From simulation to surgery, advancements and challenges in robotic training for radical prostatectomy: a narrative review
Introduction
Background
The notion of advanced skills training originated not within the medical field but in aviation, notably with the introduction of the simulation approach as a crucial component in pilot training from the 1930s and during most of the 20th century (1). Over time, simulations have evolved to a point where pilots undergo extensive training hours in simulators, mastering takeoffs and landings before engaging with a real aircraft (1). Recognizing its potential application, aviation training practices have been considered for adoption in medicine, particularly for teams in operating theaters, intensive care units, and emergency rooms (2).
Several studies have highlighted the connection between absence in both technical and non-technical skills and complications, leading to unfavorable clinical outcomes and subsequently increased costs for the healthcare system (3,4). For instance, Birkmeyer et al. assessed the relationship between the technical skill of 20 Michigan Bariatric Surgery Collaborative (MBSC) surgeons and postoperative complications in 10,343 patients undergoing laparoscopic gastric bypass (5). Using the validated Objective Structured Assessment of Technical Skills (OSATS) score, the authors categorized surgeons into four groups based on their technical skills. Results indicated that surgeons in the least skilled group exhibited a higher risk-adjusted complications rate, ranging from wound infections to mortality (5).
However, existing studies have not fully considered the evolving landscape of surgery, which increasingly tends toward minimally invasive procedures involving novel environments and technologies. Robot-assisted surgery, introduced in the early 2000s, has gained global prominence. Initially, the DaVinci System (Intuitive Surgical, Sunnyvale, CA, USA) was the sole robotic system available, but, in recent years, the landscape has changed thanks to the introduction in the global market of new robotic platforms (6,7). Consequently, both novice and expert surgeons are now confronted with more demanding challenges compared to the past.
Globally, the robotic approach is mainly used in the urological field, with the most frequently performed robot-assisted procedure being radical prostatectomy (8).
In light of these considerations, which would be the best path to provide effective and homogeneous technical and non-technical skills to a novice robotic surgeon (who could be a resident or a very senior and experienced surgeon)?
Rationale and objective
Between 2000 and 2013, approximately 10,624 adverse events associated with robotic procedures were documented in the United States across various surgical specialties (9). In response to these data, the European Commission of the World Health Organization initiated the Patient Safety in Robotic Surgery (SAFROS) project (10). The project aimed to investigate whether robotic surgery, conducted in adherence to safety criteria, could enhance the current safety standards achieved by traditional surgery. This involved an analysis of safety in robotic surgery, the formulation of safety requirements, and the establishment of safety procedures and verification protocols. Recognizing the pivotal role of surgical experience in improving patient outcomes (11-13), the project also emphasized the necessity for adequate training and preparation of robotic surgeons. The prioritization of structured robotic surgical training programs became imperative to standardize procedures, a recognized safety factor for patients (14).
Currently, there are limited standardized curricula available for training in robot-assisted radical prostatectomy (RARP) (15,16). For instance, the European Association of Urology Robotic Urologic Section (ERUS) has standardized major robotic urological procedures, including RARP They have defined specific phases, steps, and error metrics to enable and enhance the reproducibility of training and training standards for surgeons entering the field. The ERUS curriculum encompasses a tailored training program with different levels, including e-learning, simulation-based training (utilizing virtual reality simulators, dry and wet laboratories), and modular console training in the operating room (15,17). However, this training program cannot be easily by accessed by surgeons from all around the world, in terms of cost and accessibility.
The aim of this narrative review is to synthesize the scientific literature pertaining to RARP training, shedding light on diverse methodologies and recent innovations. We present this article in accordance with the Narrative Review reporting checklist (available at https://cco.amegroups.com/article/view/10.21037/cco-24-14/rc).
Methods
A literature review was performed following the criteria specified in Table 1. The article search strategy encompassed all articles meeting the criteria in Table 1, with a filter for those published after the year 2000. References cited within each article were thoroughly examined, and primary sources were selected for further investigation.
Table 1
Items | Specification |
---|---|
Date of search | 04/12/2023 to 07/12/2023 |
Databases searched | PubMed |
Search terms used | Training AND robotic AND prostatectomy; robotic training AND prostatectomy AND learning; simulator AND robotic AND prostatectomy |
Timeframe | 2000 to present |
Inclusion criteria | All study types from case reports to randomized trials were included except for editorial letters. Only studies in English were considered |
Selection process | All studies were selected based on their relevance to each subsection within the review article. Studies were initially chosen by the authors (M.T., A.P.) and were reviewed by senior author (C.C.R.) |
This narrative review will explore various aspects of robotic surgery training, with a specific emphasis on RARP. The exploration will commence by scrutinizing the diverse levels of training complexity and will subsequently progress to various training methodologies, with a particular focus on highlighting the latest innovations in this field.
