Immunotherapy for patients with thyroid cancer: a comprehensive appraisal
Introduction
Thyroid cancer is the most common endocrine neoplasm (1). In recent decades, its incidence has increased due to advances in diagnostic methods such as ultrasonography (2). Among the histological subtypes, papillary thyroid carcinoma (PTC) represents 90% of cases and is characterized by a favorable response to surgical treatment (thyroidectomy), followed by radioiodine therapy in high-risk cases, resulting in a 10-year survival rate of 90% (3). However, some PTC cases (5–10%) exhibit recurrence or metastatic disease and do not respond to standard treatment. In addition, there are other subtypes of thyroid cancers, such as undifferentiated carcinomas, including anaplastic carcinoma, and medullary thyroid carcinoma (MTC), which show poor response to surgical treatment and radioiodine therapy, resulting in lower survival rates and a worse prognosis (4). This fact reinforces the urgent need for a deep understanding of the tumor biology of thyroid neoplasms, especially the molecular biology of the tumor microenvironment.
The close relationship between tumor microenvironment and cancer cells is well established. The modulation of the immune system within the tumor microenvironment is of enormous importance to oncologists and immunologists in the fight against cancer. In this context, the field of immunotherapy has been developed. In recent years, some examples of this strategy, such as immune checkpoint inhibitors (ICIs), have demonstrated promising clinical results in patients with metastatic melanoma, non-small cell lung carcinoma, and renal carcinoma (5). In fact, emerging literature in translational immunology has opened up new frontiers for immunotherapy against various tumors, like poorly differentiated thyroid cancer. Furthermore, the increasing knowledge about immunotherapy has brought this anticancer modality closer to many physicians. Therefore, the management of patients undergoing immunotherapy modalities could become a real clinical scenario. As a result of these findings, there has been interest in studying and researching the use of immunotherapeutics against other solid cancers, especially those with poor response to initial treatment and aggressive behavior, including undifferentiated thyroid carcinoma (6) and MTC.
The goals of this review are to examine aspects of the immune response to thyroid cancer, discuss current modalities with evidence of immunotherapy against refractory thyroid cancer, and address the therapeutic challenges posed by such thyroid cancers. Table 1 summarizes the main studies of immunotherapy in the treatment of advanced thyroid cancer.
Table 1
NCT number | Study status | Conditions | Interventions | Primary outcome | Secondary outcome | Phase | Sponsor | Allocation | Intervention model | Masking |
---|---|---|---|---|---|---|---|---|---|---|
NCT02973997 | Completed | Advanced follicular cell-derived thyroid cancer | Lenvatinib and pembrolizumab | CR | Tolerability, PFS, OS | II | Academic and Community Cancer Research United | N/A | Single group assignment | N/A |
NCT03914300 | A/NR | Advanced follicular cell-derived thyroid cancer | Cabozantinib, nivolumab, and ipilimumab | ORR | Tolerability, PFS, OS | II | National Cancer Institute | N/A | Single group assignment | N/A |
NCT04731740 | Suspended by financial problems | Metastatic anaplastic thyroid cancer and poorly differentiated thyroid cancer | Pembrolizumab + lenvatinib and pembrolizumab + chemotherapy | ORR, OS | Tolerability, DoR, PFS | II | Saint Petersburg State University, Russia | Non randomized | Parallel assignment | Open label |
NCT03211117 | Completed | Anaplastic thyroid cancer | Pembrolizumab, surgery, chemoradiation | OS | Tolerability | II | Mayo Clinic | Non randomized | Parallel assignment | Open label |
NCT03181100 | A/NR | Advanced follicular cell-derived thyroid cancer | Cohort I: vemurafenib, cobimetinib, atezolizumab. Cohort II: atezolizumab, cobimetinib. Cohort III: atezolizumab, bevacizumab. Cohort IV: nab-paclitaxel, atezolizumab, paclitaxel | OS | PFS, tolerability | II | M.D. Anderson Cancer Center | Non randomized | Parallel assignment | Open label |
NCT03246958 | A/NR | Advanced or metastatic follicular cell-derived thyroid cancer | Nivolumab and ipilimumab | ORR | PFS, OS and tolerability | II | Dana-Farber Cancer Institute | Randomized | Parallel assignment | Open label |
NCT02688608 | Completed | Anaplastic thyroid cancer | Pembrolizumab | ORR | PFS, OS and tolerability | II | Saad A Khan | N/A | Single group assignment | Open label |
NCT04400474 | A/NR | Endocrine tumors, including anaplastic thyroid cancer | Combination of cabozantinib and atezolizumab | ORR | Tolerability, PFS, OS, DoR | II | Grupo Espanol de Tumores Neuroendocrinos | N/A | Single group assignment | Open label |
NCT03072160 | Completed | Recurrent or metastatic medullary thyroid Cancer | Pembrolizumab | ORR | Peripheral blood cells, sustained decline in carcinoembryonic antigen, sustained decline in calcitonin, PFS, OS, tolerability | II | National Cancer Institute | Randomized | Parallel assignment | Open label |
NCT02404441 | Completed | Advanced malignancies, including thyroid cancer | PDR001† | Phase I: pharmacokinetics and dose limiting toxicities. Phase II: ORR by RECIST | Phase I: pharmacokinetics, DCR, PFS, DoR. Phase II: DCR, PFS, DoR | I and II | Novartis Pharmaceuticals | Non randomized | Single group assignment | Open label |
NCT03449108 | A/NR | Advanced malignancies, including thyroid cancer | Aldesleukin, LN-145‡, LN-145-S1‡, chemotherapy, ipilimumab, nivolumab | ORR | DCR, DoR, PFS, OS, tolerability | II | M.D. Anderson Cancer Center | Non randomized | Parallel assignment | Open label |
NCT03012620 | A/NR | Rare tumors, including thyroid cancer | Pembrolizumab | ORR | PFS, OS, DoR, time to response, tolerability and ORR according to expression of immune markers | II | UNICANCER | N/A | Single group assignment | Open label |
NCT03170960 | A/NR | Advanced malignancies, including thyroid cancer | Dose escalation of cabozantinib and atezolizumab | Tolerability | Tolerability | Ib | Exelixis | Non randomized | Sequential assignment | Open label |
NCT04234113 | A/NR | Advanced malignancies, including thyroid cancer | SO-C101§ and pembrolizumab | Tolerability | Pharmacokinetics | Ib | SOTIO Biotech AG | Non randomized | Sequential assignment | Open label |
NCT02465060 | A/NR | Advanced malignancies, including thyroid cancer | Relatlimab¶, nivolumab and tyrosine-kinase inhibitors | ORR | OS, PFS | II | National Cancer Institute | Non randomized | Parallel assignment | Open label |
NCT02834013 | A/NR | Rare tumors, including thyroid cancer | Nivolumab and ipilimumab | ORR | Tolerability, OS, PFS | II | National Cancer Institute | Non randomized | Parallel assignment | Open label |
†, block the interaction between PD-1 and its ligands, PD-L1 and PD-L2; ‡, Autologous Tumor Infiltrating Lymphocytes; §, IL 15/IL-15Rα sushi + domain fusion protein; ¶, block LAG-3 and PD-1. NCT, National Clinical Trial; A/NR, active not recruiting; ORR, objective response rate (PR + CR); PR, partial response; CR, complete response; OS, overall survival; PFS, progression-free survival; DoR, duration of response; DCR, disease control rate; N/A, not available.
