Metformin as a therapeutic tool for resistant hematological malignancies: a literature review
Introduction
Cancer is a worldwide public health problem responsible for 10 million deaths in 2020, corresponding to one in six deaths in the world (1). According to origin, cancers can be classified as hematological or solid neoplasms (2). HMs are a group of neoplasms associated with an uncontrolled growth of hematopoietic and lymphoid tissues, due to the loss of normal hematopoietic function (3,4). HMs are responsible for 1.2 million cases per year in the world and correspond to 7% of diagnosed cancers (5), which are generally divided into three types: leukemia, lymphoma, and multiple myeloma (MM) (6). HMs treatment evolved over the years and currently involves chemotherapy, targeted therapies, immunotherapies, chimeric antigen receptor T-cells (CAR-T cells), radiotherapy, and hematopoietic stem cell transplant (HSCT) (5,7). Despite the evolution of the treatment and prognostic of HMs patients, many patients deal with treatment resistance and recurrence (5). The loss of treatment efficiency is linked to cell adaptations for survival, which involves mutations and modulation of cell processes, such as apoptosis, autophagy, proliferation, and metabolism (5). Another important tool for HMs resistance/relapse is CSCs, mainly for leukemias and MM (7,8). Therefore, new therapeutic strategies are necessary to overcome the treatment failure.
Metformin is a biguanide drug and an anti-hyperglycemic agent, approved by U.S. Food and Drug Administration (FDA) for the treatment of type 2 diabetes mellitus (T2DM) in 1994, being considered the first-line treatment for T2DM due to its efficacy (9,10). In addition to being an anti-diabetic drug, studies have shown the action of metformin in multiple pathways in cancer, highlighting its potential use in the treatment of different types of cancer, including lung (11,12), breast (13,14), colorectal (15,16) and ovarian cancer (17). The number of studies that have been evaluating metformin use in the therapy of HMs is increasing, highlighting its capacity to overcome drug resistance in HMs (18-20). In this paper the antitumoral mechanisms of metformin will be reviewed and its potential application on resistant HMs will be discussed. We present this article in accordance with the Narrative Review reporting checklist (available at https://cco.amegroups.com/article/view/10.21037/cco-23-157/rc).
Methods
We performed a literature review using PubMed to understand metformin’s action in cancer and evaluate its potential application in HMs, including in resistant, relapse, and refractory diseases. Studies published between 1996 and 2023 were considered for this review. To explore metformin use in clinical, clinical trials submitted to clinicaltrials.gov about metformin use in resistant/relapse/refractory HMs were considered. Only studies published in English were evaluated (Table 1).
Table 1
Items | Specification |
---|---|
Date of search | October 10th–November 26th, 2023 |
Databases and other sources searched | PubMed, clinicaltrials.gov |
Search terms used | Metformin and mechanisms of action |
Metformin and hematological tumors | |
Metformin and resistant leukemias | |
Metformin and resistant lymphomas | |
Metformin and resistant multiple myeloma | |
Epidemiology and hematological malignancies | |
Metformin and cancer stem cells | |
Timeframe | 1996–2023 |
Inclusion criteria | Research and review articles and clinical trials were considered. Studies published in English were considered |
Selection process | The selection of the data present in this paper was made by G.S.P. and J.F. |
Metformin and its antitumoral mechanisms of action
Metformin is a biguanide drug, first synthesized in 1922 by Werner & Bell from a plant called Galega officinalis (21). Associated with its anti-hyperglycemic action, low cost, and tolerance (low risk of lactic acidosis), metformin is considered the first-line therapy for T2DM patients (22). In 2005, Evans et al. published an innovative study, that highlighted for the first time the potential antitumoral effects of metformin by the demonstration that T2DM patients under metformin use have a lower risk of developing cancer compared to other diabetic patients that not used metformin (23). This study opened new ways for the use of metformin, motivating the scientific community to investigate the role of metformin in cancer. Nowadays, metformin is known as a potential antitumoral drug, acting in different kinds of cancer and under investigation in 440 cancer clinical trials, including in hematological tumors.
Metformin is a hydrophilic and cation drug on physiological pH, requiring transporters to cross cell membranes, such as solute carrier organic transporters, enabling its action in cells (24). The main transporters involved with metformin transport are (I) the plasma membrane monoamine transporter (PMAT), (II) organic cation transporters (OCTs) 1–3 and, (III) the multidrug and toxin extrusion 1–2 (MATE 1/2) (24,25); PMAT and OCT1 mediates the gastrointestinal uptake of metformin. Once in the bloodstream, OCT1 promotes hepatic uptake and OCT3 is important for muscle uptake (24). As metformin is not metabolized, its excretion depends on kidney elimination, for this metformin enters the kidney through OCT2 and is excreted in urine by MATE 1/2 (24). The transport of metformin is essential for its mechanisms of action in cancer cells, which is generally divided into indirect (related to metformin’s anti-hyperglycemic effect) and direct effects.
Indirect effects
Metformin could exert its anti-cancer action through its anti-diabetic effects, which are associated with the reduction of blood glucose and insulinemia, important factors in tumorigenesis. Metformin improves insulin sensitivity, reducing insulinemia and insulin growth factor-1 (IGF-1), which promotes cancer progression (26). Associated with the reduction of insulin levels, the inhibition of hepatic glucose production, and the higher uptake of glucose by skeletal muscle, the drug reduces blood glucose (26) (Figure 1). Through the reduction of blood glucose and insulin levels, metformin impairs inflammation and the activation of the phosphatidylinositol-3-kinase/Akt and mammalian target of rapamycin (PI3K/Akt/mTOR) pathway, important to tumorigenesis (9).
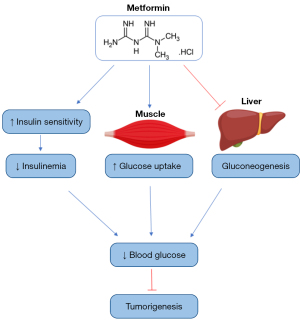
Direct effects (tumor cell metabolism)
Metformin acts through modulation of tumor cell energetic metabolism, mainly through adenosine monophosphate (AMP)-activated protein kinase (AMPK) activation (9). In 2000, Leverve group discovered that metformin inhibits the complex I (CI) of the electron transport chain (ETC), impairing the electron flow throughout the ETC, reducing NADH oxidation (enhancing NADH/NAD ratio), leading to the loss of mitochondrial membrane potential (MMP), reactive oxygen species (ROS) production, impairment of oxidative phosphorylation (OXPHOS), diminishing oxygen consumption and decreasing adenosine triphosphate (ATP) levels, which increases the AMP/ATP or adenosine diphosphate (ADP)/ATP ratio (27,28). The most acceptable explanation for metformin action in mitochondria is related to the positive charge of the drug, enabling its interaction with the MMP, drug accumulation in the mitochondrial matrix, and its action (29,30). Different from its similar, rotenone which is an irreversible inhibitor of CI, metformin is known as a reversible inhibitor (29).
As a result of ATP depletion, metformin activates AMPK to restore energy homeostasis, leading to a change from anabolic to catabolic status, impairing the synthesis of proteins, lipids, and nucleotides, important for cancer growth (3,22). Although AMPK is directly activated as a consequence of ATP depletion, AMPK can be activated indirectly by the liver kinase B1 (LKB1), an upstream kinase that promotes AMPK activation through the phosphorylation of alpha (α) AMPK subunit, and through lysosomal pathway, where metformin binds to presenilin enhancer-2 (PEN2), making a complex that interacts with ATP6AP1 (V-ATPase subunit), inhibiting V-ATPase and activating AMPK (31,32). Consequently through AMPK activation, occurs: (I) the inhibition of mTOR, which mediates protein synthesis and cancer growth (33,34), (II) activation of p53, mediating cell cycle arrest, autophagy and apoptosis (35,36), (III) inactivation of acetyl-CoA carboxylase (ACC), reducing lipogenesis (37,38) and (IV) inhibition of adenylate cyclase (AC), histone deacetylase (HDAC) and CREB-regulated transcription coactivator 2 (CRTC2), which are involved with the reduction of gluconeogenesis genes transcription (22).
Associated with the increase of NADH/NAD ratio, metformin reduces the tricarboxylic acid (TCA) cycle and its intermediates (39), which are used for cancer cells to energy production and macromolecule biosynthesis (40). Therefore, through AMPK activation, metformin impairs the energetic metabolism of cancer cells and the biosynthesis of macromolecules, processes that are essential for cancer progression.
Independent of AMPK activation, metformin inhibits the mitochondrial glycerol-3-phosphate (G3P) dehydrogenase (mGPDH), that forms with its cytosolic version the glycerol-phosphate shuttle (41). mGPDH acts in the oxidation of G3P to dihydroxyacetone phosphate (DAP) associated with the reduction of FAD to FADH2, transferring the electrons to coenzyme Q of ETC, helping the maintenance of MMP and thus the ETC function (42). Through this inhibition, occurs the reduction of gluconeogenesis from glycerol and the increase of NADH/NAD ratio (43) (Figure 2).
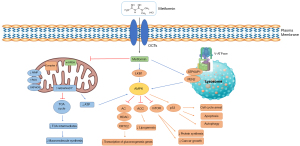
Another important effect of metformin is its action in CSCs. CSCs are a group of cancer cells with unlimited self-renewal capacity, frequently linked to tumor recurrence, resistance to treatment, and metastasis, because many antitumoral treatments are unable to kill CSCs (44). Despite this, there is an increasing number of studies that demonstrate the capacity of metformin to inhibit CSCs in different cancers, including gastric (45), breast (46), and ovarian (47) cancers. Through its action in CSCs, metformin can be a tool for avoiding cancer resistance and metastasis, which are recurring problems among oncology patients and the main causes of treatment failure and cancer deaths (48) (Figure 3).
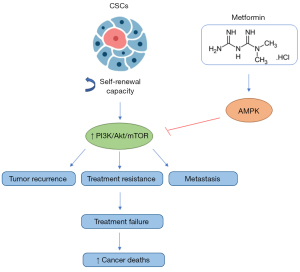
Linked to metformin’s antitumoral properties, metformin is being used with other cancer treatments to achieve a synergistic effect (49-51), beyond its use in the resensitization of drugs, being able to reverse resistance cases (52,53).
Metformin as a therapeutic approach for resistant hematological tumors
HMs consist of a group of tumors with hematopoietic origin and are currently divided into leukemias, lymphomas, and MM (54,55). Despite the available therapeutic approaches’ efficiency, many HMs become resistant to therapy in distinct ways, considered an important obstacle to a successful treatment (56,57). The resistance can be intrinsic, when the cells are resistant before the treatment, or acquired, characterized by the cells’ acquisition of resistance during the therapy (57-60). Beyond that, the causes of cancer resistance to therapy are classified as host factors, tumor factors, and tumor-host interactions (59). The resistance related to host factors, includes: (I) patient’s genetics and habits, (II) genetic variants, and (III) drug-drug interactions (DDIs) (60-64). Tumor factors refer to alterations in the drug targets (65-67), diminished drug concentration inside the cell (68-74), impairment of the regulation of cell death pathways (75-77), enhanced of DNA repair mechanisms (78,79), epigenetic modifications (80,81), intratumoral heterogeneity (82) and CSCs (83). The interaction between tumor and its microenvironment (TME) and low pH TME are elements linked to resistance caused by tumor-host interactions (60,84). Given this scenario of HMs resistance, new therapeutic approaches are necessary. Below, the potential use of metformin in different resistant HMs will be presented.