Levels of training
In the past, surgeons underwent training following the Halsted apprenticeship approach: frequently caricatured as see one, do one, teach one (18). This approach primarily relied only on active patient practice by the trainee surgeons, with at best minimal preclinical training. Consequently, this approach did not ensure adequate preparation for surgeons and in truth posed potential safety risks to patients. Numerous studies in the literature demonstrate that insufficient training, resulting in deficient technical or non-technical skills, can lead to a higher incidence of complications (9). Intraoperative complications not only impact patients but also contribute to elevated healthcare costs (4). Hence, it is crucial to invest time and resources in an effective and dedicated training process. One of the best validated training methodologies is proficiency-based progression (PBP) training, which notably diverges from conventional approaches (19-21). It involves a meticulous delineation of the operative procedure in phases, steps, errors and critical errors, encompassing both technical and non-technical skills. The aim is to pinpoint specific intraoperative performance metrics indicative of both optimal and suboptimal performance using a binary evaluation. Once these objective metrics are established and validated, trainees must continue their metric-based, deliberate practice training until they demonstrate a quantitatively predefined proficiency benchmark, i.e., proficiency level. Throughout this practice, continuous formative feedback is provided to the trainee, aligning with the concept of deliberate practice. The proficiency level is determined based on the mean performance of experienced practitioners executing the same training tasks. In a systematic review and meta-analysis and a comparison with non-PBP training, ratio-of-means results showed that PBP training reduced the number of performance errors by 60%, decreased procedural time by 15%, and increased the number of steps performed by 47% (21). In conclusion, surgeons receiving the PBP training improved their overall procedural performance, when compared to conventional or quality-assured training.
Robotic surgery poses greater demands on surgeons compared to standard open or laparoscopic procedures for several reasons. The surgeon deals with new technologies and complex tools that enable more precise and accurate procedures. This is particularly evident in RARP, where magnification views and precise robotic instruments movement enable optimal tissue dissection. These characteristics increase the likelihood of preserving nerves and tissues, resulting in improved functional outcomes (22,23). Therefore, the skills required for robotic surgery are unique and warrant specialized training.
Drawing from the aviation field’s experience, the transition from theoretical training to practical execution cannot occur directly. Intermediate training steps, such as virtual reality simulators, are essential to ensure trainees are well-prepared when they are ready to undertake the actual procedures.
The subsequent paragraphs will provide an overview of each stage of robotic surgery training in the context of RARP, starting from online learning and progressing to console mentoring in the operating theater (Figure 1).
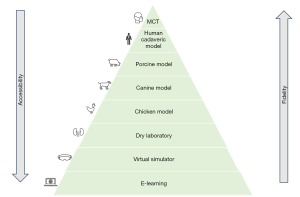
E-learning
E-learning, as defined by Ruiz et al. (24), utilizes internet technologies to deliver a diverse range of solutions aimed at enhancing knowledge and performance, making it a readily accessible training source in the field of surgery. This can range from simple videos on platforms like YouTube to well-structured, validated online courses. However, the risk of encountering low-quality information from online sources is considerable (25). Arslan et al. assessed the content quality of 226 YouTube videos on laparoscopic and RARP using the Prostatectomy Assessment and Competency Evaluation (PACE) score (26). According to PACE, YouTube contains high-quality videos for both laparoscopic and RARP. Nevertheless, there is a lack of objective parameters to predict the educational quality of these videos.
In contrast, ERUS offers a validated and high-quality online RARP course (15). Fellows undergo e-learning via the e-module developed by the ERUS expert panel, covering each RARP step through specific videos created by robotic surgery experts. These videos highlight potential errors and critical mistakes that fellows might make. Furthermore, a mandatory final assessment is required before progressing to the next level of training (27). Puliatti et al. present intriguing findings on the impact of e-learning training for performing robotic suturing, anastomosis, and knot tying tasks using the “Venezuelan Chicken model”. The study involved four distinct groups: (I) the full PBP group had access to online materials detailing task performance, coupled with the requirement to meet predefined proficiency benchmarks before advancing in training; (II) the e-learning group accessed the same online materials as the first group but without the obligation to achieve a predefined proficiency benchmark; (III) the Traditional training group received face-to-face lectures at the training center on task execution before commencing practical modules; (IV) the Apprenticeship group relied on information from peer-reviewed publications describing the suturing and knot tying tasks, including performance metrics for proficiency assessment. However, at the conclusion of the randomized clinical trial, none of the trainees demonstrated proficiency in completing the robotic surgery task (27).