Physiology of the immune response in thyroid cancer
Cancer development is a multi-step process involving mutations in a series of genes that play a role in maintaining a balance between cell proliferation and apoptosis, resulting in uncontrolled cell mass growth (7). Associated with the genetic basis, the immune system plays a crucial role in the control and development of cancer, from its early stages to progression and metastasis (8). The complex and dynamic interaction between the immune system and cancer cells can be understood from the perspective of cancer immunoediting, a process comprising three phases: elimination, equilibrium, and escape (9).
In the elimination phase, the immune system identifies and eliminates cancer cells, preventing the cancer from becoming clinically apparent (10). This is a continuous process that prevents the tumoral progression of a significant percentage of mutated/atypical cells and can be referred to as immune surveillance (11). However, developing mechanisms by tumor cells to bypass immune surveillance can hinder the complete elimination of a tumoral tissue (10,11). Thus, the selection of mutated cells resistant to immune surveillance creates a balance between the destruction and proliferation of tumor cells, characterizing the equilibrium phase, where there is constant remodeling of the tumor tissue by the action of the immune system (12). The progressive accumulation of mutations in cancer cells surviving the equilibrium phase may lead to complete evasion of the innate and adaptive immune response, characterizing the escape phase (12). The development of immune escape mechanisms is, therefore, essential in the progression and dissemination of the tumor (13).
The strategies acquired by cancer cells to circumvent the anti-tumor action of the immune system, as shown in Figure 1, consist of loss of antigenicity, loss of immunogenicity, and the promotion of an immunologically suppressed tumor microenvironment (13). First, the loss of antigenicity involves the reduction in the production and expression of immunogenic antigens (those capable of activating an immune response) by tumor cells, whether they are unique to tumor cells (tumor-specific antigens) or shared with host normal cells (tumor-associated antigens) (14). As a result, subpopulations of cancer cells with lower antigenicity have a survival advantage and can escape the immune system in the elimination phase (15). Loss of immunogenicity, on the other hand, involves a decrease in the immune response mediated by the recognition of antigens on tumor cells (13). Although the reduction in antigenicity of tumor cells reflects lower immunogenicity, other mechanisms are responsible for this reduction.
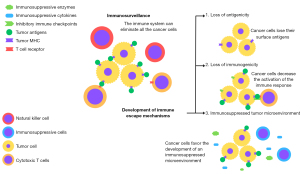
Understanding the complex interaction between cancer and the immune system, from anti-tumor immune mechanisms to the multiple strategies used by cancer cells to escape, is a crucial piece in the development of therapies that support the immune system’s action in eliminating and controlling cancer.
Immunotherapy and modalities
The fields of immunology and oncology have been interconnected since the late 19th century when surgeon William Coley reported that injecting dead bacteria into sarcoma sites could lead to tumor reduction (16). Since then, immune system modulations have piqued the interest of oncologists and immunologists because they aim to enhance the immune response, and harness immunosurveillance to target cancer cells, particularly in cases with poor response to standard treatment (17). In this context, several immunotherapies have been studied and developed in recent years to unleash the immune system and control malignancy. These approaches include antibody-based therapy, chimeric antigen receptor T-cell (CART T cell) therapy, cancer vaccines, cytokines, oncolytic viruses, and T cells (ICIs and agonism of costimulatory receptors). Recently, ICIs, such as anti-cytotoxic T-lymphocyte-associated protein 4 (CTLA-4) and anti-programmed cell death protein 1/programmed cell death protein-ligand 1 (PD-L1/PD-1), have stood out and provide significant clinical benefits to patients in advanced stages (18,19).
CTLA-4 is a transmembrane receptor that negatively modulates the immune response, functioning as a physiological “brake”. CTLA-4 expression primarily occurs in regulatory T cells and activated CD4+ and CD8+ T cells and is regulated by cytokines such as IL-12 and IFN-gamma (20). This receptor binds to CD80 or CD86 on antigen-presenting cells. Anti-CTLA-4 drugs work by inhibiting the interaction between CTLA-4 on T cells during antigen presentation (Figure 2), thereby promoting stimulation of the adaptive immune response and enhancing T cell activation (21). Ipilimumab, a monoclonal antibody that blocks CTLA-4, was the first successfully developed medication in the checkpoint inhibitor class for the treatment of metastatic melanoma (22,23).
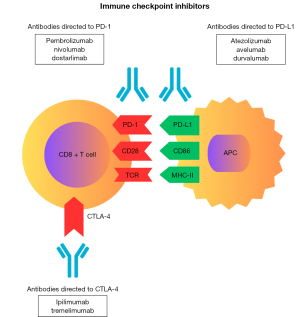
Another target for immune control is the transmembrane protein PD-1, expressed in activated T cells, B cells, and natural killer (NK) cells (24). This inhibitory molecule binds to its ligands, PD-L1 and PD-L2 (programmed death-ligand 2), which are expressed in many tumor cells and hematopoietic cells, including leukocytes (25). The immunosuppressive action of the PD-1 receptor is activated in the effector phase of the interaction between T lymphocytes and tumor cells through the binding of PD-1 to PD-L1/L2, resulting in the inhibition of tumor cell apoptosis, exhaustion of peripheral effector T cells, and conversion of effector T cells into Tregs (26). Other cells also express PD-1 and/or PD-L1, including NK cells, monocytes, and dendritic cells (DCs) (27). The class of drugs that block PD-1/PD-L1 (Figure 2), including PD-1 inhibitors (pembrolizumab, nivolumab, dostarlimab) and PD-L1 inhibitors (atezolizumab, avelumab, and durvalumab), exhibit varying efficacy for various types of cancers, including renal, small cell lung, non-small cell lung, head and neck, esophageal, gastric, hepatocellular, bladder, breast, Hodgkin lymphoma, and others (9,28), based on phase II/III studies and specific clinical indications.