Leukemias
Leukemia is a malignancy resulting from the dysregulated proliferation of leukocytes. This malignancy is classified as acute or chronic, according to its proliferation profile, and in myeloid or lymphoid, related to the cell origination. This classification divided leukemias into four types: acute myeloid leukemia (AML), chronic myeloid leukemia (CML), acute lymphoblastic leukemia (ALL), and chronic lymphoblastic leukemia (CLL) (85). Leukemia is responsible for 2.5% of the diagnosed cancers, affecting approximately 400,000 people per year (5).
CML
CML is a malignant hematological disorder cytogenetically characterized by the Philadelphia (Ph) chromosome, formed through a reciprocal translocation between the chromosomes 9 and 22, t(9;22) (q34;q11), resulting in the formation of BCR-ABL1 oncogene (86,87). This oncogene promotes the activation of the tyrosine kinase pathway, an important step for leukemogenesis (88). Worldwide, CML had an incidence rate nearly of 1 per 100,000 people in 2018, making CML responsible for around 15% of leukemia cases in adults (89). This incidence rate increases among older people, as the median age of diagnosis is 56 years old (90). The available therapies for CML involve chemotherapy, immunotherapy by interferon-alpha (IFN-α), stem cell transplant, and tyrosine kinase inhibitors (TKIs) (91). The use of TKIs revolutionized CML treatment, so that imatinib (first-generation TKI) is used as first-line therapy for CML due to its efficiency (92). Although its action, around 10% of patients develop resistance to imatinib, for these patients second-(dasatinib, nilotinib, bosutinib, and radotinib) and third-generation TKIs as ponatinib can be employed (92-95). The main cause of imatinib resistance is point mutations in BCR-ABL1 oncogene, among these mutations, T315I is considered the most challenging, and only the third-generation TKI, ponatinib can act against this mutation (92,96). Despite ponatinib action, the use of this TKI can result in cardiovascular thrombotic events (97). This scenario evidences the importance of new therapies to treat resistant CML, especially to overcome the T315I mutation.
The achievement of complete cytogenetic response (CcyR), characterized by less than 1% Ph chromosome-positive cells, is an important step to avoid relapse (98), but only 66–88% of patients achieve CcyR after one year under TKI treatment (Table 2) (99). Pokorny et al. developed a clinical trial where 59 CML patients were divided into two groups: the metformin group (TKI and metformin concomitant use—5 patients) and the non-metformin group (54 patients—TKI therapy alone). The metformin group achieved 100% CcyR against 73.6% in the non-metformin group. In relation to major molecular response (MMR; BCR-ABL gene in blood or bone marrow is 1/1,000 or less) and complete molecular response (CMR; BCR-ABL gene is not present), the median time to achieve these parameters was 11.1 months in metformin group and 19.5 months in non-metformin group for MMR, and 37.4 months for CMR in metformin group (in non-metformin group CMR was not observed) (Table 2) (99). This study indicates that the concomitant use of metformin and TKIs enhances treatment success, increasing the chance of CcyR and reducing the time to achieve MMR and CMR, important factors to avoid a relapse/treatment failure.
Table 2
Disease | Type of study | Co-treatment | Results | References |
---|---|---|---|---|
CML | Clinical | TKI | Metformin induces 100% of CcyR and takes 11.1 months to achieve MMR and CMR | (99) |
CML | Clinical/in vitro | – | Metformin acts against positive cells line for Ph chromosome and primary leukemia cells of patients (including those harboring T315I mutation) | (100) |
CML | In vitro | Imatinib | Metformin resensitizes resistant CML cells to imatinib and their combination promoted a higher inhibition of proliferation and cell viability than imatinib alone | (101) |
CML | In vitro | Imatinib | Metformin acts against MDR CML cell line (K562-Lucena) and enhances imatinib cytotoxicity | (102) |
CML | In vitro | TQ | Metformin + TQ promotes a stronger effect than in monotherapy, reducing proliferation and promoting apoptosis of imatinib resistant cells | (103) |
CML | In vitro | Nilotinib | Metformin resensitizes cells to nilotinib through reduction of JNK phosphorylation and enhances nilotinib effect by reducing Bcl-Xl expression | (104) |
CML | In vitro | Vincristine | Metformin sensitizes CML cells to vincristine, enhancing apoptosis by AMPK activation | (105) |
CLL | In vitro | Ritonavir | Metformin + ritonavir affect CLL viability, decreasing OXPHOS and glycolysis, indicating an alternative to CLL treatment | (106) |
CLL | In vitro | Fludarabine and ABT-737 | Metformin acts as a cytotoxicity drug in CLL and enhances fludarabine and ABT-737 effect in CLL cells | (107) |
CML, chronic myeloid leukemia; CLL, chronic lymphocytic leukemia; TKI, tyrosine kinase inhibitor; CcyR, complete cytogenetic response; MMR, major molecular response; CMR, complete molecular response; Ph, Philadelphia; MDR, multidrug resistance; TQ, thymoquinone; JNK, jun kinase; AMPK, adenosine monophosphate-activated protein kinase; OXPHOS, oxidative phosphorylation.
The resistance to imatinib is increasing and overcoming this scenario is a challenge. In this context, Vakana et al. showed for the first time the capacity of metformin to act against cell lines positive for Ph chromosome and primary leukemic cells of CML patients, including those harboring T315I-BCR-ABL mutation (Table 2) (100). Due to the potential antileukemic effect of metformin, Shi et al. analyzed if metformin was able to restore the imatinib effect in the imatinib-resistant CML cell line (K562R). In this study, K562R cells were more sensitive to metformin than its parental cell line (K562) and the metformin + imatinib combination promoted a better inhibition of proliferation and cell viability than imatinib alone. Metformin also resensitized K562R cells to imatinib (Table 2) (101) (Figure 4A).
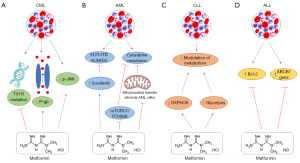
Multidrug resistance (MDR) is a factor responsible for treatment failure and the expression of P-glycoprotein (P-gp) is often related to MDR. P-gp is an ATP-dependent efflux pump that mediates the drug transport to the cell outside, reducing drug concentration inside the cell (108). Curvello et al. demonstrated that metformin acts against the MDR CML cell line (K562-Lucena) and enhances imatinib cytotoxicity when in combination, probably by the reduction of P-gp expression (Table 2) (102) (Figure 4A).
Aiming to find an alternative for imatinib-resistant CML, Glamoclija et al. showed that metformin and thymoquinone (TQ), both at monotherapy and combination therapy, reduced the viability and proliferation and promoted apoptosis of CML cells, including those resistant to imatinib. Highlighting a stronger effect of the combination in reducing proliferation and apoptosis of imatinib-resistant CML cells (Table 2) (103). Indicating metformin and TQ combination as an alternative therapy in the context of imatinib resistance.
Although imatinib is considered the first-line therapy for CML, the second-generation TKI nilotinib, has a similar action to imatinib and a stronger effect both in imatinib-sensitive and -resistant CML patients, possibly its use in CML treatment (Table 2) (104). Despite nilotinib efficiency, the number of resistance cases is increasing and needs attention (109,110). Na et al. showed for the first time that jun kinase (JNK) overexpression is related to nilotinib resistance in CML cell lines. As metformin is a known regulator of JNK pathway, the study demonstrated the reduction of the JNK phosphorylation in nilotinib-sensitive and resistant cells by metformin, resensitizing cells to nilotinib (Figure 4A). Metformin also enhances nilotinib cytotoxicity through the lower expression of the anti-apoptotic protein Bcl-Xl (Table 2) (104).
Vincristine chemotherapy is an approach used to treat leukemias, including CML. Despite this, its toxicity and resistance are common problems related to vincristine use (105). Yi et al. showed that metformin sensitizes leukemia cells (K562-CML and A301-ALL) to vincristine, enhancing apoptosis in an AMPK-dependent manner (Table 2) (105). This study proposes metformin as an adjuvant therapy to vincristine in leukemia treatment.
CML leukemia stem cells (LSCs) are considered the progenitors of CML cells, responsible for the chronic phase of CML and its recurrence (111). CML LSCs are resistant to TKIs, including imatinib, nilotinib, and dasatinib, so that 50% of relapse CML patients are linked to LSCs action (7,112). Due to that, drugs that can act in LSCs are promising candidates to avoid CML recurrence. As demonstrated by different studies, metformin inhibits CSCs in many cancers, including in AML (113). Purposing metformin as a therapeutic approach to act against LSCs, possibly the reduction of CML recurrence and the enhancement of survival.
AML
AML is the main leukemia type among adults, being responsible for 80% of leukemia cases. It is caused by a clonal expansion of immature blast cells in the peripheral blood and bone marrow (114). Due to the treatment evolution, involving chemotherapy, HSCT, immunotherapy, and target therapy, the number of AML patients that achieve complete remission (CR) is increasing (115). The first-line therapy for AML is the combination of daunorubicin (DA)/idarubicin (IDA) with cytosine arabinoside (cytarabine, Ara-C), with a CR rate of 60–80% in young adults and 40–60% in older adults (>65 years old) (115-117). Despite therapy evolution and the efficiency of the first-line treatment, many patients failed therapy and relapsed after CR, mainly among older people (19,115) (Table 3). The treatment failure is often related to drug resistance, which can be caused by distinct resistant mechanisms, as (I) drug resistance-related protein and enzyme, like P-gp and multidrug resistance-related protein (MRP1) that reduces drug accumulation in the cell; (II) genetic modifications; (III) miRNAs alterations in drug resistance and (IV) extensive activation of drug resistance-related signal pathway, like PI3K/Akt (115).
Table 3
Disease | Type of study | Co-treatment | Results | References |
---|---|---|---|---|
AML | In vitro/in vivo | Cytarabine | Metformin sensitizes AML cells to cytarabine by the inhibition of mitochondrial transfer between stromal and AML cells | (19) |
AML | In vitro | Sorafenib | Metformin synergistically increases sorafenib effect through mTOR inhibition in FLT3-ITD AML cells | (118) |
AML | In vitro/in vivo | Cytarabine | Metformin potentializes cytarabine effect in AML in vitro and in vivo through mTORC1/P70S6K pathway | (119) |
ALL | In vitro | – | Metformin promotes cell cycle arrest and apoptosis of 10E1-CEM cells (resistant) with Bcl-2 overexpression | (120) |
AML, acute myeloid leukemia; ALL, acute lymphoblastic leukemia; mTOR, mammalian target of rapamycin; FLT3-ITD, fms-like tyrosine kinase 3-internal tandem duplication; mTORC1, mammalian target of rapamycin complex 1.