In conclusion, e-learning stands out as a crucial, but not sufficient, component of training for novice robotic surgeons. The potential resources for online learning are vast, but there are challenges, as neither the quality of content (as observed with YouTube videos) nor open access (as seen with the ERUS curriculum) is consistently guaranteed.
Virtual reality simulator
Virtual reality entails a three-dimensional computer-generated “world” where an individual can navigate and interact as if present in an imaginary setting (1). This simulation has been essential to aviation training for the mid 1950s, evolving to a point where pilots dedicate initial training hours to flawless take-offs and landings within a simulator before venturing into an actual aircraft. Satava, in 1993, was among the pioneers discussing virtual reality simulators in surgical training, envisioning a future where surgical residents gain new perspectives on anatomy and practice procedures until achieving perfection prior to patient surgery.
As per Satava et al., an ideal surgical simulator should feature precise detailing and high interactivity (28,29). Visuals must be anatomically accurate, simulated organs should mimic natural properties, and body parts should be manipulable with realistic responses, such as bleeding or fluid leakage. These principles find application in the context of RARP, where virtual reality simulators, like “Da Vinci SimNow™”, replicate key procedural steps. Additionally, various simulators, including The Robotic Surgical Simulator (RoSS) and Da Vinci Skills Simulator (dVSS), present diverse features. Innovations, like Hands-On Surgical Training (HOST™) in RoSS™ and Maestro AR in dV-Trainer™, enable trainees to register procedure-specific movements using tailored videos, mimicking the surgeon’s actions in real time (30). Chowriappa et al. investigated the potential benefit of virtual reality simulators made by the Hands-on Surgical Training (HoST) technology on robotic skills in performing urethrovesical anastomosis using an inanimate model on the daVinci Surgical System. The participants belonging in the HoST training group exhibited a higher score in needle driving, needle positioning and suture placement, relative to the control group (29).
In conclusion, virtual reality simulators are fundamental for teaching and surgeon development, offering substantial room for improvement (31). Future advancements are anticipated to enhance anatomical precision, tissue physics, and instrument handling fidelity and responsiveness.
Dry laboratory
The dry laboratory provides cost-effective training using inanimate bench-top models, simulating real-time challenges. It serves as a valuable interface for learning initial console troubleshooting, especially concerning camera and clutch control, hand positioning, and the use of the reserve arm (28). Consumables for dry laboratory exercises range from routine beads, needles, and sutures to sophisticated vascular and bowel models (32). However, dry laboratory exercises have some limits, including the absence of bleeding and the difficulty in maintaining a standardized record or assessment method.
Dry laboratory exercises enable the acquisition of basic robotic skills but face challenges in replicating complex steps of procedures like RARP (Figure 2). In particular, there is a lack of studies in current literature showing dry laboratory model exercises specifically related to RARP. Conversely, two studies showcasing a dry laboratory model for robotic-assisted pancreaticojejunostomy (33) and coronary anastomoses (34) are available.
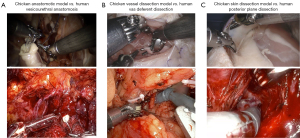
Therefore, the urological scientific community should direct its attention toward developing an efficient dry laboratory model exercise tailored for RARP. Given that RARP is the most widely performed robot-assisted procedure globally, leveraging new technologies such as 3-dimensional printing could be advantageous in this endeavor.
Wet laboratory
The wet laboratory constitutes a more advanced level of training in comparison to the virtual reality simulator and the dry laboratory model (28). It provides trainees with the opportunity to acquire fundamental robotic skills, including tissue handling, dissection, excision, diathermy, and suturing techniques. Furthermore, trainees can progress to performing complete surgical procedures, such as RARP (28). The subsequent sections outline the primary advantages and disadvantages of the most utilized models for dry laboratory training (Table 2).
Table 2
Types of wet laboratory | Costs | Storage | Blood perfusion | Anatomical fidelity | Disposal | Lymph node dissection |
---|---|---|---|---|---|---|
Chicken model | + | + | − | + | + | − |
Canine model | ++ | ++ | + | ++ | +++ | ++ |
Porcine model | +++ | +++ | ++++ | ++ | +++ | ++++ |
Human cadaveric model | +++++ | +++ | + | ++++ | +++ | +++ |
The number of “+” represents how much the type of wet laboratory is characterized by that specific item. If the type of wet laboratory is not characterized by the specific item, the symbol “−” is used.