The simultaneous blockade of PD-1 and CTLA-4 is also a current research strategy (29). We have clinical evidence that the combination of nivolumab (PD-1 inhibitor) and ipilimumab (CTLA-4 inhibitor) improved overall survival in cases of metastatic melanoma, renal cell carcinoma, non-small cell lung cancer, and other neoplasms (29-31). However, despite the tremendous advances in clinical benefits of the ICI, the side effects of these drugs can be an important bottleneck for the treatment for some patients.
Side effects related to immunotherapy
Published data suggest a 7–19% rate of side effects related to the use of anti-PD1/PD-L1 (32,33), 15% with ipilimumab, and up to 36% with the combined therapy of nivolumab and ipilimumab (34). Virtually all organs can be affected by immune-related side effects, with the time of onset of signs and symptoms varying, averaging around 40 days after treatment initiation. The addition of ICIs to chemotherapy does not exacerbate the side effects related to chemotherapy but adds toxicities related to the immune system (35,36).
The American Society of Oncology organized a multidisciplinary panel that reviewed the literature and proposed general guidelines and organ-specific recommendations for managing adverse events associated with ICIs. Treatment is based on the affected organ and the severity of observed toxicity. In general, the treatment of adverse events related to ICI therapy requires careful monitoring and surveillance of patients in mild cases and the discontinuation of ICIs and immunosuppression with glucocorticoids in moderate to severe cases (37).
Among the systemic effects related to ICIs, fatigue is most commonly noted, with an incidence of 16–24% in PD-1/PD-L1 inhibitors and approximately 26% in combination immunotherapy. Fatigue is generally a mild condition, and severe cases are rare (38). Infusion-related effects may also occur, especially with the use of avelumab, for which pre-medication with antihistamines and analgesics/antipyretics is recommended (39). Among other adverse effects, we can highlight:
- Cutaneous manifestations related to the use of ICIs include inflammatory dermatoses, bullous dermatoses, and severe cutaneous adverse reactions (Stevens-Johnson Syndrome, Toxic Epidermal Necrolysis, and Acute Generalized Exanthematous Pustulosis) with an average onset time of 4 weeks (40). Rash, itching (with or without rash), and vitiligo are the most prevalent, reported in 71.5% of patients on immunotherapy (41).
- Intestinal toxicity is one of the most common complications associated with the use of ICIs. The incidence of colitis varies from 8% to 27%, and diarrhea can reach up to 54% in patients using anti-CTLA-4, especially in combination therapy. The incidence of diarrhea related to anti-PD-1 monotherapy is less than 20% (42). Other gastrointestinal manifestations include hepatitis, gastritis, and enterocolitis, with an average onset time of 6 weeks. These present as nausea, fever, abdominal pain, diarrhea, mucus, and blood in the stool (43).
- Pneumonitis is a potentially severe and fatal pulmonary complication associated with the use of ICIs, although it is uncommon, with a variable incidence of approximately 2.7% with anti-PD-1/PD-L1 use. It is less common with anti-CTLA-4 use, reported in less than 1% of patients using Ipilimumab in the study (44). Pulmonary symptoms manifest as cough, shortness of breath, chest pain, desaturation, and fever, with an onset time of approximately 34 weeks (43,45).
- Hypophysitis and thyroid dysfunctions are the most common immune-mediated toxicities related to the endocrine system, respectively, being most frequent with anti-PD-1 and anti-CTLA-4. Meanwhile, cases of type 1 diabetes mellitus (T1DM) and adrenal insufficiency are rare (46). Hypophysitis is one of the few irreversible toxicities, with studies showing recovery of the pituitary-thyroid axis in up to 50% of patients, 50–60% of the pituitary-gonadal axis, and a few cases of recovery of corticotrophic cells (47-49). Thyroid dysfunctions can occur in up to 6% of patients using ICIs, such as thyrotoxicosis, hypothyroidism, painless thyroiditis, and even cases of thyroid storm (48). Most anti-CTLA-4-related cases are subclinical thyroiditis that resolves after 2 to 4 infusions (47).
- The use of ICIs has also been associated with uncommon side effects in other organs, in some cases being potentially fatal and severe, including renal injuries, exocrine pancreatic toxicities, neurological manifestations (neuropathies, Guillain-Barré syndrome, myasthenia gravis), cardiac toxicity (myocarditis and pericarditis), hematological manifestations (immune thrombocytopenic purpura, autoimmune hemolytic anemia, hemophagocytic syndrome), ocular effects (episcleritis, uveitis, and conjunctivitis), and rheumatological manifestations (myositis, arthritis, vasculitis, and Sjögren’s syndrome) (50).
Immunotherapy for patients with follicular cell-derived thyroid carcinomas
Follicular cell-derived thyroid carcinomas are derived from the follicular cells in the thyroid gland, which are the epithelial cells responsible for the secretion of the iodine-containing thyroid hormones (triiodothyronine and thyroxine) (51). Follicular cell-derived thyroid carcinomas are a set of different neoplasms, such as papillary thyroid cancer (80–85%), follicular thyroid cancer (10–15%), poorly differentiated thyroid cancer (<2%), and undifferentiated (anaplastic) thyroid cancer (<2%), and these have a favorable prognosis, except for poorly differentiated and undifferentiated thyroid cancer (51). Molecularly, follicular cell-derived thyroid carcinomas present genetic alterations that constitutively activate the MAPK, PI3K, and β-catenin signaling pathways, which have been implicated in thyroid carcinoma development and progression (52). Most malignant thyroid neoplasms are initially treated with total or near-total thyroidectomy or a combination of surgery and radioactive iodine therapy (53). However, in patients with locally invasive, metastatic disease or relapse, many neoplasms become refractory to radioactive iodine therapy, and these patients will eventually require alternative treatments, such as locoregional therapies and/or systemic treatment (53). In this scenario, immunotherapy emerges as a potentially successful therapy.
The initial investigation of immunotherapy for patients with thyroid cancer was in 1992 by Misaki and collaborators (54). They investigated fourteen patients with newly diagnosed differentiated thyroid cancer and randomly assigned them to be either injected with OK-432 (streptococcal preparation) or the control group (54). The group demonstrated that the local injection of OK-432 in thyroid neoplasm was shown to recruit T lymphocytes to induce the production of tumor necrosis factor (TNF), a known potent tumoricidal molecule (54). Although the authors failed to demonstrate a clinical benefit of OK-432 injection, they brought to light the idea that follicular cell-derived thyroid carcinomas would be immunogenic and for which immunotherapy could be promising. Subsequently, clinical trials failed to demonstrate a clinical benefit of injection of interleukin 2 and interferon alpha, while these drugs were associated with considerable toxicities (54,55).
