMed]
- Morganti AG, Cellini F, Mignogna S, et al. Low-dose radiotherapy and concurrent FOLFIRI-bevacizumab: a Phase II study. Future Oncol 2016;12:779-87. [Crossref] [PubMed]
- Chadha AS, Skinner HD, Gunther JR, et al. Phase I Trial of Consolidative Radiotherapy with Concurrent Bevacizumab, Erlotinib and Capecitabine for Unresectable Pancreatic Cancer. PLoS One 2016;11:e0156910. [Crossref] [PubMed]
- Berlin JD, Feng Y, Catalano P, et al. An Intergroup Randomized Phase II Study of Bevacizumab or Cetuximab in Combination with Gemcitabine and in Combination with Chemoradiation in Patients with Resected Pancreatic Carcinoma: A Trial of the ECOG-ACRIN Cancer Research Group (E2204). Oncology 2018;94:39-46. [Crossref] [PubMed]
- Sohal DP, Metz JM, Sun W, et al. Toxicity study of gemcitabine, oxaliplatin, and bevacizumab, followed by 5-fluorouracil, oxaliplatin, bevacizumab, and radiotherapy, in patients with locally advanced pancreatic cancer. Cancer Chemother Pharmacol 2013;71:1485-91. [Crossref] [PubMed]
- Small W Jr, Mulcahy MF, Rademaker A, et al. Phase II trial of full-dose gemcitabine and bevacizumab in combination with attenuated three-dimensional conformal radiotherapy in patients with localized pancreatic cancer. Int J Radiat Oncol Biol Phys 2011;80:476-82. [Crossref] [PubMed]
- Miyazawa T, Ebe K, Koide N, et al. Complete Response of Isolated Para-aortic Lymph Node Recurrence from Rectosigmoid Cancer Treated by Chemoradiation Therapy with Capecitabine/Oxaliplatin plus Bevacizumab: A Case Report. Case Rep Oncol 2012;5:216-21. [Crossref] [PubMed]
- Barney BM, Markovic SN, Laack NN, et al. Increased bowel toxicity in patients treated with a vascular endothelial growth factor inhibitor (VEGFI) after stereotactic body radiation therapy (SBRT). Int J Radiat Oncol Biol Phys 2013;87:73-80. [Crossref] [PubMed]
- Vuky J, Pham HT, Warren S, et al. Phase II study of long-term androgen suppression with bevacizumab and intensity-modulated radiation therapy (IMRT) in high-risk prostate cancer. Int J Radiat Oncol Biol Phys 2012;82:e609-15. [Crossref] [PubMed]
- Fury MG, Xiao H, Sherman EJ, et al. Phase II trial of bevacizumab + cetuximab + cisplatin with concurrent intensity-modulated radiation therapy for patients with stage III/IVB head and neck squamous cell carcinoma. Head Neck 2016;38:E566-70. [Crossref] [PubMed]
- Ahn PH, Machtay M, Anne PR, et al. Phase I Trial Using Induction Ciplatin, Docetaxel, 5-FU and Erlotinib Followed by Cisplatin, Bevacizumab and Erlotinib With Concurrent Radiotherapy for Advanced Head and Neck Cancer. Am J Clin Oncol 2018;41:441-6. [Crossref] [PubMed]
- Yoo DS, Kirkpatrick JP, Craciunescu O, et al. Prospective trial of synchronous bevacizumab, erlotinib, and concurrent chemoradiation in locally advanced head and neck cancer. Clin Cancer Res 2012;18:1404-14. [Crossref] [PubMed]
- Yao M, Galanopoulos N, Lavertu P, et al. Phase II study of bevacizumab in combination with docetaxel and radiation in locally advanced squamous cell carcinoma of the head and neck. Head Neck 2015;37:1665-71. [Crossref] [PubMed]
- Argiris A, Bauman JE, Ohr J, et al. Phase II randomized trial of radiation therapy, cetuximab, and pemetrexed with or without bevacizumab in patients with locally advanced head and neck cancer. Ann Oncol 2016;27:1594-600. [Crossref] [PubMed]
- Salama JK, Haraf DJ, Stenson KM, et al. A randomized phase II study of 5-fluorouracil, hydroxyurea, and twice-daily radiotherapy compared with bevacizumab plus 5-fluorouracil, hydroxyurea, and twice-daily radiotherapy for intermediate-stage and T4N0-1 head and neck cancers. Ann Oncol 2011;22:2304-9. [Crossref] [PubMed]