Chicken model
One commonly utilized model in robotic surgery training involves frozen animals, and among these, chickens are frequently employed (35). In the context of RARP training, numerous studies have explored the use of chicken models for improve the technical skills required in various RARP steps (36-39) (Figure 2). For example, Puliatti et al. developed performance metrics (procedure steps, errors, and critical errors) for robotic suturing and knot tying, utilizing a chicken anastomotic model with the PBP training methodology (38). With this exercise trainees learn how to perform the anastomosis between the stomach and the cloaca of the chicken. Another exercise employing the chicken model, useful for RARP training, is skin dissection in the chicken leg (37). This exercise enables trainees to navigate a narrow space using three robotic instruments to develop the plane between the posterior surface of the prostate and rectum during the RARP procedure. Despite the limitations of these models, restricted to basic exercises and a relatively short learning curve for surgeons, the chicken model stands out as a cost-effective and easily accessible resource, making it universally applicable.
Canine model
The cadaveric canine model offers surgeons comprehensive training on all steps of RARP, ranging from port placement to vesicourethral anastomosis (40). One notable advantage of this model in the context of RARP lies in its resemblance to the human prostate. Nerve sparing in the canine model presents well-identifiable neurovascular bundles, which, though thinner than in humans, affords an even greater challenge in their preservation, providing a valuable training experience for surgeons.
Furthermore, the realism extends to the vesicourethral anastomosis, demonstrating an almost identical anatomical configuration. The catheterization process also allows for the performance of a leakage test. Despite the similarities to the human prostatic gland, there are anatomical differences, such as the absence of the seminal vesicles and the deep venous complex (41). The lack of blood perfusion is another factor to consider, potentially constraining the fidelity of nodes dissection and creating a gap between the surgeon’s experience and a real-life scenario that involves the risk of bleeding. In addition, the difficulty in sourcing canine cadavers is a notable limitation in utilizing this model.
Porcine model
The live porcine model is the primary choice in surgical training (42). In contrast to the cadaveric canine model, the presence of blood perfusion in the live porcine model enables trainees to enhance their technical skills in plane dissection and hemostasis control, critical aspects of surgical training (43). A valuable exercise in this model is lymph node dissection due to the similarity in human anatomy and the associated risk of bleeding. However, a limitation of this model is the relatively small size of the porcine prostate, which differs significantly from the human prostate, resembling a minor bulge in the urethra. Obvious limits of this model include the costs associated with pig farming, the need for anesthesia management during the procedure, and the animal disposal.
Human cadaveric model
The human cadaveric model stands out as the optimal training model in terms of anatomical fidelity (44). It is applicable across and within surgical specialties. The limited availability however, and high costs make this model challenging to use in many countries. Additionally, similar to the cadaveric canine model, the absence of blood perfusion accentuates differences in tissue quality between living and cadaveric specimens. It is also important to take into account the potential risk of infection when using cadavers for training purposes. Thus, wet laboratories offer varying levels of training complexity, allowing surgeons to develop basic robotic surgical skills and progress to mastering all the steps of complex procedures such as RARP. Unfortunately, to the best of our knowledge, there are no studies examining the effect of robotic skills training using wet laboratory models on surgical clinical outcomes. This gap should be addressed in the future publications.
It is crucial to recognize that the use of animal models may be restricted by legal considerations. Animal research within the European Union is governed by Directive 2010/63/EU on the protection of animals used for scientific purposes (45), which adheres to the principle of the “Three Rs”: replacement, reduction, and refinement. Replacement refers to methods, strategies, or approaches that don’t involve live animals. Reduction encompasses approaches that use fewer animals to achieve the same objective. Refinement involves modifying procedures or practices throughout an experimental animal’s life to minimize suffering and improve well-being or transitioning from more sentient species to less sentient ones. Each training center in Europe must adhere to this legislation. For instance, the ORSI Academy training center in Belgium mandates that each trainee sign a “Declaration on animal welfare”, outlining specific rules for training procedures. Unfortunately, legislation varies worldwide, impeding the standardization and dissemination of high-quality wet laboratory training. While strict legislation is essential for ensuring animal welfare, it also poses a reasonable limitation on establishing high-level training centers in every country. Consequently, both novice and expert surgeons seeking robotic surgery training may need to travel abroad for adequate training. For example, Rocha et al. documented how surgeons in Brazil interested in learning RARP began their training program by spending a week at Florida Hospital (Celebration, FL, USA) (46).
This aspect could pose a limitation in providing a standardized training process for surgeons worldwide.