The ICIs for patients with follicular cell-derived thyroid carcinomas have been recently investigated. Eight patients with advanced thyroid carcinomas (six with anaplastic and two with poorly differentiated thyroid carcinoma) who were treated with combination therapy of lenvatinib and pembrolizumab were retrospectively revisited by Dierks et al. (56). Four (50%) patients presented severe adverse events (grade 3 or 4) (56). The best overall response among patients with anaplastic thyroid carcinoma was 66% complete remissions, 16% stable disease, and 16% progressive disease (56). All patients with poorly differentiated thyroid carcinoma presented partial remission, suggesting that the combination of therapies would be more suitable for those patients (56).
In a phase Ib clinical trial, Mehnert et al. assessed the safety of pembrolizumab 10 mg/kg every 2 weeks (57). Twenty-two patients with advanced papillary or follicular thyroid cancer present failure of standard therapy, and PD-L1 expression in tumor or stroma cells by immunohistochemistry (57). Most of the patients presented mild adverse events and only one patient presented grade 3 colitis (57). Two patients presented partial response and the response duration was 8 and 20 months (57). One phase 2 clinical trial was performed to assess the efficacy and toxicities of pembrolizumab 200 mg intravenously every 3 weeks, combined with chemoradiotherapy for patients with anaplastic thyroid carcinoma (58). Three patients were enrolled. They presented a favorable profile of toxicities (58). However, all three patients died before 6 months of the investigation, suggesting that pembrolizumab was not efficient in stopping the progression of the tumor (58).
Oh et al. recently published one ongoing single-arm multicenter, multicohort, open-label study of pembrolizumab monotherapy 200 mg once every 3 weeks for 35 cycles (59). The authors included 103 patients with papillary or follicular thyroid carcinoma who presented progression on or intolerance to at least one treatment and/or unresectable disease (59). Interestingly, most of the patients (87/103; 84.5%) discontinued the treatment (59). The reason for the discontinuation was disease progression (67/87; 65%), adverse events (12/87; 11.7%), patient withdrawal (6/87; 5.8%), physician decision (1/87; 1%) and loss of follow-up (1/87; 1%) (59). Complete response was achieved in 2/104 patients (1.9%), partial response was achieved in 5/103 patients (4.9%), and stable disease was achieved in 62/104 patients (60.2%) (59).
The NCT02688608 trial register published at clinicaltrial.gov (60) was a phase II, multicenter, open-label trial of pembrolizumab in metastatic or locally advanced anaplastic/undifferentiated thyroid carcinoma (60). Pembrolizumab was administered at 200 mg intravenous (IV) once every 3 weeks (60). Six patients were enrolled and five patients completed the study (60). Authors defined overall response as the disappearance of all target lesions (complete response) or ≥30% decrease in the sum of the longest diameter of target lesions (partial response) (60). Overall response was achieved in 3/5 patients (60%) (60). Stable disease was achieved in 1/5 patients (20%) (60).
Although preclinical studies have suggested the potential benefit of immunotherapy modalities for patients with follicular cell-derived thyroid carcinoma, to date, clinical studies have failed to demonstrate clear clinical benefits in patients with advanced thyroid cancer. More studies, especially those that focus on the combination of immunotherapeutics and other drugs, are necessary to elucidate this issue.
Immunotherapy for patients with MTCs
Medullary thyroid cancers are neuroendocrine tumors of thyroid parafollicular cells, which are responsible for the secretion of calcitonin hormones (61). It accounts for 2–4% of thyroid neoplasms (62). They can occur as sporadic tumors in 70% of the cases (63) or as an inherited manifestation of multiple endocrine neoplasia type 2 (MEN2) in 25–30% of the cases (63). Even though the treatment is primarily with surgical resection (64), and the role of radiotherapy is limited (65), for patients with metastatic disease, there is a need for systemic therapy, where immunotherapy may have an important role, and has been studied as an emerging therapeutic option (66).
Besides MTC usually have low PD-L1 expression levels (67), its positivity is related to a more aggressive behavior (68) and is sometimes associated with RET mutation (69). Therefore, some clinical trials using immunotherapy have been studied to assess the effects of blocking the PD-1/PD-L1 pathway (70). Yamamoto et al. (71) performed a phase I clinical trial using nivolumab in patients with malignant solid organ tumors, including a single MTC patient, who received 10 mg/kg infused intravenously over 1 h, through a 0.2-µm in-line filter in a total volume of 60 mL, every 2 weeks, for 12 months, and achieved partial response (71).
Few phase-II clinical trials have been registered in ClinicalTrials.gov with PD-L1. The NCT03072160 (72) (Phase II Trial of Pembrolizumab in Recurrent or Metastatic Medullary Thyroid Cancer) is a phase II, open-label, non-randomized, single-center clinical trial in patients who have recurrent or metastatic MTC, for whom surgery is not a curative option (72). Patients enrolled were separated into two arms according to previous use, or absence of use, of immune-stimulating cancer vaccine. Pembrolizumab 200 mg was administered as a 30-minute IV infusion every 3 weeks for 2 years (72). Results published in ClinicalTrials.gov show that 13 patients were enrolled in the group that received cancer vaccine previously, and four patients in the group that did not use this therapy (72). Only two patients from the vaccine group completed the study, and none of the patients enrolled achieved clinical response (defined as a 50% decrease in calcitonin levels) or imaging response (≥30% decrease in the sum of the largest diameter) (72).
Other ongoing phase-II trials, or with no results available yet, for PD-1/PD-L1 blockade immunotherapy are found on ClinicalTrials.gov. NCT03012620 (73) (Secured Access to Pembrolizumab for Patients With Selected Rare Cancer Types) investigates the efficacy and safety of pembrolizumab in patients with advanced rare cancers for which no other treatment options are available for seven groups of tumors, including MTC (73). NCT03753919 (74) [A Phase II Study of C (MEDI4736) Plus Tremelimumab for the Treatment of Patients With Progressive, Refractory Advanced Thyroid Carcinoma - The DUTHY Trial] evaluated durvalumab 1,500 mg plus tremelimumab 75 mg every 4 weeks up to 4 cycles followed by durvalumab 1,500 mg every 4 weeks until disease progression, unacceptable toxicity or patients’ decision, in patients with advanced thyroid cancer, including MTC (74).
Another pathway expressed in MTC patients that could be used as a therapeutic approach is CTLA-4, and some studies using ipilimumab have been conducted, associating with PD-1/PD-L1 pathway blockade, since they are different pathways and might have synergic effects (75). A phase-II study of patients with radioactive iodine-refractory, aggressive thyroid cancer conducted an exploratory cohort of MTC patients included seven patients with MTC enrolled between October 2017 and May 2019, treated with ipilimumab plus nivolumab. Still, none of the MTC patients achieved partial response criteria using RECIST v1.1 after 2 years (76). Only preliminary results, published as a meeting abstract, are available (76).