- Fury MG, Lee NY, Sherman E, et al. A phase 2 study of bevacizumab with cisplatin plus intensity-modulated radiation therapy for stage III/IVB head and neck squamous cell cancer. Cancer 2012;118:5008-14. [Crossref] [PubMed]
- Nyflot MJ, Kruser TJ, Traynor AM, et al. Phase 1 trial of bevacizumab with concurrent chemoradiation therapy for squamous cell carcinoma of the head and neck with exploratory functional imaging of tumor hypoxia, proliferation, and perfusion. Int J Radiat Oncol Biol Phys 2015;91:942-51. [Crossref] [PubMed]
- Lee NY, Zhang Q, Pfister DG, et al. Addition of bevacizumab to standard chemoradiation for locoregionally advanced nasopharyngeal carcinoma (RTOG 0615): a phase 2 multi-institutional trial. Lancet Oncol 2012;13:172-80. [Crossref] [PubMed]
- De Yao JT, Sun D, Powell AT, et al. Scalp Angiosarcoma Remission with Bevacizumab and Radiotherapy without Surgery: A Case Report and Review of the Literature. Sarcoma 2011;2011:160369. [Crossref] [PubMed]
- Monk SH, Biester EC, Kadakia KC, et al. Esophageal-meningeal fistula after anterior cervical corpectomy, stereotactic body radiation therapy (SBRT), and bevacizumab-containing systemic therapy for metastatic epidural spinal cord compression (MESCC). Interdisciplinary Neurosurgery 2021;26:101343. [Crossref]
- Pernin V, Belin L, Cottu P, et al. Late toxicities and outcomes of adjuvant radiotherapy combined with concurrent bevacizumab in patients with triple-negative non-metastatic breast cancer. Br J Radiol 2015;88:20140800. [Crossref] [PubMed]
- Porcelli T, Sessa F, Gambale C, et al. Management of one patient with oligoprogressive thyroid cancer during treatment with lenvatinib. Future Oncol 2019;15:21-5. [Crossref] [PubMed]
- Wang Q, Ji X, Sun J, et al. Comparison of stereotactic body radiotherapy with and without lenvatinib for advanced hepatocellular carcinoma: a propensity score analysis. J Cancer Res Clin Oncol 2023;149:7441-52. [Crossref] [PubMed]
- Yu JI, Kang W, Yoo GS, et al. Safety and Efficacy of Liver-Directed Radiotherapy in Combination With Lenvatinib for Hepatocelluar Carcinoma With Macroscopic Tumor Thrombosis. Front Oncol 2022;12:888755. [Crossref] [PubMed]
- Hiniker SM, Chen DS, Reddy S, et al. A systemic complete response of metastatic melanoma to local radiation and immunotherapy. Transl Oncol 2012;5:404-7. [Crossref] [PubMed]
- Gutkin PM, Hiniker SM, Swetter SM, et al. Complete Response of Metastatic Melanoma to Local Radiation and Immunotherapy: 6.5 Year Follow-Up. Cureus 2018;10:e3723. [Crossref] [PubMed]
- Chiang CL, Chan ACY, Chiu KWH, et al. Combined Stereotactic Body Radiotherapy and Checkpoint Inhibition in Unresectable Hepatocellular Carcinoma: A Potential Synergistic Treatment Strategy. Front Oncol 2019;9:1157. [Crossref] [PubMed]
- Hermel DJ, Du EZ, Lin R, et al. Checkpoint Inhibition in the Treatment of Unresectable, Advanced Lymphoepithelioma-like Hepatocellular Carcinoma. J Clin Transl Hepatol 2021;9:265-8. [Crossref] [PubMed]
- Xie C, Duffy AG, Brar G, et al. Immune Checkpoint Blockade in Combination with Stereotactic Body Radiotherapy in Patients with Metastatic Pancreatic Ductal Adenocarcinoma. Clin Cancer Res 2020;26:2318-26. [Crossref] [PubMed]
- Welsh JW, Tang C, de Groot P, et al. Phase II Trial of Ipilimumab with Stereotactic Radiation Therapy for Metastatic Disease: Outcomes, Toxicities, and Low-Dose Radiation-Related Abscopal Responses. Cancer Immunol Res 2019;7:1903-9. [Crossref] [PubMed]
- McBride S, Sherman E, Tsai CJ, et al. Randomized Phase II Trial of Nivolumab With Stereotactic Body Radiotherapy Versus Nivolumab Alone in Metastatic Head and Neck Squamous Cell Carcinoma. J Clin Oncol 2021;39:30-7. [Crossref] [PubMed]