Modular console training
Teaching robotic surgery significantly differs from traditional open or laparoscopic surgery. In open or laparoscopic surgery, the supervising surgeon is in close proximity to the trainee, sharing the same view of the operation field. Additionally, the supervising surgeon has the capability to assume control over the trainee’s maneuvers at any point during the procedure (30). The modular console training phase is particularly critical in robotic surgery education. Here, the surgeon begins applying robotic skills to actual patients in the operating theater.
Therefore, mentors bear a significant responsibility, primarily to the patients and also to the trainees. To mitigate patient risk, console training initiates with simpler and safer steps, such as bladder detachment, seminal vesicle dissections, or the final stitches of the vesicourethral anastomosis in the context of RARP (47). Once the trainee and the mentor feel comfortable performing these steps, they can progress to more complex ones. The ERUS-validated RARP curriculum involves the graduated submission of trainee videos for the purpose of monitoring improvement, culminating in the submission of a full RARP procedure video at the fellowship’s conclusion (15,17).
An important consideration in console training is the choice of the robotic platform. Over the past few years, the landscape has evolved with the introduction of new robotic platforms into the global market (7,48-50). These platforms can vary in terms of their robotic console design, either being close (as seen in the DaVinci Intuitive system) or open (as seen in the HUGO Robot-Assisted Surgical System) (6).
Indeed, when a closed console is utilized, as in the case of the DaVinci system, the interaction between the mentor and trainees may be challenging. Although the DaVinci system offers a double console specifically designed for training purposes, its availability is not guaranteed and is usually quite expensive. For instance, in the ERUS program, the host center must be equipped with a double console. On the other hand, in training with an open console, the mentor can easily guide trainees by being physically close to them. All these approaches assume that the trainer and trainees need to be in the same location.
Discussion of the key findings
In the preceding paragraphs, we have provided a detailed description of various training methodologies. The current narrative review highlights two main points: the diversity in possible training complexity levels and the significant barriers to obtaining adequate robotic training all over the world.
Training for robotic surgery presents a new challenge in today’s surgical field, demanding unique skills and technologies distinct from traditional open or laparoscopic methods. The surgeon operating with the robotic system benefits from three-dimensional imaging of the surgical area (7). The instruments, with their seven degrees of freedom, effectively emulate the flexibility of the human wrist. Additionally, during the robotic surgery, both the console surgeon and the assisting team member at the patient’s side must rely on visual cues as there is no tactile feedback. Understanding the spatial relationships of the instruments beyond direct line of sight is crucial for minimizing inadvertent tissue injury and avoiding collisions. These particular factors pose challenges to robotic surgery training, especially for surgeons used to straight and unarticulated laparoscopic instruments. Conversely, for resident novices in minimally invasive surgery, the learning curve for acquiring robotic skills may be quicker. However, further studies are necessary to validate our hypothesis.
Moreover, an additional challenge to obtain adequate training, is the possibilities to have an additional console or a virtual reality simulator. At the start, trainees should use virtual reality simulators, which need both the free robot console and a special simulator, which has specific costs. Ideally, having a double console in each robotic center would greatly enhance training opportunities for surgery residents and experienced surgeons venturing into robotic surgery. This setup would enable them to receive direct guidance and instruction from experts during or even after surgeries, thereby facilitating comprehensive training and skill development. As training gets more advanced, like using dry laboratory exercises or animal models in wet laboratory sessions, it gets even harder because of the permissions and the equipment needed. Consequently, robotic training incurs higher costs and demands substantial space to ensure optimal training conditions.
Regardless of the equipment and furniture that are essential to provide the correct training, the training approach is also an important factor. Among various training methodologies, the PBP approach appears most effective, as demonstrated by the randomized clinical trial conducted by De Groote et al. (51,52). Specifically, the PROVESA trial, a multicentric, prospective, randomized study, compared PBP training with traditional training for robotic suturing and knot-tying anastomosis skills. The PBP group exhibited a 51% reduction in the number of performance errors from baseline to final assessment (51). Mazzone et al. summarized in a recent published systematic review and metanalysis, the positive impact of PBP approach relative to standard training methodology in terms of number of performance errors, procedural time, and number of steps performed (21). However, future larger studies are needed to confirm these results in both clinical outcomes (such as complications rates or functional results) and healthcare costs.
The above observations underscore significant barriers to training for both surgical residents and experienced surgeons transitioning to robotic surgery. The discrepancy between institution possibilities, influenced by financial constraints, international collaborations for clinical fellowships, or availability of onsite training facilities, further compound these challenges. Ideally, every institution embracing robotic surgery should adopt the most effective training approach to ensure optimal performance across all levels of surgical expertise. However, achieving this goal remains distant at present due to important barriers represented by different languages, healthcare systems and financial possibilities across the countries.