The mutation of fms-like tyrosine kinase 3-internal tandem duplication (FLT3-ITD) found in 30% of AML patients is associated with poor prognosis and is considered a relapse-related genetic marker (Table 3) (118). To treat FLT3-ITD AML is used sorafenib, a kinase inhibitor that acts against FLT3 (121). Although its efficiency in FLT3-AML, sorafenib use as monotherapy can cause resistance (Table 3) (118). Wang et al. showed that metformin acts on FLT3-ITD AML cells and synergistically increases the sorafenib effect through the inhibition of mTOR and promotion of apoptosis and autophagy of these cells (Table 3) (118). Therefore, metformin potentializes the anti-FLT3-ITD AML effect of sorafenib, possibly overcoming sorafenib resistance in FLT3 AML cells (Figure 4B).
Cytarabine has been used as the first-line treatment for AML since 1973, despite its efficiency with high CR rates, many patients relapse and 80% of them do not have an efficient alternative therapy, leading to death (122). Yuan et al. demonstrated an improvement of cytarabine anti-AML effect through inhibition of mTORC1/P70S6K pathway by metformin, leading to cell cycle arrest in S phase in vitro and showed a similar synergetic effect with cytarabine in AML mice (Table 3) (119) (Figure 4B). By potentializing the cytarabine effect, metformin constitutes a promising therapy to overcome that resistance and thus enhance the survival of AML patients.
Bone marrow microenvironment (BMME) is essential for AML cells and is related to drug resistance, enhancing relapse cases (123,124). The BMME-relapse cases are associated with the mitochondrial transfer from stromal cells to AML cells in bone marrow, which was already observed in other HMs, including AML, ALL, and MM (125-127), an interaction that mediates chemoresistance and relapse. In view of disrupting this interaction, You et al. showed that this interaction and mitochondria transfer between stromal cells and AML cells occurs both in vitro and in vivo, and was responsible for cytarabine resistance. When metformin was applied, AML cells turned sensitive to cytarabine due to the inhibition of mitochondrial transfer between stromal and AML cells, in vitro. A similar effect was observed in vivo, where metformin increases Ara-C anti-AML action by disrupting the interaction of BMME and AML cells (Table 3) (19). Thus, blocking mitochondrial transfer from stromal BM to AML cells, as done by metformin, is a potential mechanism to overcome chemotherapy resistance (Figure 4B).
P-gp and MRP1 are ATP-binding cassette (ABC) transporter proteins frequently associated with AML resistance through the decrease of drug concentration in cells (128). Possibly due to the decrease of ATP content, metformin can reduce the expression of P-gp and MRP1, as showed in some types of cancer (129-131). Beyond its action on ABC transporters, metformin as a metabolic disruptor can reduce the activation of the PI3K/Akt pathway in cancer (132). Considering the importance of ABC transporters and PI3K/Akt pathway for AML resistance, which are targets of metformin, evaluating the action of metformin in these factors can be a potential way to overcome AML resistance by metformin.
LSCs can be responsible for chemotherapy resistance in AML. Studies have shown that therapy-resistant leukemia cells, known as minimal residual disease (MRD) in LSCs are currently related to resistance cases (133). Therefore, there are necessary therapies that can eliminate these cells. As shown in other studies, metformin can kill CSCs, like AML CSCs (113). Thus, metformin’s possible contribution to eliminating MRD-LSCs needs investigation.
Linked to the potential use of metformin in resistant AML, a phase 2 clinical trial (NCT05854966) is on course to evaluate its effects with CPI-613 in relapsed/refractory AML (Table 4). Highlighting metformin as a tool to overcome AML drug resistance.
Table 4
Disease | Co-treatment | Objective | Phase | ClinicalTrials.gov ID |
---|---|---|---|---|
AML | CPI-613 | Effect of the combination treatment with metformin + CPI-613 in relapse/refractory AML | 2 | NCT05854966 |
MM/CLL | Ritonavir | Identify the correct dose and side effects of metformin + ritonavir combination in relapsed/refractory MM or CLL | 1 | NCT02948283 |
CLL | – | Evaluation of metformin action in relapse/untreated CLL | 2 | NCT01750567 |
ALL | VXLD | Evaluate the MTD and effect of metformin + VXLD combination therapy in relapsed ALL | 1 | NCT01324180 |
ALL | Standard induction remission chemotherapy | Evaluate the effect of metformin + standard induction remission chemotherapy in the expression of AMPK/ABCB1 genes in ALL | – | NCT05326984 |
DLBCL/FL3 | – | Evaluate the effect of metformin in complete remission DLBCL/FL3 patients with high risk for relapse | 2 | NCT03600363 |
MM | Dexamethasone | Evaluate the activity of metformin + dexamethasone in relapsed/refractory MM | 2 | NCT02967276 |
MM | Nelfinavir + bortezomib | Find the best dose and side effects of metformin + nelfinavir + bortezomib combination therapy in relapsed/refractory MM | 1 | NCT03829020 |
ALL | Prednisone | Evaluate the effect of metformin + prednisone in the expression of ABCB1 gene in de novo ALL | – | NCT03118128 |
HMs, hematological malignancies; AML, acute myeloid leukemia; MM, multiple myeloma; CLL, chronic lymphocytic leukemia; ALL, acute lymphoblastic leukemia; VXLD, vincristine + dexamethasone + PEG-asparaginase + doxorubicin; MTD, maximum tolerated dose; DLBCL, diffuse large B-cell lymphoma; FL3, follicular lymphoma grade 3.
CLL
CLL is an adult leukemia, characterized by a higher accumulation of dysfunctional lymphocytes in blood, bone marrow, lymph nodes, and spleen (134). In Western countries, CLL represents 25–30% of leukemia cases (135), being responsible for more than 40,000 deaths and with an average incidence of 100,000 cases in 2019, worldwide (134). CLL generally affects elderly people, with a median age of 70 years old, beyond that CLL has an incidence two times higher in men than in women (136-138).
CLL treatment currently involves targeted drugs, including venetoclax (Bcl-2 inhibitor) and bruton tyrosine kinase inhibitors (BTK), like ibrutinib and zanubrutinib (139-142). Although treatment evolution and efficiency, the development of drug resistance and relapse are increasing, making this disease considered incurable (143). Genetic alterations have an important contribution to CLL relapses, deletions of TP53 and ataxia telangiectasia mutated (ATM) at the 17p13 and 11q22-q23 chromosomal regions are frequently found in relapsed/refractory patients (144). In this context, new drugs are necessary to improve CLL survival.
Targeting cancer metabolism and its adaptations are very important in anti-tumoral therapy, such as for CLL treatment. Adekola et al. showed that the combination of metformin (CI inhibitor) and ritonavir (GLUT4 inhibitor) affects CLL viability, reducing oxygen consumption rate (OCR) and extracellular acidification rate (ECAR), indicating impairment of OXPHOS and glycolysis, and thus of CLL metabolism (106) (Table 2). Showing metformin + ritonavir combination as an alternative therapy to CLL. In the study of Bruno et al., metformin was shown as a cytotoxicity agent for CLL and potentialized fludarabine and ABT-737 action in CLL cells (107) (Table 2). Purposing metformin as a potential alternative therapy for CLL, both in monotherapy and combination therapy (Figure 4C).
Currently, two clinical trials are on course associated with the use of metformin in relapsed CLL. One is a phase 1 study of the combination therapy between metformin and ritonavir in relapsed/refractory CLL (NCT02948283), and the other is a phase 2 study about the use of metformin in relapsed or untreated CLL (NCT01750567) (Table 4).
ALL
ALL is characterized by an uncontrolled proliferation of lymphocytes, which can disseminate to bone marrow, blood, and extramedullary sites (145). This leukemia occurs mainly in children, with a high incidence between 1–4 years old, so the probability of developing ALL tends to fall with increasing age (145). In the USA, nearly 5,960 ALL cases were diagnosed, and 1,470 deaths were registered in 2018 (145,146). Although the treatment advances, with an increase of 5-year overall survival, in adults with 50 years old or more, just 25% of them live for 5 years after the diagnosis (147-149). The treatment of ALL involves four steps: induction, which involves glucocorticoid, vincristine, L-asparaginase and anthracycline; consolidation, with cytarabine, methotrexate, vincristine, asparaginase, mercaptopurine and glucocorticoids; intensification (like induction) and maintenance, which involves mercaptopurine, methotrexate, vincristine and glucocorticoids (145). Despite the treatment advances, the rate of successfully therapy in adults is 30–40%, while in children is nearly 90% (150,151). This lower cure rate in adults adding to the resistant ALL cases indicates the necessity of new therapeutical approaches.
Bcl-2 overexpression in ALL is commonly related to drug resistance (152-156), therefore Rodríguez-Lirio et al. showed that metformin promotes cell cycle arrest and apoptosis in 10E1-CEM cells, harboring Bcl-2 overexpression and drug resistance (120) (Figure 4D) (Table 3). Another important factor for ALL resistance and lower survival is the overexpression of the ABCB1 gene (Pgp encoder), mediating drug extrusion and lower accumulation in cells (157-159). In a clinical trial (NCT03118128) with 102 ALL patients, divided into metformin users (n=26, receiving metformin + prednisone) and metformin non-users (n=76, in prednisone treatment), Ramos-Peñafiel et al. demonstrated metformin as a protective agent against drug failure and relapse in ALL patients with high ABCB1 expression, leading to an increase of the patient’s survival (18) (Figure 4D). In a clinical trial by Trucco et al. (NCT01324180) with 14 relapsed/refractory ALL patients (only 9 patients were evaluated), metformin was used with vincristine, dexamethasone, PEG-asparaginase, and doxorubicin (VXLD) for 28 days and was able to induce 5 CR, 1 partial response and 1 stable disease (160). Indicating metformin as an adjuvant therapy in resistant ALL (Table 4).
Associated with the importance of AMPK gene expression for antitumoral effects and the relationship between the ABCB1 gene and drug resistance in ALL, a clinical trial (NCT05326984) with adolescents harboring ALL is on a recruiting stage to evaluate the capacity of metformin added to standard induction remission chemotherapy to reduce ABCB1 and increase AMPK gene levels (Table 4). The success of this study will indicate metformin as a tool to improve ALL treatment and reduce the relapse rate of ALL.
Lymphoma
Lymphoma is a malignancy resulting from an uncontrolled proliferation of lymphocytes and is classified as Hodgkin lymphoma (HL) or non-Hodgkin lymphoma (NHL), which represent 10% and 90% of lymphomas, respectively (161,162). Moreover, HL can be classical or nonclassical type and NHL can be divided into B-cell, T-cell, or natural killer (NK) cell types (162). In the USA (2009–2013) the incidence of lymphoma was 22/100,000 people with an average age of diagnosis of 63 years old (162). Although the improvement of survival rate is 72% at 5 years (162), cases of resistant and relapse lymphomas are increasing, thus, new therapies to overcome this scenario are essential.
mTOR pathway is currently activated in lymphomas, being important for tumorigenesis and drug resistance (163-167). Shi et al. showed a higher mTOR activation in B- and T-lymphoma cells associated with AMPK inactivation. When treated with metformin, AMPK was activated leading to the inhibition of mTOR and proliferation of both T and B lymphoma cells, similar effects were also observed in vivo (168) (Figure 5) (Table 5). In the study, metformin was also able to enhance the anti-lymphoma action of doxorubicin in vitro and in vivo (168) (Table 5).