- Theelen WSME, Peulen HMU, Lalezari F, et al. Effect of Pembrolizumab After Stereotactic Body Radiotherapy vs Pembrolizumab Alone on Tumor Response in Patients With Advanced Non-Small Cell Lung Cancer: Results of the PEMBRO-RT Phase 2 Randomized Clinical Trial. JAMA Oncol 2019;5:1276-82. [Crossref] [PubMed]
- Louvel G, Bahleda R, Ammari S, et al. Immunotherapy and pulmonary toxicities: can concomitant immune-checkpoint inhibitors with radiotherapy increase the risk of radiation pneumonitis? Eur Respir J 2018;51:1701737. [Crossref] [PubMed]
- Luke JJ, Lemons JM, Karrison TG, et al. Safety and Clinical Activity of Pembrolizumab and Multisite Stereotactic Body Radiotherapy in Patients With Advanced Solid Tumors. J Clin Oncol 2018;36:1611-8. [Crossref] [PubMed]
- Welsh J, Menon H, Chen D, et al. Pembrolizumab with or without radiation therapy for metastatic non-small cell lung cancer: a randomized phase I/II trial. J Immunother Cancer 2020;8:e001001. [Crossref] [PubMed]
- Maity A, Mick R, Huang AC, et al. A phase I trial of pembrolizumab with hypofractionated radiotherapy in patients with metastatic solid tumours. Br J Cancer 2018;119:1200-7. [Crossref] [PubMed]
- Monjazeb AM, Giobbie-Hurder A, Lako A, et al. A Randomized Trial of Combined PD-L1 and CTLA-4 Inhibition with Targeted Low-Dose or Hypofractionated Radiation for Patients with Metastatic Colorectal Cancer. Clin Cancer Res 2021;27:2470-80. [Crossref] [PubMed]
- Hiniker SM, Reddy SA, Maecker HT, et al. A Prospective Clinical Trial Combining Radiation Therapy With Systemic Immunotherapy in Metastatic Melanoma. Int J Radiat Oncol Biol Phys 2016;96:578-88. [Crossref] [PubMed]
- Zhong L, Wu D, Peng W, et al. Safety of PD-1/PD-L1 Inhibitors Combined With Palliative Radiotherapy and Anti-Angiogenic Therapy in Advanced Hepatocellular Carcinoma. Front Oncol 2021;11:686621. [Crossref] [PubMed]
- Tang C, Welsh JW, de Groot P, et al. Ipilimumab with Stereotactic Ablative Radiation Therapy: Phase I Results and Immunologic Correlates from Peripheral T Cells. Clin Cancer Res 2017;23:1388-96. [Crossref] [PubMed]
- Levy A, Massard C, Soria JC, et al. Concurrent irradiation with the anti-programmed cell death ligand-1 immune checkpoint blocker durvalumab: Single centre subset analysis from a phase 1/2 trial. Eur J Cancer 2016;68:156-62. [Crossref] [PubMed]
- Amagai R, Fujimura T, Kambayashi Y, et al. Three cases of nivolumab therapy-failed advanced melanoma successfully controlled by ipilimumab with intensity-modulated radiotherapy. J Dermatol 2019;46:449-52. [Crossref] [PubMed]
- Golden EB, Demaria S, Schiff PB, et al. An abscopal response to radiation and ipilimumab in a patient with metastatic non-small cell lung cancer. Cancer Immunol Res 2013;1:365-72. [Crossref] [PubMed]
- Thariat J, Hannoun-Levi JM, Sun Myint A, et al. Past, present, and future of radiotherapy for the benefit of patients. Nat Rev Clin Oncol 2013;10:52-60. [Crossref] [PubMed]
- Folkert MR, Timmerman RD. Stereotactic ablative body radiosurgery (SABR) or Stereotactic body radiation therapy (SBRT). Adv Drug Deliv Rev 2017;109:3-14. [Crossref] [PubMed]
- Qiu B, Aili A, Xue L, et al. Advances in Radiobiology of Stereotactic Ablative Radiotherapy. Front Oncol 2020;10:1165. [Crossref] [PubMed]
- Steel GG, McMillan TJ, Peacock JH. The 5Rs of radiobiology. Int J Radiat Biol 1989;56:1045-8. [Crossref] [PubMed]
- Withers HR. The Four R's of Radiotherapy. Advances in Radiation Biology 1975;5:241-71. [Crossref]
- Brown JM, Carlson DJ, Brenner DJ. The tumor radiobiology of SRS and SBRT: are more than the 5 Rs involved? Int J Radiat Oncol Biol Phys 2014;88:254-62. [Crossref] [PubMed]