Finally, last consideration should be done. With advancements in technology, especially with the introduction of 5G, there is a shift in training methodologies towards tele-mentoring (53). This approach enables interaction between mentors and trainees regardless of geographic location. Nevertheless, significant challenges persist (53). First, safety considerations are paramount. Connectivity issues, such as inherent latency and signal delays, pose a potential threat to patient safety. Moreover, the risk of cyber-attacks should not be underestimated. Second, legal aspects require careful attention. The licensure of the treating surgeon and the delineation of geographic boundaries in the patient-surgeon relationship must be clearly defined. Third, financial and economic factors must be taken into account. While telesurgery may offer advantages by reducing travel costs for expert surgeons, the expenses associated with developing and implementing telementoring, tele-assist, or telesurgical services need to be carefully considered. Last, ethical considerations are critical. Telesurgery introduces patients to higher risks of hacking or technical failures, which could result in intraoperative complications. Thus, specific regulations addressing these concerns must be developed for the future. In conclusion, the feasibility of utilizing tele-mentoring and telesurgery is increasingly tangible with each passing year. However, significant challenges persist and must be addressed comprehensively.
Conclusions
In conclusion, the landscape of surgical training, particularly in the context of robot-assisted procedures like radical prostatectomy, is undergoing transformative changes. The traditional “see one, do one, teach one” approach is giving way to more scientific and standardized training methodologies, such as PBP. PBP, with its meticulous focus on both technical and non-technical skills, has demonstrated significant improvements in trainee performance, reducing errors and procedural time.
The advent of robotic surgery introduces unique challenges, requiring surgeons to navigate new technologies and tools to master their subject matter and skills. From e-learning to modular console training, each step in the training process plays a pivotal role. However, challenges persist, ranging from the availability and accessibility of standardized curricula to ethical concerns surrounding the use of animal models. As technology advances, the potential for tele-mentoring via platforms like 5G offers new possibilities in training, albeit with safety, legal, financial, economic, and ethical considerations.
This narrative review underscores the evolving nature of surgical training, emphasizing the need for standardized, effective, and accessible programs to equip surgeons globally with the skills needed for intricate procedures like RARP. The scientific community ought to investigate the impact of comprehensive robotic surgical training on surgical outcomes and healthcare costs. Such research can provide empirical evidence to support future investments from institutions. This parallels the emphasis historically placed on airplane pilot training, highlighting the importance of ensuring proficiency for optimal performance and safety in complex tasks.
Acknowledgments
Funding: None.
Footnote
Provenance and Peer Review: This article was commissioned by the editorial office, Chinese Clinical Oncology for the series “New Evidence and Advances in Surgical Treatment of Prostate Cancer”. The article has undergone external peer review.
Reporting Checklist: The authors have completed the Narrative Review reporting checklist. Available at https://cco.amegroups.com/article/view/10.21037/cco-24-14/rc
Peer Review File: Available at https://cco.amegroups.com/article/view/10.21037/cco-24-14/prf
Conflicts of Interest: All authors have completed the ICMJE uniform disclosure form (available at https://cco.amegroups.com/article/view/10.21037/cco-24-14/coif). The series “New Evidence and Advances in Surgical Treatment of Prostate Cancer” was commissioned by the editorial office without any funding or sponsorship. D.C. served as the unpaid Guest Editor of the series and serves as an unpaid editorial board member of Chinese Clinical Oncology from August 2022 to July 2024. S.P. and S.F. also served as the unpaid Guest Editors of the series. The authors have no other conflicts of interest to declare.
Ethical Statement: The authors are accountable for all aspects of the work in ensuring that questions related to the accuracy or integrity of any part of the work are appropriately investigated and resolved. All clinical procedures described in this study were performed in accordance with the ethical standards of the institutional and/or national research committee(s) and with the Helsinki Declaration (as revised in 2013). Written informed consent was obtained from the patients for the publication of this article and accompanying images.
Open Access Statement: This is an Open Access article distributed in accordance with the Creative Commons Attribution-NonCommercial-NoDerivs 4.0 International License (CC BY-NC-ND 4.0), which permits the non-commercial replication and distribution of the article with the strict proviso that no changes or edits are made and the original work is properly cited (including links to both the formal publication through the relevant DOI and the license). See: https://creativecommons.org/licenses/by-nc-nd/4.0/.