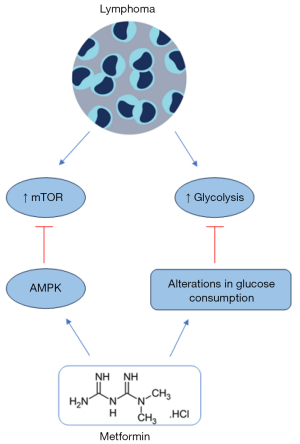
Table 5
Type of study | Co-treatment | Results | References |
---|---|---|---|
In vitro/in vivo | Doxorubicin | Metformin activates AMPK and promotes the inhibition of mTOR (resistance marker) in T- and B-lymphoma cells and in vivo metformin potentializes doxorubicin effect in vitro and in vivo | (168) |
Clinical | Rituximab + CHOP | Metformin + rituximab + CHOP enhance the survival of low and high risk for relapse patients and decrease relapse rate | (169) |
In vitro/in vivo | Doxorubicin, dexamethasone, rituximab | Metformin acts in resistant lymphoma cells and enhances doxorubicin and dexamethasone effect in vivo, metformin potentializes rituximab action, enhancing animals survival | (170) |
In vitro | – | Metformin reduces the viability of BL cells and induces alterations in glucose consumption, important for drug resistance | (171) |
AMPK, adenosine monophosphate-activated protein kinase; mTOR, mammalian target of rapamycin; CHOP, cyclophosphamide, doxorubicin, vincristine and prednisone; BL, Burkitt lymphoma.
Diffuse large B-cell lymphoma (DLBCL) is responsible for 35–40% of NHL cases, and similar to DLBCL is the follicular lymphoma grade 3b (FL3b), a type of NHL with the same therapy for DLBCL (169) (Table 5). The first-line treatment involves the use of rituximab + CHOP (cyclophosphamide, doxorubicin, vincristine, and prednisone), despite its efficiency rate of 75–80%, nearly 30–40% of responsiveness patients relapse (172,173). To overcome the relapse of DLBCL patients, autologous stem-cell transplantation (ASCT) can be used, however, this therapy is toxic for older and weakened patients (172). Therefore, therapeutic agents that can reduce the relapse rate of DLBCL patients are interesting. Fan et al. conducted a phase 2 clinical trial where 245 CR patients with DLBCL or FL3b have treated with/out metformin + rituximab + CHOP, resulting in an enhancement of survival time in both low and high risk for relapse patients and decreased the relapse rate when compared to non-metformin group (5% vs. 9.2%) (169) (Table 5).
Singh et al. (170) performed an in vitro study with lymphoma cell lines, sensitive (RSCL) and resistant (RRCL) to rituximab. The treatment with metformin reduced cell viability, proliferation, ATP levels and induced apoptosis, loss of MMP, ROS generation, and cell cycle arrest in the G1 phase, as monotherapy both in RSCL and RRCL cells (172). Metformin also acts as an adjuvant agent, increasing doxorubicin and dexamethasone anti-lymphoma effect both in RSCL and RRCL. In vivo, metformin exerts an adjuvant effect, enhancing the anti-lymphoma activity of rituximab and the survival time of animals, which was 72.5 days with metformin and 51.5 days without metformin (170) (Table 5). Highlighting the capacity of metformin to resensitize resistant lymphoma cells to therapy, beyond its potential use as monotherapy in sensitive and resistant lymphomas.
Burkitt lymphoma (BL) is a rare and aggressive NHL. Although therapies advance, the relapse of BL can occur and new options to treat BL are necessary (174). Bagaloni et al. demonstrated that DAUDI cells (BL) have a higher rate of aerobic glycolysis, which is linked to the Warburg effect, but when metformin was applied it resulted in reduced cell viability and alterations in glucose consumption (171) (Table 5). A high rate of glycolysis is important for tumorigenesis and is associated with drug resistance and tumor recurrence (175,176). Therefore, through modifications of glucose usage, metformin can limit drug resistance and cases of relapse, constituting an alternative therapeutic tool for aggressive lymphoma (Figure 5).
Due to the good perspective of the metformin uses to avoid relapse, a phase 2 clinical trial of metformin use as maintenance therapy for DLBCL and stage 3 follicular lymphoma patients in CR with high risk of relapse is on course (NCT03600363) (Table 4).
MM
MM is a HM characterized by a clonal proliferation of plasma cells, which are mature antibody-producing B cells found in the bone marrow and important for humoral immunity, promoting monoclonal antibody production and organ injury (177). MM was responsible for 160,000 cases in 2018, corresponding to 0.9% of all cancers, and 106,000 deaths (1.1% of cancer deaths), indicating a high mortality rate (177). The median age of diagnosis is around 65–74 years old, whereas its occurrence among people under 40 years old is rare (178). The first-line therapy is autologous hematopoietic stem cell transplantation (HSCT), for patients not indicated to HSCT, triple therapy with proteasome inhibitor, immunomodulator, and steroid drugs (bortezomib, lenalidomide and, dexamethasone), known as VRd therapy is recommended (179,180). Currently, CAR-T cell therapy is approved by the FDA to use as an MM immunotherapy (178). Despite the advances in MM treatment, many MM patients relapse and tend to a refractory disease (181). Associated with the higher mortality and relapse rate, MM is considered a treatable, but incurable disease.
MM has a higher glucose uptake, which is linked to drug resistance in many tumors, such as in MM (182,183). Due to the importance of glycolysis to MM, ritonavir (GLUT4 inhibitor) is used to treat MM, however, resistant cases can occur (184-186). Dalva-Aydemir et al. evaluated the use of another metabolic disruptor, metformin together with ritonavir in MM, resulting in a synergetic cytotoxicity in vitro, beyond the reduction of tumor weight, tumor regressed and a prolonged survival in vivo by this combination. The cytotoxicity effects were associated with the impairment of energetic metabolism of MM (187) (Table 6). Thus, metformin can act as a ritonavir sensitizer in MM (Figure 6).
Table 6
Type of study | Co-treatment | Results | References |
---|---|---|---|
In vitro/in vivo | Ritonavir | Metformin synergistically enhances ritonavir cytotoxicity in vitro and in vivo, acting as ritonavir sensitizer | (187) |
In vitro | Fingolimod (FTY720) | Metformin potentializes FTY720 effect in MM, enhancing apoptosis and promoting ER stress, inhibition of PI3K/Akt/mTOR, loss of MMP and ROS generation | (188) |
In vitro/in vivo | – | Metformin acts as anti-MM drug, blocking proliferation and inducing autophagy, cell cycle arrest in GO/G1 phase, mTORC1/C2 inhibition and AMPK activation in vitro. Similar results were obtained in vivo | (189) |
In vitro/in vivo | Bortezomib | Metformin + bortezomib suppress GRP78 (linked to bortezomib resistance), leading to apoptosis and promoted a stronger action in stem cell-like population (resistant) in vivo | (190) |
In vitro | Melphalan | Metformin enhances melphalan anti-MM effect, improving MM sensitivity to melphalan | (191) |
MM, multiple myeloma; ER, endoplasmic reticulum; PI3K/Akt/mTOR, phosphatidylinositol-3-kinase/Akt and mammalian target of rapamycin; MMP, mitochondrial membrane potential; ROS, reactive oxygen species; mTORC1/C2, mammalian target of rapamycin complex 1/2; AMPK, adenosine monophosphate-activated protein kinase; GRP78, glucose-regulated protein 78.
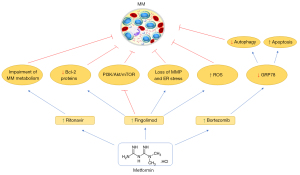
Zhao et al. showed that metformin + FTY720 (fingolimod, an immunomodulator) has a stronger cytotoxicity effect in MM, promoting apoptosis through reducing anti-apoptotic Bcl-2 proteins (including Mcl-1, which is related to the worst prognosis of MM), endoplasmic reticulum (ER) stress, inhibition of PI3K/Akt/mTOR, loss of MMP and ROS generation (188) (Figure 6) (Table 6). Wang et al. presented metformin as a promising anti-MM drug in monotherapy, being able to block MM proliferation through autophagy and induced cell cycle arrest in G0/G1 phase by mTORC1/C2 inhibition and AMPK activation, in vitro. Similar effects were also observed in vivo, where metformin exerts an anti-MM effect associated with AMPK activation, reduced proliferation, and mTOR inhibition (189) (Table 6). Establishing metformin as an adjuvant agent to improve MM therapy.
Despite bortezomib efficacy, cases of resistance are a challenge. Jagannathan et al. discovered in MM patients an upregulation of the HSPA5 gene, which encodes the UPR effector glucose-regulated protein 78 (GRP78) and was related to failed response to bortezomib. The study demonstrated that metformin + bortezomib suppresses GRP78, reducing autophagy and increasing apoptosis (Figure 6). Furthermore, it exerts a higher cytotoxicity in stem cell-like side population (chemoresistant population) and in vivo (190) (Table 6).
Melphalan is an alkylating drug used in the treatment of MM, despite its efficiency, cases of resistance and relapse are common (192,193). Song et al. performed a study where metformin enhanced the anti-MM effect of melphalan, inducing higher DNA damage, apoptosis, and reducing ATP concentration (191) (Table 6). Indicating metformin as a tool to improve MM sensitivity to melphalan.
An important source of drug resistance and relapse in MM is the population of MM cancer stem cells (MM CSCs), which can survive the treatment through drug efflux and detoxifying capacity (181,194). Many studies have demonstrated the action of metformin in CSCs, possibly the inhibition of these cells and the improvement of therapy efficiency through the reduction of drug resistance and recurrence rate among cancers (195,196).
Due to the potential use of metformin in MM, some clinical trials related to its action in relapse MM are in progress. The phase 2 NCT02967276 study evaluates the effect of metformin + dexamethasone in relapsed/refractory MM. The phase 1 NCT03829020 study aims to identify the correct dose and side effects of metformin + nelfinavir + bortezomib treatment in relapsed/refractory MM. Another phase 1 study, NCT02948283, evaluates the effect of metformin + ritonavir in relapsed/refractory MM or CLL (Table 4). Demonstrating metformin as a promised therapeutic approach to relapse/refractory MM patients.
Conclusions
The growing understanding of the metformin mode of action in tumor cell metabolism is central to understanding its adjuvant potential observed in clinical trials. Among several features of growing malignancy for most tumors, is the enhancement of aerobic glycolysis, even in conditions of normoxia. However, in some HMs while some oncogenic signaling favors glycolysis, in other HMs OXPHOS is favored, enhancing sensitivity towards metformin. The metformin action on CI and in AMPK activation may induce apoptosis of T-cell acute lymphoblastic leukemia (T-ALL), while it is less toxic to CD4+ T-lymphocytes from healthy donors. These biochemical features elicited by metformin demonstrated the capacity of metformin to act in HMs, being able to enhance the effect and the sensitivity of anti-HMs drugs, and its action in resistant, relapse, and refractory HMs, both in vitro and in vivo studies.
Acknowledgments
Funding: The present study was supported by
Footnote
Reporting Checklist: The authors have completed the Narrative Review reporting checklist. Available at https://cco.amegroups.com/article/view/10.21037/cco-23-157/rc
Peer Review File: Available at https://cco.amegroups.com/article/view/10.21037/cco-23-157/prf
Conflicts of Interest: Both authors have completed the ICMJE uniform disclosure form (available at https://cco.amegroups.com/article/view/10.21037/cco-23-157/coif). The authors have no conflicts of interest to declare.