- Liu L, Cao Y, Chen C, et al. Sorafenib blocks the RAF/MEK/ERK pathway, inhibits tumor angiogenesis, and induces tumor cell apoptosis in hepatocellular carcinoma model PLC/PRF/5. Cancer Res 2006;66:11851-8. [Crossref] [PubMed]
- Strumberg D, Clark JW, Awada A, et al. Safety, pharmacokinetics, and preliminary antitumor activity of sorafenib: a review of four phase I trials in patients with advanced refractory solid tumors. Oncologist 2007;12:426-37. [Crossref] [PubMed]
- Moore M, Hirte HW, Siu L, et al. Phase I study to determine the safety and pharmacokinetics of the novel Raf kinase and VEGFR inhibitor BAY 43-9006, administered for 28 days on/7 days off in patients with advanced, refractory solid tumors. Ann Oncol 2005;16:1688-94. [Crossref] [PubMed]
- Cao Y, Li CY, Moeller BJ, et al. Observation of incipient tumor angiogenesis that is independent of hypoxia and hypoxia inducible factor-1 activation. Cancer Res 2005;65:5498-505. [Crossref] [PubMed]
- Girard N, Mornex F. Sorafenib and radiotherapy association for hepatocellular carcinoma. Cancer/Radiothérapie 2011;15:77-80. [Crossref] [PubMed]
- Brandi G, De Lorenzo S, Di Girolamo S, et al. Fulminant hepatitis in a patient with hepatocellular carcinoma related to nonalcoholic steatohepatitis treated with sorafenib. Tumori 2015;101:e46-8. [Crossref] [PubMed]
- Van Hootegem A, Verslype C, Van Steenbergen W. Sorafenib-induced liver failure: a case report and review of the literature. Case Reports Hepatol 2011;2011:941395. [Crossref] [PubMed]
- Rao J, Feng M, Qian X, et al. Liver transplantation treating the patient with hepatic failure associated with sorafenib treatment: report of a case. Hepatogastroenterology 2013;60:1317-9. [PubMed]
- Gupta-Abramson V, Troxel AB, Nellore A, et al. Phase II trial of sorafenib in advanced thyroid cancer. J Clin Oncol 2008;26:4714-9. [Crossref] [PubMed]
- Koay EJ, Owen D, Das P. Radiation-Induced Liver Disease and Modern Radiotherapy. Semin Radiat Oncol 2018;28:321-31. [Crossref] [PubMed]
- Dawson LA, Normolle D, Balter JM, et al. Analysis of radiation-induced liver disease using the Lyman NTCP model. Int J Radiat Oncol Biol Phys 2002;53:810-21. [Crossref] [PubMed]
- Lee MT, Kim JJ, Dinniwell R, et al. Phase I study of individualized stereotactic body radiotherapy of liver metastases. J Clin Oncol 2009;27:1585-91. [Crossref] [PubMed]
- Andreyev J. Gastrointestinal complications of pelvic radiotherapy: are they of any importance? Gut 2005;54:1051-4. [Crossref] [PubMed]
- Velec M, Haddad CR, Craig T, et al. Predictors of Liver Toxicity Following Stereotactic Body Radiation Therapy for Hepatocellular Carcinoma. Int J Radiat Oncol Biol Phys 2017;97:939-46. [Crossref] [PubMed]
- Kazazi-Hyseni F, Beijnen JH, Schellens JH. Bevacizumab. Oncologist 2010;15:819-25. [Crossref] [PubMed]
- Schmidt B, Lee HJ, Ryeom S, et al. Combining Bevacizumab with Radiation or Chemoradiation for Solid Tumors: A Review of the Scientific Rationale, and Clinical Trials. Curr Angiogenes 2012;1:169-79. [Crossref] [PubMed]
- Gorski DH, Beckett MA, Jaskowiak NT, et al. Blockage of the vascular endothelial growth factor stress response increases the antitumor effects of ionizing radiation. Cancer Res 1999;59:3374-8. [PubMed]
- Lee CG, Heijn M, di Tomaso E, et al. Anti-Vascular endothelial growth factor treatment augments tumor radiation response under normoxic or hypoxic conditions. Cancer Res 2000;60:5565-70. [PubMed]
- Gupta VK, Jaskowiak NT, Beckett MA, et al. Vascular endothelial growth factor enhances endothelial cell survival and tumor radioresistance. Cancer J 2002;8:47-54. [Crossref] [PubMed]