References
- Satava RM. Virtual reality surgical simulator. The first steps. Surg Endosc 1993;7:203-5. [Crossref] [PubMed]
- Flin R, Maran N. Identifying and training non-technical skills for teams in acute medicine. Qual Saf Health Care 2004;13:i80-4. [Crossref] [PubMed]
- Yule S, Paterson-Brown S. Surgeons' non-technical skills. Surg Clin North Am 2012;92:37-50. [Crossref] [PubMed]
- Gawande AA, Zinner MJ, Studdert DM, et al. Analysis of errors reported by surgeons at three teaching hospitals. Surgery 2003;133:614-21. [Crossref] [PubMed]
- Birkmeyer JD, Finks JF, O'Reilly A, et al. Surgical skill and complication rates after bariatric surgery. N Engl J Med 2013;369:1434-42. [Crossref] [PubMed]
- Frego N, Ruvolo CC, Mottrie A. Opening up the Market to New Robotic Platforms: The Best Way To Handle New Options. Eur Urol 2024;85:190-2. [Crossref] [PubMed]
- Farinha R, Puliatti S, Mazzone E, et al. Potential Contenders for the Leadership in Robotic Surgery. J Endourol 2022;36:317-26. [Crossref] [PubMed]
- Abboudi H, Khan MS, Guru KA, et al. Learning curves for urological procedures: a systematic review. BJU Int 2014;114:617-29. [Crossref] [PubMed]
- Collins JW, Dell'Oglio P, Hung AJ, et al. The Importance of Technical and Non-technical Skills in Robotic Surgery Training. Eur Urol Focus 2018;4:674-6. [Crossref] [PubMed]
- CORDIS | European Commission. [cited 2024 Jan 26]. Patient Safety in Robotic Surgery | SAFROS Project | Fact Sheet | FP7. Available online: https://cordis.europa.eu/project/id/248960/it
- Collins JW, Tyritzis S, Nyberg T, et al. Robot-assisted radical cystectomy (RARC) with intracorporeal neobladder - what is the effect of the learning curve on outcomes? BJU Int 2014;113:100-7. [Crossref] [PubMed]
- Vickers A, Savage C, Bianco F, et al. Cancer control and functional outcomes after radical prostatectomy as markers of surgical quality: analysis of heterogeneity between surgeons at a single cancer center. Eur Urol 2011;59:317-22. [Crossref] [PubMed]
- Larcher A, Muttin F, Peyronnet B, et al. The Learning Curve for Robot-assisted Partial Nephrectomy: Impact of Surgical Experience on Perioperative Outcomes. Eur Urol 2019;75:253-6. [Crossref] [PubMed]
- Palagonia E, Mazzone E, De Naeyer G, et al. The safety of urologic robotic surgery depends on the skills of the surgeon. World J Urol 2020;38:1373-83. [Crossref] [PubMed]
- Volpe A, Ahmed K, Dasgupta P, et al. Pilot Validation Study of the European Association of Urology Robotic Training Curriculum. Eur Urol 2015;68:292-9. [Crossref] [PubMed]
- Harrison P, Raison N, Abe T, et al. The Validation of a Novel Robot-Assisted Radical Prostatectomy Virtual Reality Module. J Surg Educ 2018;75:758-66. [Crossref] [PubMed]
- Mottrie A, Mazzone E, Wiklund P, et al. Objective assessment of intraoperative skills for robot-assisted radical prostatectomy (RARP): results from the ERUS Scientific and Educational Working Groups Metrics Initiative. BJU Int 2021;128:103-11. [Crossref] [PubMed]
- Kotsis SV, Chung KC. Application of the "see one, do one, teach one" concept in surgical training. Plast Reconstr Surg 2013;131:1194-1201. [Crossref] [PubMed]
- Gallagher AG. Metric-based simulation training to proficiency in medical education:- what it is and how to do it. Ulster Med J 2012;81:107-13. [PubMed]
- Gallagher AG, O’Sullivan GC. Fundamentals of Surgical Simulation. London: Springer London; 2012 [cited 2023 Sep 13]. Available online: http://link.springer.com/10.1007/978-0-85729-763-1
- Mazzone E, Puliatti S, Amato M, et al. A Systematic Review and Meta-analysis on the Impact of Proficiency-based Progression Simulation Training on Performance Outcomes. Ann Surg 2021;274:281-9. [Crossref] [PubMed]
- Nyberg M, Hugosson J, Wiklund P, et al. Functional and Oncologic Outcomes Between Open and Robotic Radical Prostatectomy at 24-month Follow-up in the Swedish LAPPRO Trial. Eur Urol Oncol 2018;1:353-60. [Crossref] [PubMed]
- Tewari A, Srivasatava A, Menon M, et al. A prospective comparison of radical retropubic and robot-assisted prostatectomy: experience in one institution. BJU Int 2003;92:205-10. [Crossref] [PubMed]