Ethical Statement: The authors are accountable for all aspects of the work in ensuring that questions related to the accuracy or integrity of any part of the work are appropriately investigated and resolved.
Open Access Statement: This is an Open Access article distributed in accordance with the Creative Commons Attribution-NonCommercial-NoDerivs 4.0 International License (CC BY-NC-ND 4.0), which permits the non-commercial replication and distribution of the article with the strict proviso that no changes or edits are made and the original work is properly cited (including links to both the formal publication through the relevant DOI and the license). See: https://creativecommons.org/licenses/by-nc-nd/4.0/.
References
- Zheng R, Wang S, Zhang S, et al. Global, regional, and national lifetime probabilities of developing cancer in 2020. Sci Bull (Beijing) 2023;68:2620-8. [Crossref] [PubMed]
- Carbone A. Cancer Classification at the Crossroads. Cancers (Basel) 2020;12:980. [Crossref] [PubMed]
- Zhang N, Wu J, Wang Q, et al. Global burden of hematologic malignancies and evolution patterns over the past 30 years. Blood Cancer J 2023;13:82. [Crossref] [PubMed]
- Tietsche de Moraes Hungria V, Chiattone C, Pavlovsky M, et al. Epidemiology of Hematologic Malignancies in Real-World Settings: Findings From the Hemato-Oncology Latin America Observational Registry Study. J Glob Oncol 2019;5:1-19. [Crossref] [PubMed]
- Auberger P, Tamburini-Bonnefoy J, Puissant A. Drug Resistance in Hematological Malignancies. Int J Mol Sci 2020;21:6091. [Crossref] [PubMed]
- Damlaj M, El Fakih R, Hashmi SK. Evolution of survivorship in lymphoma, myeloma and leukemia: Metamorphosis of the field into long term follow-up care. Blood Rev 2019;33:63-73. [Crossref] [PubMed]
- Zhai X, Jiang X. Properties of Leukemic Stem Cells in Regulating Drug Resistance in Acute and Chronic Myeloid Leukemias. Biomedicines 2022;10:1841. [Crossref] [PubMed]
- Gao M, Kong Y, Yang G, et al. Multiple myeloma cancer stem cells. Oncotarget 2016;7:35466-77. [Crossref] [PubMed]
- Hua Y, Zheng Y, Yao Y, et al. Metformin and cancer hallmarks: shedding new lights on therapeutic repurposing. J Transl Med 2023;21:403. [Crossref] [PubMed]
- Alušík Š, Paluch Z. Metformin: the past, presence, and future. Minerva Med 2015;106:233-8. [PubMed]
- Xiao K, Liu F, Liu J, et al. The effect of metformin on lung cancer risk and survival in patients with type 2 diabetes mellitus: A meta-analysis. J Clin Pharm Ther 2020;45:783-92. [Crossref] [PubMed]
- Brancher S, Støer NC, Weiderpass E, et al. Metformin use and lung cancer survival: a population-based study in Norway. Br J Cancer 2021;124:1018-25. [Crossref] [PubMed]
- Shi B, Hu X, He H, et al. Metformin suppresses breast cancer growth via inhibition of cyclooxygenase-2. Oncol Lett 2021;22:615. [Crossref] [PubMed]
- Cejuela M, Martin-Castillo B, Menendez JA, et al. Metformin and Breast Cancer: Where Are We Now? Int J Mol Sci 2022;23:2705. [Crossref] [PubMed]
- Higurashi T, Nakajima A. Metformin and Colorectal Cancer. Front Endocrinol (Lausanne) 2018;9:622. [Crossref] [PubMed]
- Xiao Q, Xiao J, Liu J, et al. Metformin suppresses the growth of colorectal cancer by targeting INHBA to inhibit TGF-β/PI3K/AKT signaling transduction. Cell Death Dis 2022;13:202. [Crossref] [PubMed]
- Tossetta G. Metformin Improves Ovarian Cancer Sensitivity to Paclitaxel and Platinum-Based Drugs: A Review of In Vitro Findings. Int J Mol Sci 2022;23:12893. [Crossref] [PubMed]
- Ramos-Peñafiel C, Olarte-Carrillo I, Cerón-Maldonado R, et al. Effect of metformin on the survival of patients with ALL who express high levels of the ABCB1 drug resistance gene. J Transl Med 2018;16:245. [Crossref] [PubMed]
- You R, Wang B, Chen P, et al. Metformin sensitizes AML cells to chemotherapy through blocking mitochondrial transfer from stromal cells to AML cells. Cancer Lett 2022;532:215582. [Crossref] [PubMed]
- Van der Vreken A, Oudaert I, Ates G, et al. Metformin confers sensitisation to syrosingopine in multiple myeloma cells by metabolic blockage and inhibition of protein synthesis. J Pathol 2023;260:112-23. [Crossref] [PubMed]
- Werner EA, Bell J. CCXIV.—The preparation of methylguanidine, and of ββ-dimethylguanidine by the interaction of dicyanodiamide, and methylammonium and dimethylammonium chlorides respectively. Journal of the Chemical Society, Transactions 1922;121:1790-4. [Crossref]
- Andrzejewski S, Siegel PM, St-Pierre J. Metabolic Profiles Associated With Metformin Efficacy in Cancer. Front Endocrinol (Lausanne) 2018;9:372. [Crossref] [PubMed]
- Evans JM, Donnelly LA, Emslie-Smith AM, et al. Metformin and reduced risk of cancer in diabetic patients. BMJ 2005;330:1304-5. [Crossref] [PubMed]
- Foretz M, Guigas B, Bertrand L, et al. Metformin: from mechanisms of action to therapies. Cell Metab 2014;20:953-66. [Crossref] [PubMed]
- Saiz-Rodríguez M, Ochoa D, Zubiaur P, et al. Identification of Transporter Polymorphisms Influencing Metformin Pharmacokinetics in Healthy Volunteers. J Pers Med 2023;13:489. [Crossref] [PubMed]
- Zhang Y, Zhou F, Guan J, et al. Action Mechanism of Metformin and Its Application in Hematological Malignancy Treatments: A Review. Biomolecules 2023;13:250. [Crossref] [PubMed]
- Whitaker-Menezes D, Martinez-Outschoorn UE, Flomenberg N, et al. Hyperactivation of oxidative mitochondrial metabolism in epithelial cancer cells in situ: visualizing the therapeutic effects of metformin in tumor tissue. Cell Cycle 2011;10:4047-64. [Crossref] [PubMed]
- Pierotti MA, Berrino F, Gariboldi M, et al. Targeting metabolism for cancer treatment and prevention: metformin, an old drug with multi-faceted effects. Oncogene 2013;32:1475-87. [Crossref] [PubMed]
- Vial G, Detaille D, Guigas B. Role of Mitochondria in the Mechanism(s) of Action of Metformin. Front Endocrinol (Lausanne) 2019;10:294. [Crossref] [PubMed]
- Bridges HR, Jones AJ, Pollak MN, et al. Effects of metformin and other biguanides on oxidative phosphorylation in mitochondria. Biochem J 2014;462:475-87. [Crossref] [PubMed]
- Ma T, Tian X, Zhang B, et al. Low-dose metformin targets the lysosomal AMPK pathway through PEN2. Nature 2022;603:159-65. [Crossref] [PubMed]
- Zhang CS, Li M, Ma T, et al. Metformin Activates AMPK through the Lysosomal Pathway. Cell Metab 2016;24:521-2. [Crossref] [PubMed]
- Xu J, Ji J, Yan XH. Cross-talk between AMPK and Mtor in regulating energy balance. Crit Rev Food Sci Nutr 2012;52:373-81. [Crossref] [PubMed]
- Zachariah Tom R, Garcia-Roves PM, Sjögren RJ, et al. Effects of AMPK activation on insulin sensitivity and metabolism in leptin-deficient ob/ob mice. Diabetes 2014;63:1560-71. [Crossref] [PubMed]
- Pernicova I, Korbonits M. Metformin–mode of action and clinical implications for diabetes and cancer. Nat Rev Endocrinol 2014;10:143-56. [Crossref] [PubMed]
- Yu X, Mao W, Zhai Y, et al. Anti-tumor activity of metformin: from metabolic and epigenetic perspectives. Oncotarget 2017;8:5619-28. [Crossref] [PubMed]
- Hardie DG, Ross FA, Hawley SA. AMPK: a nutrient and energy sensor that maintains energy homeostasis. Nat Rev Mol Cell Biol 2012;13:251-62. [Crossref] [PubMed]
- Loubière C, Goiran T, Laurent K, et al. Metformin-induced energy deficiency leads to the inhibition of lipogenesis in prostate cancer cells. Oncotarget 2015;6:15652-61. [Crossref] [PubMed]
- Janzer A, German NJ, Gonzalez-Herrera KN, et al. Metformin and phenformin deplete tricarboxylic acid cycle and glycolytic intermediates during cell transformation and NTPs in cancer stem cells. Proc Natl Acad Sci U S A 2014;111:10574-9. [Crossref] [PubMed]
- Marquez J, Flores J, Kim AH, et al. Rescue of TCA Cycle Dysfunction for Cancer Therapy. J Clin Med 2019;8:2161. [Crossref] [PubMed]
- Madiraju AK, Erion DM, Rahimi Y, et al. Metformin suppresses gluconeogenesis by inhibiting mitochondrial glycerophosphate dehydrogenase. Nature 2014;510:542-6. [Crossref] [PubMed]