- Trotti A, Bellm LA, Epstein JB, et al. Mucositis incidence, severity and associated outcomes in patients with head and neck cancer receiving radiotherapy with or without chemotherapy: a systematic literature review. Radiother Oncol 2003;66:253-62. [Crossref] [PubMed]
- Szczesniak MM, Maclean J, Zhang T, et al. Persistent dysphagia after head and neck radiotherapy: a common and under-reported complication with significant effect on non-cancer-related mortality. Clin Oncol (R Coll Radiol) 2014;26:697-703. [Crossref] [PubMed]
- Qi WX, Shen Z, Tang LN, et al. Bevacizumab increases the risk of gastrointestinal perforation in cancer patients: a meta-analysis with a focus on different subgroups. Eur J Clin Pharmacol 2014;70:893-906. [Crossref] [PubMed]
- Kavanagh BD, Pan CC, Dawson LA, et al. Radiation dose-volume effects in the stomach and small bowel. Int J Radiat Oncol Biol Phys 2010;76:S101-7. [Crossref] [PubMed]
- Garcia-Barros M, Paris F, Cordon-Cardo C, et al. Tumor response to radiotherapy regulated by endothelial cell apoptosis. Science 2003;300:1155-9. [Crossref] [PubMed]
- Fuks Z, Kolesnick R. Engaging the vascular component of the tumor response. Cancer Cell 2005;8:89-91. [Crossref] [PubMed]
- Une N, Takano-Kasuya M, Kitamura N, et al. The anti-angiogenic agent lenvatinib induces tumor vessel normalization and enhances radiosensitivity in hepatocellular tumors. Med Oncol 2021;38:60. [Crossref] [PubMed]
- Matsuki M, Hoshi T, Yamamoto Y, et al. Lenvatinib inhibits angiogenesis and tumor fibroblast growth factor signaling pathways in human hepatocellular carcinoma models. Cancer Med 2018;7:2641-53. [Crossref] [PubMed]
- Maritaz C, Broutin S, Chaput N, et al. Immune checkpoint-targeted antibodies: a room for dose and schedule optimization? J Hematol Oncol 2022;15:6. [Crossref] [PubMed]
- Grassberger C, Ellsworth SG, Wilks MQ, et al. Assessing the interactions between radiotherapy and antitumour immunity. Nat Rev Clin Oncol 2019;16:729-45. [Crossref] [PubMed]
- Suresh K, Voong KR, Shankar B, et al. Pneumonitis in Non-Small Cell Lung Cancer Patients Receiving Immune Checkpoint Immunotherapy: Incidence and Risk Factors. J Thorac Oncol 2018;13:1930-9. [Crossref] [PubMed]
- Bledsoe TJ, Nath SK, Decker RH. Radiation Pneumonitis. Clin Chest Med 2017;38:201-8. [Crossref] [PubMed]
- Kountouras J, Zavos C. Recent advances in the management of radiation colitis. World J Gastroenterol 2008;14:7289-301. [Crossref] [PubMed]
- Prieux-Klotz C, Dior M, Damotte D, et al. Immune Checkpoint Inhibitor-Induced Colitis: Diagnosis and Management. Target Oncol 2017;12:301-8. [Crossref] [PubMed]
- Antonia SJ, Villegas A, Daniel D, et al. Durvalumab after Chemoradiotherapy in Stage III Non-Small-Cell Lung Cancer. N Engl J Med 2017;377:1919-29. [Crossref] [PubMed]
- Corsello SM, Barnabei A, Marchetti P, et al. Endocrine side effects induced by immune checkpoint inhibitors. J Clin Endocrinol Metab 2013;98:1361-75. [Crossref] [PubMed]
- Faje A, Reynolds K, Zubiri L, et al. Hypophysitis secondary to nivolumab and pembrolizumab is a clinical entity distinct from ipilimumab-associated hypophysitis. Eur J Endocrinol 2019;181:211-9. [Crossref] [PubMed]
- Couey MA, Bell RB, Patel AA, et al. Delayed immune-related events (DIRE) after discontinuation of immunotherapy: diagnostic hazard of autoimmunity at a distance. J Immunother Cancer 2019;7:165. [Crossref] [PubMed]
- Kanjanapan Y, Day D, Butler MO, et al. Delayed immune-related adverse events in assessment for dose-limiting toxicity in early phase immunotherapy trials. Eur J Cancer 2019;107:1-7. [Crossref] [PubMed]