Other immunotherapy approaches include DC vaccination and radioimmunotherapy using an anti-carcinoembryonic antigen (CEA) monoclonal antibody and 131I-labeled hapten carrying the therapeutic payload. However, most of these studies are from several years ago, and no recent advances were found in the literature.
DC vaccination is performed through the modification of DC epitopes in the bone marrow, which among their functions, present antigens, so that it is possible to induce the patient’s immune response against the tumor by including characteristic tumor antigens in the vaccine (77). For thyroid tumors, several strategies have been tested, involving the injection of thyroid cells with lysates of tumor extracts (78,79), or tumor-specific calcitonin (80), or the association of calcitonin and CEA (81). Safety data showed this therapy to be well tolerated, with the most commonly reported adverse effect being a low-grade fever (81).
Schott et al. (81) conducted a study with DCs loaded with calcitonin and CEA peptide repeatedly delivered by subcutaneous injections, and the results showed that three of seven patients recruited had clinical responses with a decrease of serum calcitonin and CEA, one of them with complete regression of liver metastases and reduction of pulmonary lesions. However, this study was a phase-1 trial, and the follow-up period was too short to evaluate any significant clinical effect on survival (mean 13.1 months) (81).
Stift et al. (78) conducted a study with tumor cells extracted from patients with MTC, injecting autologous tumor lysate-pulsed DCs from 10 patients suffering from advanced MTC into a groin lymph node (78). The results show indications of the therapy’s effect starting from the fourth application, carried out every 21 days (78). In patients where in-vitro analysis was performed, they detected markers of adequate immune response (78). However, concerning the clinical response, of ten patients, three had a partial response, one presented a minor response, two showed stable disease, and the remaining four patients had progressive disease (78).
Papewalis et al. (80) conducted a trial in five MTC patients with tumor-specific calcitonin DCs administered twice. However, the results were only in-vitro analysis, that showed an increase in anti-tumour immune response. In this study, no clinical response was evaluated.
Bachleitner-Hofmann et al. (79) conducted a trial with ten patients with metastatic MTC treated with a novel approach, using DC vaccination from different tumor (79) lysate(s) from allogeneic tumor cell lines (79). The treatments were well-tolerated and safe. Median follow-up was 11 months (range, 7–26 months), and 3 patients showed stable disease (79).
Also, a case report from Del Rivero et al. (82) reported the case of a 61-year-old male patient diagnosed with MTC, who experienced continuous local recurrence despite undergoing multiple surgical procedures and discontinued treatment with sunitinib due to adverse effects (82). The patient was subsequently admitted to a clinical trial involving a heat-killed yeast-CEA vaccine (NCT01856920), which resulted in an improvement in his calcitonin doubling time over 3 months (82). Following a temporary discontinuation of the vaccine for elective surgery, the patient’s calcitonin levels began to rise. He was then enrolled in a phase I trial of avelumab, a PD-L1 inhibitor (NCT01772004), and had his calcitonin levels decreased by more than 40% in five consecutive evaluations (82).
Another strategy that has been studied is the use of Radioimmunotherapy, using monoclonal antibodies that bind to specific cells and carry radiotherapeutic products. In MTC, a phase-2 study (83) using 131I-labeled bivalent hapten with anti-CEA and anti-calcitonin expression (targeting parafollicular cells, such as c-cells) evaluated 29 patients with advanced-stage MTC who used this therapy and compared them with another 39 patients with the same prognostic indicators (calcitonin doubling time less than 2 years) and showed that the use of this therapy increased the overall survival time from 60 to 110 months (83). However, safety data showed high rates of bone marrow toxicity, manifesting as neutropenia, thrombocytopenia, and one case of myelodysplasia (83).
Another study with radioimmunotherapy was conducted by Kraeber-Bodéré et al. (84), a phase-I study regarding the use of anti-CEA hMN-14 × m734 bispecific antibody (BsmAb) and 131I-di-diethylenetriamine pentaacetic acid (DTPA)-indium hapten was evaluated in a phase I study performed on patients with CEA-expressing tumors, and enrolled nine patients with proven CEA-expressing MTC (84). Results showed that six of nine MTC patients had tumor stabilization of at least 3 months to more than 12 months (84). Hepatic toxicity and severe hematologic toxicity were frequent and dose-dependent (84).
Based on the current body of evidence, the potential of immunotherapy in the treatment of medullary thyroid cancer remains largely unexplored, with only phase-I and phase-II clinical trials conducted to date. These preliminary studies have yet to provide substantial data to substantiate the efficacy of immunotherapy, particularly when administered as monotherapy. Nonetheless, for patients with recurrent or refractory disease, in instances where regular treatment is not viable or the previous therapeutic approach was not effective and other clinical interventions are not an option, immunotherapeutic approaches may serve as a viable option in the advanced stages of the disease. The combination of ICIs with targeted therapies may yield enhanced therapeutic outcomes, although this hypothesis necessitates further exploration through high-quality phase-3 randomized controlled trials.
Conclusions
Thyroid cancer, despite being associated with therapeutic success in the majority of patients, can, in a small subset of patients, progress with therapeutic refractoriness and a reserved prognosis. Investigations into new treatment modalities beyond surgery, radioactive iodine ablation therapy, and thyroid-stimulating hormone (TSH) suppression therapy are increasingly necessary. In this context, understanding the interaction between immune cells and tumor cells in the thyroid cancer tumor microenvironment may provide relevant ideas for modulating this immune response in favor of malignant cell destruction. This is the fundamental concept of immunotherapy, which is advancing rapidly in various solid and hematologic tumors. Particularly, ICIs have emerged as a potentially revolutionary subtype of immunotherapy. Although associated with serious adverse events, ICI therapy has changed the prognosis for many cancers, and several studies are attempting to apply this same success to thyroid cancer. Several preclinical studies suggest that immunotherapy could be a potent therapeutic tool in patients with advanced or treatment-refractory thyroid cancer. It is worth noting that these studies in thyroid cancer patients are still in their early stages, with the vast majority in phase II, and require greater energy and investment to reach conclusive findings.
Acknowledgments
Funding: The work has received funding support from
Footnote
Peer Review File: Available at https://cco.amegroups.com/article/view/10.21037/cco-23-133/prf
Conflicts of Interest: All authors have completed the ICMJE uniform disclosure form (available at https://cco.amegroups.com/article/view/10.21037/cco-23-133/coif). The authors have no conflicts of interest to declare.
Ethical Statement: The authors are accountable for all aspects of the work in ensuring that questions related to the accuracy or integrity of any part of the work are appropriately investigated and resolved.