- Mráček T, Drahota Z, Houštěk J. The function and the role of the mitochondrial glycerol-3-phosphate dehydrogenase in mammalian tissues. Biochim Biophys Acta 2013;1827:401-10. [Crossref] [PubMed]
- Foretz M, Guigas B, Viollet B. Metformin: update on mechanisms of action and repurposing potential. Nat Rev Endocrinol 2023;19:460-76. [Crossref] [PubMed]
- Song CW, Lee H, Dings RP, et al. Metformin kills and radiosensitizes cancer cells and preferentially kills cancer stem cells. Sci Rep 2012;2:362. [Crossref] [PubMed]
- Courtois S, Durán RV, Giraud J, et al. Metformin targets gastric cancer stem cells. Eur J Cancer 2017;84:193-201. [Crossref] [PubMed]
- Shi P, Liu W. Metformin suppresses triple-negative breast cancer stem cells by targeting KLF5 for degradation. Cell Discov 2017;3:17010. [Crossref] [PubMed]
- Urpilainen E, Puistola U, Boussios S, et al. Metformin and ovarian cancer: the evidence. Ann Transl Med 2020;8:1711. [Crossref] [PubMed]
- Kilmister EJ, Koh SP, Weth FR, et al. Cancer Metastasis and Treatment Resistance: Mechanistic Insights and Therapeutic Targeting of Cancer Stem Cells and the Tumor Microenvironment. Biomedicines 2022;10:2988. [Crossref] [PubMed]
- Zarei E, Sefidi-Heris Y, Saadat I. Synergistic effects of metformin and curcumin on cytotoxicity of chemotherapy drugs using a gastric cancer cell line model. EXCLI J 2021;20:1488-98. [PubMed]
- Lee DE, Lee GY, Lee HM, et al. Synergistic apoptosis by combination of metformin and an O-GlcNAcylation inhibitor in colon cancer cells. Cancer Cell Int 2023;23:108. [Crossref] [PubMed]
- Kao HW, Tsai KW, Lin WC. Synergistic Effect of Metformin and Lansoprazole Against Gastric Cancer through Growth Inhibition. Int J Med Sci 2023;20:717-24. [Crossref] [PubMed]
- Sorokin D, Shchegolev Y, Scherbakov A, et al. Metformin Restores the Drug Sensitivity of MCF-7 Cells Resistant Derivates via the Cooperative Modulation of Growth and Apoptotic-Related Pathways. Pharmaceuticals (Basel) 2020;13:206. [Crossref] [PubMed]
- Li L, Wang Y, Peng T, et al. Metformin restores crizotinib sensitivity in crizotinib-resistant human lung cancer cells through inhibition of IGF1-R signaling pathway. Oncotarget 2016;7:34442-52. [Crossref] [PubMed]
- Di Marco M, Ramassone A, Pagotto S, et al. MicroRNAs in Autoimmunity and Hematological Malignancies. Int J Mol Sci 2018;19:3139. [Crossref] [PubMed]
- Ghafouri-Fard S, Esmaeili M, Taheri M. Expression of non-coding RNAs in hematological malignancies. Eur J Pharmacol 2020;875:172976. [Crossref] [PubMed]
- Housman G, Byler S, Heerboth S, et al. Drug resistance in cancer: an overview. Cancers (Basel) 2014;6:1769-92. [Crossref] [PubMed]
- Vasconcelos MH, Caires HR, Ābols A, et al. Extracellular vesicles as a novel source of biomarkers in liquid biopsies for monitoring cancer progression and drug resistance. Drug Resist Updat 2019;47:100647. [Crossref] [PubMed]
- Vasconcelos MH. Special Issue: New Approaches to Counteract Drug Resistance in Cancer. Molecules 2016;22:6. [Crossref] [PubMed]
- Xavier CP, Pesic M, Vasconcelos MH. Understanding Cancer Drug Resistance by Developing and Studying Resistant Cell Line Models. Curr Cancer Drug Targets 2016;16:226-37. [Crossref] [PubMed]
- Assaraf YG, Brozovic A, Gonçalves AC, et al. The multi-factorial nature of clinical multidrug resistance in cancer. Drug Resist Updat 2019;46:100645. [Crossref] [PubMed]
- Kim DH, Sriharsha L, Xu W, et al. Clinical relevance of a pharmacogenetic approach using multiple candidate genes to predict response and resistance to imatinib therapy in chronic myeloid leukemia. Clin Cancer Res 2009;15:4750-8. [Crossref] [PubMed]
- Pérez-Ramírez C, Cañadas-Garre M, Molina MÁ, et al. Contribution of genetic factors to platinum-based chemotherapy sensitivity and prognosis of non-small cell lung cancer. Mutat Res Rev Mutat Res 2017;771:32-58. [Crossref] [PubMed]
- Abbas R, Boni J, Sonnichsen D. Effect of rifampin on the pharmacokinetics of bosutinib, a dual Src/Abl tyrosine kinase inhibitor, when administered concomitantly to healthy subjects. Drug Metab Pers Ther 2015;30:57-63. [Crossref] [PubMed]
- Hussaarts KGAM, Veerman GDM, Jansman FGA, et al. Clinically relevant drug interactions with multikinase inhibitors: a review. Ther Adv Med Oncol 2019;11:1758835918818347. [Crossref] [PubMed]
- Mansoori B, Mohammadi A, Davudian S, et al. The Different Mechanisms of Cancer Drug Resistance: A Brief Review. Adv Pharm Bull 2017;7:339-48. [Crossref] [PubMed]
- Jones D, Kamel-Reid S, Bahler D, et al. Laboratory practice guidelines for detecting and reporting BCR-ABL drug resistance mutations in chronic myelogenous leukemia and acute lymphoblastic leukemia: a report of the Association for Molecular Pathology. J Mol Diagn 2009;11:4-11. [Crossref] [PubMed]
- Yaghmaie M, Yeung CC. Molecular Mechanisms of Resistance to Tyrosine Kinase Inhibitors. Curr Hematol Malig Rep 2019;14:395-404. [Crossref] [PubMed]
- He Z, Hu B, Tang L, et al. The overexpression of MRP4 is related to multidrug resistance in osteosarcoma cells. J Cancer Res Ther 2015;11:18-23. [Crossref] [PubMed]
- Krisnamurti DG, Louisa M, Anggraeni E, et al. Drug Efflux Transporters Are Overexpressed in Short-Term Tamoxifen-Induced MCF7 Breast Cancer Cells. Adv Pharmacol Sci 2016;2016:6702424. [Crossref] [PubMed]
- Rinaldetti S, Pfirrmann M, Manz K, et al. Effect of ABCG2, OCT1, and ABCB1 (MDR1) Gene Expression on Treatment-Free Remission in a EURO-SKI Subtrial. Clin Lymphoma Myeloma Leuk 2018;18:266-71. [Crossref] [PubMed]
- Robey RW, Pluchino KM, Hall MD, et al. Revisiting the role of ABC transporters in multidrug-resistant cancer. Nat Rev Cancer 2018;18:452-64. [Crossref] [PubMed]
- Tan SF, Dunton W, Liu X, et al. Acid ceramidase promotes drug resistance in acute myeloid leukemia through NF-Kb-dependent P-glycoprotein upregulation. J Lipid Res 2019;60:1078-86. [Crossref] [PubMed]
- Goler-Baron V, Assaraf YG. Structure and function of ABCG2-rich extracellular vesicles mediating multidrug resistance. PLoS One 2011;6:e16007. [Crossref] [PubMed]
- Gong J, Luk F, Jaiswal R, et al. Microparticle drug sequestration provides a parallel pathway in the acquisition of cancer drug resistance. Eur J Pharmacol 2013;721:116-25. [Crossref] [PubMed]
- Indran IR, Tufo G, Pervaiz S, et al. Recent advances in apoptosis, mitochondria and drug resistance in cancer cells. Biochim Biophys Acta 2011;1807:735-45. [Crossref] [PubMed]
- Ferreira BI, Lie MK, Engelsen AST, et al. Adaptive mechanisms of resistance to anti-neoplastic agents. Medchemcomm 2016;8:53-66. [Crossref] [PubMed]
- Kim EK, Jang M, Song MJ, et al. Redox-Mediated Mechanism of Chemoresistance in Cancer Cells. Antioxidants (Basel) 2019;8:471. [Crossref] [PubMed]
- Cucè M, Gallo Cantafio ME, Siciliano MA, et al. Trabectedin triggers direct and NK-mediated cytotoxicity in multiple myeloma. J Hematol Oncol 2019;12:32. [Crossref] [PubMed]
- Sakthivel KM, Hariharan S. Regulatory players of DNA damage repair mechanisms: Role in Cancer Chemoresistance. Biomed Pharmacother 2017;93:1238-45. [Crossref] [PubMed]
- Zeller C, Dai W, Steele NL, et al. Candidate DNA methylation drivers of acquired cisplatin resistance in ovarian cancer identified by methylome and expression profiling. Oncogene 2012;31:4567-76. [Crossref] [PubMed]
- Shah K, Rawal RM. Genetic and Epigenetic Modulation of Drug Resistance in Cancer: Challenges and Opportunities. Curr Drug Metab 2019;20:1114-31. [Crossref] [PubMed]
- Lavi O, Greene JM, Levy D, et al. The role of cell density and intratumoral heterogeneity in multidrug resistance. Cancer Res 2013;73:7168-75. [Crossref] [PubMed]
- Phi LTH, Sari IN, Yang YG, et al. Cancer Stem Cells (CSCs) in Drug Resistance and their Therapeutic Implications in Cancer Treatment. Stem Cells Int 2018;2018:5416923. [Crossref] [PubMed]
- Wu T, Dai Y. Tumor microenvironment and therapeutic response. Cancer Lett 2017;387:61-8. [Crossref] [PubMed]
- Chennamadhavuni A, Lyengar V, Mukkamalla SKR, et al. Leukemia. Treasure Island, FL, USA: StatPearls Publishing; 2023.
- Jabbour E, Kantarjian H. Chronic myeloid leukemia: 2020 update on diagnosis, therapy and monitoring. Am J Hematol 2020;95:691-709. [Crossref] [PubMed]
- Nicholson E, Holyoake T. The chronic myeloid leukemia stem cell. Clin Lymphoma Myeloma 2009;9:S376-81. [Crossref] [PubMed]
- Frazer R, Irvine AE, McMullin MF. Chronic Myeloid Leukaemia in The 21st Century. Ulster Med J 2007;76:8-17. [PubMed]
- Jabbour E, Kantarjian H. Chronic myeloid leukemia: 2018 update on diagnosis, therapy and monitoring. Am J Hematol 2018;93:442-59. [Crossref] [PubMed]
- Hoffmann VS, Baccarani M, Hasford J, et al. The EUTOS population-based registry: incidence and clinical characteristics of 2904 CML patients in 20 European Countries. Leukemia 2015;29:1336-43. [Crossref] [PubMed]
- Rinaldi I, Winston K. Chronic Myeloid Leukemia, from Pathophysiology to Treatment-Free Remission: A Narrative Literature Review. J Blood Med 2023;14:261-77. [Crossref] [PubMed]
- Hochhaus A, Baccarani M, Silver RT, et al. European LeukemiaNet 2020 recommendations for treating chronic myeloid leukemia. Leukemia 2020;34:966-84. [Crossref] [PubMed]
- Breccia M, Alimena G. Second-Generation Tyrosine Kinase Inhibitors (Tki) as Salvage Therapy for Resistant or Intolerant Patients to Prior TKIs. Mediterr J Hematol Infect Dis 2014;6:e2014003. [Crossref] [PubMed]
- O’Hare T, Shakespeare WC, Zhu X, et al. AP24534, a pan-BCR-ABL inhibitor for chronic myeloid leukemia, potently inhibits the T315I mutant and overcomes mutation-based resistance. Cancer Cell 2009;16:401-12. [Crossref] [PubMed]
- Zhou T, Commodore L, Huang WS, et al. Structural mechanism of the Pan-BCR-ABL inhibitor ponatinib (AP24534): lessons for overcoming kinase inhibitor resistance. Chem Biol Drug Des 2011;77:1-11. [Crossref] [PubMed]
- Cortes JE, Kim DW, Pinilla-Ibarz J, et al. A phase 2 trial of ponatinib in Philadelphia chromosome-positive leukemias. N Engl J Med 2013;369:1783-96. [Crossref] [PubMed]
- Massaro F, Molica M, Breccia M. Ponatinib: A Review of Efficacy and Safety. Curr Cancer Drug Targets 2018;18:847-56. [Crossref] [PubMed]
- Marin D, Kaeda J, Szydlo R, et al. Monitoring patients in complete cytogenetic remission after treatment of CML in chronic phase with imatinib: patterns of residual leukaemia and prognostic factors for cytogenetic relapse. Leukemia 2005;19:507-12. [Crossref] [PubMed]
- Pokorny R, Stenehjem DD, Gilreath JA. Impact of metformin on tyrosine kinase inhibitor response in chronic myeloid leukemia. J Oncol Pharm Pract 2022;28:916-23. [Crossref] [PubMed]
- Vakana E, Altman JK, Glaser H, et al. Antileukemic effects of AMPK activators on BCR-ABL-expressing cells. Blood 2011;118:6399-402. [Crossref] [PubMed]
- Shi R, Lin J, Gong Y, et al. The antileukemia effect of metformin in the Philadelphia chromosome-positive leukemia cell line and patient primary leukemia cell. Anticancer Drugs 2015;26:913-22. [Crossref] [PubMed]
- Curvello R, Neto MDS, Ramos SP, et al. Metformin Promotes Cancer Cells Death, Inhibits PGP Expression and Sensitize MDR Leukemic Cells to the Effects of Imatinib Mesylate. Ann Oncol 2013;24:i23-4. [Crossref]
- Glamoclija U, Mahmutovic L, Bilajac E, et al. Metformin and Thymoquinone Synergistically Inhibit Proliferation of Imatinib-Resistant Human Leukemic Cells. Front Pharmacol 2022;13:867133. [Crossref] [PubMed]
- Na YJ, Yu ES, Kim DS, et al. Metformin enhances the cytotoxic effect of nilotinib and overcomes nilotinib resistance in chronic myeloid leukemia cells. Korean J Intern Med 2021;36:S196-206. [Crossref] [PubMed]
- Yi Y, Gao L, Wu M, et al. Metformin Sensitizes Leukemia Cells to Vincristine via Activation of AMP-activated Protein Kinase. J Cancer 2017;8:2636-42. [Crossref] [PubMed]
- Adekola KU, Dalva Aydemir S, Ma S, et al. Investigating and targeting chronic lymphocytic leukemia metabolism with the human immunodeficiency virus protease inhibitor ritonavir and metformin. Leuk Lymphoma 2015;56:450-9. [Crossref] [PubMed]
- Bruno S, Ledda B, Tenca C, et al. Metformin inhibits cell cycle progression of B-cell chronic lymphocytic leukemia cells. Oncotarget 2015;6:22624-40. [Crossref] [PubMed]
- Callaghan R, Luk F, Bebawy M. Inhibition of the multidrug resistance P-glycoprotein: time for a change of strategy? Drug Metab Dispos 2014;42:623-31. [Crossref] [PubMed]
- Kim DS, Na YJ, Kang MH, et al. Use of deferasirox, an iron chelator, to overcome imatinib resistance of chronic myeloid leukemia cells. Korean J Intern Med 2016;31:357-66. [Crossref] [PubMed]
- Ding J, Romani J, Zaborski M, et al. Inhibition of PI3K/Mtor overcomes nilotinib resistance in BCR-ABL1 positive leukemia cells through translational down-regulation of MDM2. PLoS One 2013;8:e83510. [Crossref] [PubMed]
- Houshmand M, Simonetti G, Circosta P, et al. Chronic myeloid leukemia stem cells. Leukemia 2019;33:1543-56. [Crossref] [PubMed]
- Chereda B, Melo JV. Natural course and biology of CML. Ann Hematol 2015;94:S107-21. [Crossref] [PubMed]
- Shin MG, Eom HY, Kim HR, et al. Metformin Selectively Suppressed the Proliferation of AML Stem Cells through Increased Phosphorylation of AMP-Activated Protein Kinase. Blood 2010;116:4771. [Crossref]
- Vakiti A, Mewawalla P. Acute Myeloid Leukemia. Treasure Island, FL, USA: StatPearls Publishing; 2023.
- Zhang J, Gu Y, Chen B. Mechanisms of drug resistance in acute myeloid leukemia. Onco Targets Ther 2019;12:1937-45. [Crossref] [PubMed]
- Megías-Vericat JE, Rojas L, Herrero MJ, et al. Influence of ABCB1 polymorphisms upon the effectiveness of standard treatment for acute myeloid leukemia: a systematic review and meta-analysis of observational studies. Pharmacogenomics J 2015;15:109-18. [Crossref] [PubMed]
- Luppi M, Fabbiano F, Visani G, et al. Novel Agents for Acute Myeloid Leukemia. Cancers (Basel) 2018;10:429. [Crossref] [PubMed]
- Wang F, Liu Z, Zeng J, et al. Metformin synergistically sensitizes FLT3-ITD-positive acute myeloid leukemia to sorafenib by promoting Mtor-mediated apoptosis and autophagy. Leuk Res 2015;39:1421-7. [Crossref] [PubMed]
- Yuan F, Cheng C, Xiao F, et al. Inhibition of Mtorc1/P70S6K pathway by Metformin synergistically sensitizes Acute Myeloid Leukemia to Ara-C. Life Sci 2020;243:117276. [Crossref] [PubMed]
- Rodríguez-Lirio A, Pérez-Yarza G, Fernández-Suárez MR, et al. Metformin Induces Cell Cycle Arrest and Apoptosis in Drug-Resistant Leukemia Cells. Leuk Res Treatment 2015;2015:516460. [Crossref] [PubMed]
- Rahmani M, Davis EM, Bauer C, et al. Apoptosis induced by the kinase inhibitor BAY 43-9006 in human leukemia cells involves down-regulation of Mcl-1 through inhibition of translation. J Biol Chem 2005;280:35217-27. [Crossref] [PubMed]
- Levin M, Stark M, Berman B, et al. Surmounting Cytarabine-resistance in acute myeloblastic leukemia cells and specimens with a synergistic combination of hydroxyurea and azidothymidine. Cell Death Dis 2019;10:390. [Crossref] [PubMed]
- Crane GM, Jeffery E, Morrison SJ. Adult haematopoietic stem cell niches. Nat Rev Immunol 2017;17:573-90. [Crossref] [PubMed]
- Schepers K, Campbell TB, Passegué E. Normal and leukemic stem cell niches: insights and therapeutic opportunities. Cell Stem Cell 2015;16:254-67. [Crossref] [PubMed]
- Mistry JJ, Marlein CR, Moore JA, et al. ROS-mediated PI3K activation drives mitochondrial transfer from stromal cells to hematopoietic stem cells in response to infection. Proc Natl Acad Sci U S A 2019;116:24610-9. [Crossref] [PubMed]
- Marlein CR, Zaitseva L, Piddock RE, et al. NADPH oxidase-2 derived superoxide drives mitochondrial transfer from bone marrow stromal cells to leukemic blasts. Blood 2017;130:1649-60. [Crossref] [PubMed]
- Sahinbegovic H, Jelinek T, Hrdinka M, et al. Intercellular Mitochondrial Transfer in the Tumor Microenvironment. Cancers (Basel) 2020;12:1787. [Crossref] [PubMed]
- Leslie EM, Deeley RG, Cole SP. Multidrug resistance proteins: role of P-glycoprotein, MRP1, MRP2, and BCRP (ABCG2) in tissue defense. Toxicol Appl Pharmacol 2005;204:216-37. [Crossref] [PubMed]
- Kim HG, Hien TT, Han EH, et al. Metformin inhibits P-glycoprotein expression via the NF-Kb pathway and CRE transcriptional activity through AMPK activation. Br J Pharmacol 2011;162:1096-108. [Crossref] [PubMed]
- Xue C, Wang C, Liu Q, et al. Targeting P-glycoprotein expression and cancer cell energy metabolism: combination of metformin and 2-deoxyglucose reverses the multidrug resistance of K562/Dox cells to doxorubicin. Tumour Biol 2016;37:8587-97. [Crossref] [PubMed]
- Ling S, Tian Y, Zhang H, et al. Metformin reverses multidrug resistance in human hepatocellular carcinoma Bel‑7402/5‑fluorouracil cells. Mol Med Rep 2014;10:2891-7. Erratum in: Mol Med Rep 2022;25:175. [Crossref] [PubMed]
- Qiang P, Shao Y, Sun YP, et al. Metformin inhibits proliferation and migration of endometrial cancer cells through regulating PI3K/AKT/MDM2 pathway. Eur Rev Med Pharmacol Sci 2019;23:1778-85. [PubMed]
- Jongen-Lavrencic M, Grob T, Hanekamp D, et al. Molecular Minimal Residual Disease in Acute Myeloid Leukemia. N Engl J Med 2018;378:1189-99. [Crossref] [PubMed]
- Yao Y, Lin X, Li F, et al. The global burden and attributable risk factors of chronic lymphocytic leukemia in 204 countries and territories from 1990 to 2019: analysis based on the global burden of disease study 2019. Biomed Eng Online 2022;21:4. [Crossref] [PubMed]
- Siegel RL, Miller KD, Goding Sauer A, et al. Colorectal cancer statistics, 2020. CA Cancer J Clin 2020;70:145-64. [Crossref] [PubMed]
- Stauder R, Eichhorst B, Hamaker ME, et al. Management of chronic lymphocytic leukemia (CLL) in the elderly: a position paper from an international Society of Geriatric Oncology (SIOG) Task Force. Ann Oncol 2017;28:218-27. [Crossref] [PubMed]
- Hallek M. Chronic lymphocytic leukemia: 2017 update on diagnosis, risk stratification, and treatment. Am J Hematol 2017;92:946-65. [Crossref] [PubMed]
- Kipps TJ, Stevenson FK, Wu CJ, et al. Chronic lymphocytic leukaemia. Nat Rev Dis Primers 2017;3:16096. [Crossref] [PubMed]
- Roberts AW, Ma S, Kipps TJ, et al. Efficacy of venetoclax in relapsed chronic lymphocytic leukemia is influenced by disease and response variables. Blood 2019;134:111-22. [Crossref] [PubMed]
- Xu W, Yang S, Zhou K, et al. Treatment of relapsed/refractory chronic lymphocytic leukemia/small lymphocytic lymphoma with the BTK inhibitor zanubrutinib: phase 2, single-arm, multicenter study. J Hematol Oncol 2020;13:48. [Crossref] [PubMed]
- Woyach JA, Ruppert AS, Heerema NA, et al. Ibrutinib Regimens versus Chemoimmunotherapy in Older Patients with Untreated CLL. N Engl J Med 2018;379:2517-28. [Crossref] [PubMed]
- Jain N, Keating M, Thompson P, et al. Ibrutinib and Venetoclax for First-Line Treatment of CLL. N Engl J Med 2019;380:2095-103. [Crossref] [PubMed]
- Fatima N, Crassini KR, Thurgood L, et al. Therapeutic approaches and drug-resistance in chronic lymphocytic leukaemia. Cancer Drug Resist 2020;3:532-49. [Crossref] [PubMed]
- Condoluci A, Rossi D. SOHO State of the Art Updates and Next Questions: Clonal Evolution in Chronic Lymphocytic Leukemia. Clin Lymphoma Myeloma Leuk 2020;20:779-84. [Crossref] [PubMed]
- Malard F, Mohty M. Acute lymphoblastic leukaemia. Lancet 2020;395:1146-62. [Crossref] [PubMed]
- Siegel RL, Miller KD, Jemal A. Cancer statistics, 2018. CA Cancer J Clin 2018;68:7-30. [Crossref] [PubMed]
- Pulte D, Gondos A, Brenner H. Improvement in survival in younger patients with acute lymphoblastic leukemia from the 1980s to the early 21st century. Blood 2009;113:1408-11. [Crossref] [PubMed]
- Pulte D, Jansen L, Gondos A, et al. Survival of adults with acute lymphoblastic leukemia in Germany and the United States. PLoS One 2014;9:e85554. [Crossref] [PubMed]
- Hunger SP, Lu X, Devidas M, et al. Improved survival for children and adolescents with acute lymphoblastic leukemia between 1990 and 2005: a report from the children’s oncology group. J Clin Oncol 2012;30:1663-9. [Crossref] [PubMed]
- Urruticoechea A, Alemany R, Balart J, et al. Recent advances in cancer therapy: an overview. Curr Pharm Des 2010;16:3-10. [Crossref] [PubMed]
- Wong AH, Deng CX. Precision Medicine for Personalized Cancer Therapy. Int J Biol Sci 2015;11:1410-2. [Crossref] [PubMed]
- Coustan-Smith E, Kitanaka A, Pui CH, et al. Clinical relevance of BCL-2 overexpression in childhood acute lymphoblastic leukemia. Blood 1996;87:1140-6. [Crossref] [PubMed]
- Su Y, Zhang X, Sinko PJ. Exploitation of drug-induced Bcl-2 overexpression for restoring normal apoptosis function: a promising new approach to the treatment of multidrug resistant cancer. Cancer Lett 2007;253:115-23. [Crossref] [PubMed]
- Moon DO, Kim MO, Choi YH, et al. Bcl-2 overexpression attenuates SP600125-induced apoptosis in human leukemia U937 cells. Cancer Lett 2008;264:316-25. [Crossref] [PubMed]
- Jin CY, Park C, Lee JH, et al. Naringenin-induced apoptosis is attenuated by Bcl-2 but restored by the small molecule Bcl-2 inhibitor, HA 14-1, in human leukemia U937 cells. Toxicol In Vitro 2009;23:259-65. [Crossref] [PubMed]
- Kong CZ, Zhang Z. Bcl-2 overexpression inhibits generation of intracellular reactive oxygen species and blocks andomized-induced apoptosis in bladder cancer cells. Asian Pac J Cancer Prev 2013;14:895-901. [Crossref] [PubMed]
- Linton KJ. Structure and function of ABC transporters. Physiology (Bethesda) 2007;22:122-30. [Crossref] [PubMed]
- El-Awady R, Saleh E, Hashim A, et al. The Role of Eukaryotic and Prokaryotic ABC Transporter Family in Failure of Chemotherapy. Front Pharmacol 2017;7:535. [PubMed]
- Chen Z, Shi T, Zhang L, et al. Mammalian drug efflux transporters of the ATP binding cassette (ABC) family in multidrug resistance: A review of the past decade. Cancer Lett 2016;370:153-64. [Crossref] [PubMed]
- Trucco M, Barredo JC, Goldberg J, et al. A phase I window, dose escalating and safety trial of metformin in combination with induction chemotherapy in relapsed refractory acute lymphoblastic leukemia: Metformin with induction chemotherapy of vincristine, dexamethasone, PEG-asparaginase, and doxorubicin. Pediatr Blood Cancer 2018;65:e27224. [Crossref] [PubMed]
- Jamil A, Mukkamalla SKR. Lymphoma. Treasure Island, FL, USA: StatPearls Publishing; 2023.