Open Access Statement: This is an Open Access article distributed in accordance with the Creative Commons Attribution-NonCommercial-NoDerivs 4.0 International License (CC BY-NC-ND 4.0), which permits the non-commercial replication and distribution of the article with the strict proviso that no changes or edits are made and the original work is properly cited (including links to both the formal publication through the relevant DOI and the license). See: https://creativecommons.org/licenses/by-nc-nd/4.0/.
References
- Siegel RL, Miller KD, Fuchs HE, et al. Cancer Statistics, 2021. CA Cancer J Clin 2021;71:7-33. [Crossref] [PubMed]
- McQueen AS, Bhatia KS. Thyroid nodule ultrasound: technical advances and future horizons. Insights Imaging 2015;6:173-88. [Crossref] [PubMed]
- Haugen BR, Alexander EK, Bible KC, et al. 2015 American Thyroid Association Management Guidelines for Adult Patients with Thyroid Nodules and Differentiated Thyroid Cancer: The American Thyroid Association Guidelines Task Force on Thyroid Nodules and Differentiated Thyroid Cancer. Thyroid 2016;26:1-133. [Crossref] [PubMed]
- Sipos JA, Mazzaferri EL. Thyroid cancer epidemiology and prognostic variables. Clin Oncol (R Coll Radiol) 2010;22:395-404. [Crossref] [PubMed]
- Postow MA, Callahan MK, Wolchok JD. Immune Checkpoint Blockade in Cancer Therapy. J Clin Oncol 2015;33:1974-82. [Crossref] [PubMed]
- French JD, Bible K, Spitzweg C, et al. Leveraging the immune system to treat advanced thyroid cancers. Lancet Diabetes Endocrinol 2017;5:469-81. [Crossref] [PubMed]
- N Kontomanolis E. Basic principles of molecular biology of cancer cell-Molecular cancer indicators. J BUON 2021;26:1723-34. [PubMed]
- Chen DS, Mellman I. Oncology meets immunology: the cancer-immunity cycle. Immunity 2013;39:1-10. [Crossref] [PubMed]
- Schreiber RD, Old LJ, Smyth MJ. Cancer immunoediting: integrating immunity’s roles in cancer suppression and promotion. Science 2011;331:1565-70. [Crossref] [PubMed]
- Bates JP, Derakhshandeh R, Jones L, et al. Mechanisms of immune evasion in breast cancer. BMC Cancer 2018;18:556. [Crossref] [PubMed]
- Swann JB, Smyth MJ. Immune surveillance of tumors. J Clin Invest 2007;117:1137-46. [Crossref] [PubMed]
- Dunn GP, Bruce AT, Ikeda H, et al. Cancer immunoediting: from immunosurveillance to tumor escape. Nat Immunol 2002;3:991-8. [Crossref] [PubMed]
- Beatty GL, Gladney WL. Immune escape mechanisms as a guide for cancer immunotherapy. Clin Cancer Res 2015;21:687-92. [Crossref] [PubMed]
- Jiang T, Shi T, Zhang H, et al. Tumor neoantigens: from basic research to clinical applications. J Hematol Oncol 2019;12:93. [Crossref] [PubMed]
- Dhatchinamoorthy K, Colbert JD, Rock KL. Cancer Immune Evasion Through Loss of MHC Class I Antigen Presentation. Front Immunol 2021;12:636568. [Crossref] [PubMed]
- Coley WB. The treatment of malignant tumors by repeated inoculations of erysipelas. With a report of ten original cases. 1893. Clin Orthop Relat Res 1991;3-11. [PubMed]
- Mellman I, Coukos G, Dranoff G. Cancer immunotherapy comes of age. Nature 2011;480:480-9. [Crossref] [PubMed]
- Cunha LL, Ward LS. Translating the immune microenvironment of thyroid cancer into clinical practice. Endocr Relat Cancer 2022;29:R67-83. [Crossref] [PubMed]
- Topalian SL, Hodi FS, Brahmer JR, et al. Safety, activity, and immune correlates of anti-PD-1 antibody in cancer. N Engl J Med 2012;366:2443-54. [Crossref] [PubMed]
- Van Coillie S, Wiernicki B, Xu J. Molecular and Cellular Functions of CTLA-4. Adv Exp Med Biol 2020;1248:7-32. [Crossref] [PubMed]
- Rowshanravan B, Halliday N, Sansom DM. CTLA-4: a moving target in immunotherapy. Blood 2018;131:58-67. [Crossref] [PubMed]
- Grosso JF, Jure-Kunkel MN. CTLA-4 blockade in tumor models: an overview of preclinical and translational research. Cancer Immun 2013;13:5. [PubMed]
- Schadendorf D, Hodi FS, Robert C, et al. Pooled Analysis of Long-Term Survival Data From Phase II and Phase III Trials of Ipilimumab in Unresectable or Metastatic Melanoma. J Clin Oncol 2015;33:1889-94. [Crossref] [PubMed]
- Pu Y, Ji Q. Tumor-Associated Macrophages Regulate PD-1/PD-L1 Immunosuppression. Front Immunol 2022;13:874589. [Crossref] [PubMed]
- Pauken KE, Torchia JA, Chaudhri A, et al. Emerging concepts in PD-1 checkpoint biology. Semin Immunol 2021;52:101480. [Crossref] [PubMed]
- Francisco LM, Salinas VH, Brown KE, et al. PD-L1 regulates the development, maintenance, and function of induced regulatory T cells. J Exp Med 2009;206:3015-29. [Crossref] [PubMed]
- Li W, Wu F, Zhao S, et al. Correlation between PD-1/PD-L1 expression and polarization in tumor-associated macrophages: A key player in tumor immunotherapy. Cytokine Growth Factor Rev 2022;67:49-57. [Crossref] [PubMed]
- Ascierto PA, Addeo R, Cartenì G, et al. The role of immunotherapy in solid tumors: report from the Campania Society of Oncology Immunotherapy (SCITO) meeting, Naples 2014. J Transl Med 2014;12:291. [Crossref] [PubMed]
- Wolchok JD, Chiarion-Sileni V, Gonzalez R, et al. Overall Survival with Combined Nivolumab and Ipilimumab in Advanced Melanoma. N Engl J Med 2017;377:1345-56. [Crossref] [PubMed]
- Motzer RJ, McDermott DF, Escudier B, et al. Conditional survival and long-term efficacy with nivolumab plus ipilimumab versus sunitinib in patients with advanced renal cell carcinoma. Cancer 2022;128:2085-97. [Crossref] [PubMed]
- Hellmann MD, Paz-Ares L, Bernabe Caro R, et al. Nivolumab plus Ipilimumab in Advanced Non-Small-Cell Lung Cancer. N Engl J Med 2019;381:2020-31. [Crossref] [PubMed]
- Roberts K, Culleton V, Lwin Z, et al. Immune checkpoint inhibitors: Navigating a new paradigm of treatment toxicities. Asia Pac J Clin Oncol 2017;13:277-88. [Crossref] [PubMed]
- Motzer RJ, Escudier B, McDermott DF, et al. Nivolumab versus Everolimus in Advanced Renal-Cell Carcinoma. N Engl J Med 2015;373:1803-13. [Crossref] [PubMed]
- Larkin J, Chiarion-Sileni V, Gonzalez R, et al. Combined Nivolumab and Ipilimumab or Monotherapy in Untreated Melanoma. N Engl J Med 2015;373:23-34. [Crossref] [PubMed]
- Weber JS, Kähler KC, Hauschild A. Management of immune-related adverse events and kinetics of response with ipilimumab. J Clin Oncol 2012;30:2691-7. [Crossref] [PubMed]
- Langer CJ, Gadgeel SM, Borghaei H, et al. Carboplatin and pemetrexed with or without pembrolizumab for advanced, non-squamous non-small-cell lung cancer: a andomized, phase 2 cohort of the open-label KEYNOTE-021 study. Lancet Oncol 2016;17:1497-508. [Crossref] [PubMed]
- Schneider BJ, Naidoo J, Santomasso BD, et al. Management of Immune-Related Adverse Events in Patients Treated With Immune Checkpoint Inhibitor Therapy: ASCO Guideline Update. J Clin Oncol 2021;39:4073-126. [Crossref] [PubMed]
- Zhou X, Yao Z, Bai H, et al. Treatment-related adverse events of PD-1 and PD-L1 inhibitor-based combination therapies in clinical trials: a systematic review and meta-analysis. Lancet Oncol 2021;22:1265-74. [Crossref] [PubMed]
- BAVENCIO® (avelumab) injection, for intravenous use. Initial U.S. Approval: 2017. Highlights of prescribing information. Accessed on: 12 October 2023. Available online: http://www.accessdata.fda.gov/drugsatfda_docs/label/2017/761049s000lbl.pdf
- Malviya N, Tattersall IW, Leventhal J, et al. Cutaneous immune-related adverse events to checkpoint inhibitors. Clin Dermatol 2020;38:660-78. [Crossref] [PubMed]
- Sibaud V. Dermatologic Reactions to Immune Checkpoint Inhibitors : Skin Toxicities and Immunotherapy. Am J Clin Dermatol 2018;19:345-61. [Crossref] [PubMed]
- Gupta A, De Felice KM, Loftus EV Jr, et al. Systematic review: colitis associated with anti-CTLA-4 therapy. Aliment Pharmacol Ther 2015;42:406-17. [Crossref] [PubMed]
- Qin Q, Patel VG, Wang B, et al. Type, timing, and patient characteristics associated with immune-related adverse event development in patients with advanced solid tumors treated with immune checkpoint inhibitors. Journal of Clinical Oncology 2020;38:e15160. [Crossref]
- Chuzi S, Tavora F, Cruz M, et al. Clinical features, diagnostic challenges, and management strategies in checkpoint inhibitor-related pneumonitis. Cancer Manag Res 2017;9:207-13. [Crossref] [PubMed]
- Naidoo J, Wang X, Woo KM, et al. Pneumonitis in Patients Treated With Anti-Programmed Death-1/Programmed Death Ligand 1 Therapy. J Clin Oncol 2017;35:709-17. [Crossref] [PubMed]
- Elia G, Ferrari SM, Galdiero MR, et al. New insight in endocrine-related adverse events associated to immune checkpoint blockade. Best Pract Res Clin Endocrinol Metab 2020;34:101370. [Crossref] [PubMed]
- Corsello SM, Barnabei A, Marchetti P, et al. Endocrine side effects induced by immune checkpoint inhibitors. J Clin Endocrinol Metab 2013;98:1361-75. [Crossref] [PubMed]
- Joshi MN, Whitelaw BC, Palomar MT, et al. Immune checkpoint inhibitor-related hypophysitis and endocrine dysfunction: clinical review. Clin Endocrinol (Oxf) 2016;85:331-9. [Crossref] [PubMed]
- Byun DJ, Wolchok JD, Rosenberg LM, et al. Cancer immunotherapy – immune checkpoint blockade and associated endocrinopathies. Nat Rev Endocrinol 2017;13:195-207. [Crossref] [PubMed]
- Postow M, Johnson DB. Toxicities associated with immune checkpoint inhibitors. UpToDate. Waltham, MA, USA (Acessed on October 19, 2023). Available online: https://www.uptodate.com/contents/toxicities-associated-with-immune-checkpoint-inhibitors?search=Toxicities%20associated%20with%20immune%20779%20checkpoint%20inhibitors.&source=search_result&selectedTitle=1%7E150&usage_type=default&display_rank=1
- Dralle H, Machens A, Basa J, et al. Follicular cell-derived thyroid cancer. Nat Rev Dis Primers 2015;1:15077. [Crossref] [PubMed]
- Romitti M, Ceolin L, Siqueira DR, et al. Signaling pathways in follicular cell-derived thyroid carcinomas Int J Oncol 2013;42:19-28. (review). [Crossref] [PubMed]
- Capdevila J, Awada A, Führer-Sakel D, et al. Molecular diagnosis and targeted treatment of advanced follicular cell-derived thyroid cancer in the precision medicine era. Cancer Treat Rev 2022;106:102380. [Crossref] [PubMed]
- Misaki T, Watanabe Y, Iida Y, et al. Recruitment of T lymphocytes and induction of tumor necrosis factor in thyroid cancer by a local immunotherapy. Cancer Immunol Immunother 1992;35:92-6. [Crossref] [PubMed]
- Argiris A, Agarwala SS, Karamouzis MV, et al. A phase II trial of doxorubicin and interferon alpha 2b in advanced, non-medullary thyroid cancer. Invest New Drugs 2008;26:183-8. [Crossref] [PubMed]
- Dierks C, Seufert J, Aumann K, et al. Combination of Lenvatinib and Pembrolizumab Is an Effective Treatment Option for Anaplastic and Poorly Differentiated Thyroid Carcinoma. Thyroid 2021;31:1076-85. [Crossref] [PubMed]
- Mehnert JM, Varga A, Brose MS, et al. Safety and antitumor activity of the anti-PD-1 antibody pembrolizumab in patients with advanced, PD-L1-positive papillary or follicular thyroid cancer. BMC Cancer 2019;19:196. [Crossref] [PubMed]