- Mugnaini EN, Ghosh N. Lymphoma. Prim Care 2016;43:661-75. [Crossref] [PubMed]
- Pérez-Galán P, Dreyling M, Wiestner A. Mantle cell lymphoma: biology, pathogenesis, and the molecular basis of treatment in the genomic era. Blood 2011;117:26-38. [Crossref] [PubMed]
- Yu BH, Zhou XY, Xiao XY, et al. Activation and clinicopathologic significance of AKT/Mtor signaling pathway in diffuse large B-cell lymphoma. Zhonghua Bing Li Xue Za Zhi 2009;38:35-41. [PubMed]
- Leseux L, Hamdi SM, Al Saati T, et al. Syk-dependent Mtor activation in follicular lymphoma cells. Blood 2006;108:4156-62. [Crossref] [PubMed]
- Vega F, Medeiros LJ, Leventaki V, et al. Activation of mammalian target of rapamycin signaling pathway contributes to tumor cell survival in anaplastic lymphoma kinase-positive anaplastic large cell lymphoma. Cancer Res 2006;66:6589-97. [Crossref] [PubMed]
- Witzig TE, Gupta M. Signal transduction inhibitor therapy for lymphoma. Hematology Am Soc Hematol Educ Program 2010;2010:265-70. [Crossref] [PubMed]
- Shi WY, Xiao D, Wang L, et al. Therapeutic metformin/AMPK activation blocked lymphoma cell growth via inhibition of Mtor pathway and induction of autophagy. Cell Death Dis 2012;3:e275. [Crossref] [PubMed]
- Fan X, Zhong HJ, Zhao BB, et al. Metformin prolonged the survival of diffuse large B-cell lymphoma and grade 3b follicular lymphoma patients responding to first-line treatment with rituximab plus cyclophosphamide, doxorubicin, vincristine, and prednisone: a prospective phase II clinical trial. Transl Cancer Res 2018;7:1044-53. [Crossref]
- Singh AR, Gu JJ, Zhang Q, et al. Metformin sensitizes therapeutic agents and improves outcome in pre-clinical and clinical diffuse large B-cell lymphoma. Cancer Metab 2020;8:10. [Crossref] [PubMed]
- Bagaloni I, Visani A, Biagiotti S, et al. Metabolic Switch and Cytotoxic Effect of Metformin on Burkitt Lymphoma. Front Oncol 2021;11:661102. [Crossref] [PubMed]
- Coiffier B, Thieblemont C, Van Den Neste E, et al. Long-term outcome of patients in the LNH-98.5 trial, the first randomized study comparing rituximab-CHOP to standard CHOP chemotherapy in DLBCL patients: a study by the Groupe d’Etudes des Lymphomes de l’Adulte. Blood 2010;116:2040-5. [Crossref] [PubMed]
- Delarue R, Tilly H, Mounier N, et al. Dose-dense rituximab-CHOP compared with standard rituximab-CHOP in elderly patients with diffuse large B-cell lymphoma (the LNH03-6B study): a andomized phase 3 trial. Lancet Oncol 2013;14:525-33. [Crossref] [PubMed]
- Casulo C, Friedberg JW. Burkitt lymphoma- a rare but challenging lymphoma. Best Pract Res Clin Haematol 2018;31:279-84. [Crossref] [PubMed]
- Ganapathy-Kanniappan S, Geschwind JF. Tumor glycolysis as a target for cancer therapy: progress and prospects. Mol Cancer 2013;12:152. [Crossref] [PubMed]
- Marcucci F, Rumio C. Glycolysis-induced drug resistance in tumors-A response to danger signals? Neoplasia 2021;23:234-45. [Crossref] [PubMed]
- Padala SA, Barsouk A, Barsouk A, et al. Epidemiology, Staging, and Management of Multiple Myeloma. Med Sci (Basel) 2021;9:3. [Crossref] [PubMed]
- Guedes A, Becker RG, Teixeira LEM. Multiple Myeloma (Part 1) – Update on Epidemiology, Diagnostic Criteria, Systemic Treatment and Prognosis. Rev Bras Ortop (Sao Paulo) 2023;58:361-7. [Crossref] [PubMed]
- Rajkumar SV, Kumar S. Multiple Myeloma: Diagnosis and Treatment. Mayo Clin Proc 2016;91:101-19. [Crossref] [PubMed]
- Moreau P, Attal M, Facon T. Frontline therapy of multiple myeloma. Blood 2015;125:3076-84. [Crossref] [PubMed]
- Solimando AG, Krebs M, Bittrich M, et al. The Urgent Need for Precision Medicine in Cancer and Its Microenvironment: The Paradigmatic Case of Multiple Myeloma. J Clin Med 2022;11:5461. [Crossref] [PubMed]
- McBrayer SK, Cheng JC, Singhal S, et al. Multiple myeloma exhibits novel dependence on GLUT4, GLUT8, and GLUT11: implications for glucose transporter-directed therapy. Blood 2012;119:4686-97. [Crossref] [PubMed]
- Bredella MA, Steinbach L, Caputo G, et al. Value of FDG PET in the assessment of patients with multiple myeloma. AJR Am J Roentgenol 2005;184:1199-204. [Crossref] [PubMed]
- Murata H, Hruz PW, Mueckler M. The mechanism of insulin resistance caused by HIV protease inhibitor therapy. J Biol Chem 2000;275:20251-4. [Crossref] [PubMed]
- Vyas AK, Koster JC, Tzekov A, et al. Effects of the HIV protease inhibitor ritonavir on GLUT4 knock-out mice. J Biol Chem 2010;285:36395-400. [Crossref] [PubMed]
- Hertel J, Struthers H, Horj CB, et al. A structural basis for the acute effects of HIV protease inhibitors on GLUT4 intrinsic activity. J Biol Chem 2004;279:55147-52. [Crossref] [PubMed]
- Dalva-Aydemir S, Bajpai R, Martinez M, et al. Targeting the metabolic plasticity of multiple myeloma with FDA-approved ritonavir and metformin. Clin Cancer Res 2015;21:1161-71. [Crossref] [PubMed]
- Zhao Y, Zhang E, Lv N, et al. Metformin and FTY720 Synergistically Induce Apoptosis in Multiple Myeloma Cells. Cell Physiol Biochem 2018;48:785-800. [Crossref] [PubMed]
- Wang Y, Xu W, Yan Z, et al. Metformin induces autophagy and G0/G1 phase cell cycle arrest in myeloma by targeting the AMPK/Mtorc1 and Mtorc2 pathways. J Exp Clin Cancer Res 2018;37:63. [Crossref] [PubMed]
- Jagannathan S, Abdel-Malek MA, Malek E, et al. Pharmacologic screens reveal metformin that suppresses GRP78-dependent autophagy to enhance the anti-myeloma effect of bortezomib. Leukemia 2015;29:2184-91. [Crossref] [PubMed]
- Song Y, Chen S, Xiang W, et al. The mechanism of treatment of multiple myeloma with metformin by way of metabolism. Arch Med Sci 2020;17:1056-63. [Crossref] [PubMed]
- Koomen DC, Meads MB, Magaletti DM, et al. Metabolic Changes Are Associated with Melphalan Resistance in Multiple Myeloma. J Proteome Res 2021;20:3134-49. [Crossref] [PubMed]
- Samur MK, Roncador M, Aktas Samur A, et al. High-dose melphalan treatment significantly increases mutational burden at relapse in multiple myeloma. Blood 2023;141:1724-36. [Crossref] [PubMed]
- Guo W, Wang H, Chen P, et al. Identification and Characterization of Multiple Myeloma Stem Cell-Like Cells. Cancers (Basel) 2021;13:3523. [Crossref] [PubMed]
- Kim JH, Lee KJ, Seo Y, et al. Effects of metformin on colorectal cancer stem cells depend on alterations in glutamine metabolism. Sci Rep 2018;8:409. [Crossref] [PubMed]
- Hirsch HA, Iliopoulos D, Tsichlis PN, et al. Metformin selectively targets cancer stem cells, and acts together with chemotherapy to block tumor growth and prolong remission. Cancer Res 2009;69:7507-11. [Crossref] [PubMed]