- Chintakuntlawar AV, Yin J, Foote RL, et al. A Phase 2 Study of Pembrolizumab Combined with Chemoradiotherapy as Initial Treatment for Anaplastic Thyroid Cancer. Thyroid 2019;29:1615-22. [Crossref] [PubMed]
- Oh DY, Algazi A, Capdevila J, et al. Efficacy and safety of pembrolizumab monotherapy in patients with advanced thyroid cancer in the phase 2 KEYNOTE-158 study. Cancer 2023;129:1195-204. [Crossref] [PubMed]
- Identifier NCT02688608, Pembrolizumab in Anaplastic/Undifferentiated Thyroid Cancer; 2022 Feb 10 [cited 2023 Oct 30]. Available online: https://clinicaltrials.gov/study/NCT02688608
- Wells SA Jr, Asa SL, Dralle H, et al. Revised American Thyroid Association guidelines for the management of medullary thyroid carcinoma. Thyroid 2015;25:567-610. [Crossref] [PubMed]
- Ceolin L, Duval MADS, Benini AF, et al. Medullary thyroid carcinoma beyond surgery: advances, challenges, and perspectives. Endocr Relat Cancer 2019;26:R499-518. [Crossref] [PubMed]
- Pacini F, Castagna MG, Cipri C, et al. Medullary thyroid carcinoma. Clin Oncol (R Coll Radiol) 2010;22:475-85. [Crossref] [PubMed]
- Kim M, Kim BH. Current Guidelines for Management of Medullary Thyroid Carcinoma. Endocrinol Metab (Seoul) 2021;36:514-24. [Crossref] [PubMed]
- Huang S, Zhong J, Zhang Z, et al. Prognosis of radiotherapy in medullary thyroid carcinoma patients without distant metastasis. Transl Cancer Res 2021;10:4714-26. [Crossref] [PubMed]
- Okafor C, Hogan J, Raygada M, et al. Update on Targeted Therapy in Medullary Thyroid Cancer. Front Endocrinol (Lausanne) 2021;12:708949. [Crossref] [PubMed]
- Bongiovanni M, Rebecchini C, Saglietti C, et al. Very low expression of PD-L1 in medullary thyroid carcinoma. Endocr Relat Cancer 2017;24:L35-8. [Crossref] [PubMed]
- Shi X, Yu PC, Lei BW, et al. Association Between Programmed Death-Ligand 1 Expression and Clinicopathological Characteristics, Structural Recurrence, and Biochemical Recurrence/Persistent Disease in Medullary Thyroid Carcinoma. Thyroid 2019;29:1269-78. [Crossref] [PubMed]
- Bi Y, Ren X, Bai X, et al. PD-1/PD-L1 expressions in medullary thyroid carcinoma: Clinicopathologic and prognostic analysis of Chinese population. Eur J Surg Oncol 2019;45:353-8. [Crossref] [PubMed]
- Martins RS, Jesus TT, Cardoso L, et al. Personalized Medicine in Medullary Thyroid Carcinoma: A Broad Review of Emerging Treatments. J Pers Med 2023;13:1132. [Crossref] [PubMed]
- Yamamoto N, Nokihara H, Yamada Y, et al. Phase I study of Nivolumab, an anti-PD-1 antibody, in patients with malignant solid tumors. Invest New Drugs 2017;35:207-16. [Crossref] [PubMed]
- Identifier NCT03072160, Pembrolizumab in Recurrent or Metastatic Medullary Thyroid Cancer; 2021 Feb 11 [cited 2023 Oct 30]. Available online: https://clinicaltrials.gov/study/NCT03072160
- Identifier NCT03012620, Secured Access to Pembrolizumab for Patients with Selected Rare Cancer Types; 2023 Feb 8 [cited 2023 Oct 30]. Available online: https://clinicaltrials.gov/study/NCT03012620
- Identifier NCT03753919, Durvalumab Plus Tremelimumab for the Treatment of Patients with Progressive, Refractory Advanced Thyroid Carcinoma -The DUTHY Trial; 2022 Oct 22 [cited 2023 Oct 30]. Available online: https://clinicaltrials.gov/study/NCT03753919
- Di Molfetta S, Dotto A, Fanciulli G, et al. Immune Checkpoint Inhibitors: New Weapons Against Medullary Thyroid Cancer? Front Endocrinol (Lausanne) 2021;12:667784. [Crossref] [PubMed]
- Lorch JH, Barletta JA, Nehs M, et al. A phase II study of nivolumab (N) plus ipilimumab (I) in radioidine refractory differentiated thyroid cancer (RAIR DTC) with exploratory cohorts in anaplastic (ATC) and medullary thyroid cancer (MTC). Journal of Clinical Oncology 2020;38:6513. [Crossref]
- Timmerman JM, Levy R. Dendritic cell vaccines for cancer immunotherapy. Annu Rev Med 1999;50:507-29. [Crossref] [PubMed]
- Stift A, Sachet M, Yagubian R, et al. Dendritic cell vaccination in medullary thyroid carcinoma. Clin Cancer Res 2004;10:2944-53. [Crossref] [PubMed]
- Bachleitner-Hofmann T, Friedl J, Hassler M, et al. Pilot trial of autologous dendritic cells loaded with tumor lysate(s) from allogeneic tumor cell lines in patients with metastatic medullary thyroid carcinoma. Oncol Rep 2009;21:1585-92. [Crossref] [PubMed]
- Papewalis C, Wuttke M, Jacobs B, et al. Dendritic cell vaccination induces tumor epitope-specific Th1 immune response in medullary thyroid carcinoma. Horm Metab Res 2008;40:108-16. [Crossref] [PubMed]
- Schott M, Seissler J, Lettmann M, et al. Immunotherapy for medullary thyroid carcinoma by dendritic cell vaccination. J Clin Endocrinol Metab 2001;86:4965-9. [Crossref] [PubMed]
- Del Rivero J, Donahue RN, Marté JL, et al. A Case Report of Sequential Use of a Yeast-CEA Therapeutic Cancer Vaccine and Anti-PD-L1 Inhibitor in Metastatic Medullary Thyroid Cancer. Front Endocrinol (Lausanne) 2020;11:490. [Crossref] [PubMed]
- Chatal JF, Campion L, Kraeber-Bodéré F, et al. Survival improvement in patients with medullary thyroid carcinoma who undergo pretargeted anti-carcinoembryonic-antigen radioimmunotherapy: a collaborative study with the French Endocrine Tumor Group. J Clin Oncol 2006;24:1705-11. [Crossref] [PubMed]
- Kraeber-Bodéré F, Rousseau C, Bodet-Milin C, et al. Targeting, toxicity, and efficacy of 2-step, pretargeted radioimmunotherapy using a chimeric bispecific antibody and 131I-labeled bivalent hapten in a phase I optimization clinical trial. J Nucl Med 2006;47:247-55. [PubMed]