- Ruiz JG, Mintzer MJ, Leipzig RM. The impact of E-learning in medical education. Acad Med 2006;81:207-12. [Crossref] [PubMed]
- Morra S, Napolitano L, Collà Ruvolo C, et al. Could YouTubeTM encourage men on prostate checks? A contemporary analysis. Arch Ital Urol Androl 2022;94:285-90. [Crossref] [PubMed]
- Arslan B, Gönültaş S, Gökmen E, et al. Does YouTube include high-quality resources for training on laparoscopic and robotic radical prostatectomy? World J Urol 2020;38:1195-9. [Crossref] [PubMed]
- Puliatti S, Amato M, Farinha R, et al. Does quality assured eLearning provide adequate preparation for robotic surgical skills; a prospective, randomized and multi-center study. Int J Comput Assist Radiol Surg 2022;17:457-65. [Crossref] [PubMed]
- Sridhar AN, Briggs TP, Kelly JD, et al. Training in Robotic Surgery-an Overview. Curr Urol Rep 2017;18:58. [Crossref] [PubMed]
- Chowriappa A, Raza SJ, Fazili A, et al. Augmented-reality-based skills training for robot-assisted urethrovesical anastomosis: a multi-institutional randomised controlled trial. BJU Int 2015;115:336-45. [Crossref] [PubMed]
- El Sherbiny A, Eissa A, Ghaith A, et al. Training in urological robotic surgery. Future perspectives. Arch Esp Urol 2018;71:97-107. [PubMed]
- Seymour NE, Gallagher AG, Roman SA, et al. Virtual reality training improves operating room performance: results of a randomized, double-blinded study. Ann Surg 2002;236:458-63; discussion 463-4. [Crossref] [PubMed]
- Ramos P, Montez J, Tripp A, et al. Face, content, construct and concurrent validity of dry laboratory exercises for robotic training using a global assessment tool. BJU Int 2014;113:836-42. [Crossref] [PubMed]
- Wei F, Xu M, Lai X, et al. Three-dimensional printed dry lab training models to simulate robotic-assisted pancreaticojejunostomy. ANZ J Surg 2019;89:1631-5. [Crossref] [PubMed]
- Gulbins H, Boehm DH, Reichenspurner H, et al. 3D-visualization improves the dry-lab coronary anastomoses using the Zeus robotic system. Heart Surg Forum 1999;2:318-24; discussion 324-5. [PubMed]
- Sotelo RJ, Astigueta JC, Carmona OJ, et al. Chicken gizzard: a new training model for laparoscopic urethrovesical anastomosis. Actas Urol Esp 2009;33:1083-7. [Crossref] [PubMed]
- Cacciamani G, De Marco V, Siracusano S, et al. A new training model for robot-assisted urethrovesical anastomosis and posterior muscle-fascial reconstruction: the Verona training technique. J Robot Surg 2017;11:123-8. [Crossref] [PubMed]
- Puliatti S, Amato M, Mazzone E, et al. Development and Validation of the Metric-Based Assessment of a Robotic Dissection Task on an Avian Model. J Surg Res 2022;277:224-34. [Crossref] [PubMed]
- Puliatti S, Mazzone E, Amato M, et al. Development and validation of the objective assessment of robotic suturing and knot tying skills for chicken anastomotic model. Surg Endosc 2021;35:4285-94. [Crossref] [PubMed]
- Puliatti S, Amato M, Mazzone E, et al. Development and validation of the metric-based assessment of a robotic vessel dissection, vessel loop positioning, clip applying and bipolar coagulation task on an avian model. J Robot Surg 2022;16:677-85. [Crossref] [PubMed]
- Jamet A, Hubert N, Maire J, et al. Robot-assisted radical prostatectomy training: Description of a canine cadaveric model. Int J Med Robot 2022;18:e2381. [Crossref] [PubMed]
- Price DT, Chari RS, Neighbors JD Jr, et al. Laparoscopic radical prostatectomy in the canine model. J Laparoendosc Surg 1996;6:405-12. [Crossref] [PubMed]
- Biyani CS, Van Cleynenbreugel B, Mottrie A. editors. Practical Simulation in Urology. Cham: Springer International Publishing; 2022 [cited 2024 Jan 26].
- Swindle MM. Swine as replacements for dogs in the surgical teaching and research laboratory. Lab Anim Sci 1984;34:383-5. [PubMed]
- Gilbody J, Prasthofer AW, Costa ML. The use and effectiveness of cadaveric workshops in higher surgical training: a systematic review. Ann R Coll Surg Engl 2011;93:347-52. [Crossref] [PubMed]
- Marinou KA, Dontas IA. European Union Legislation for the Welfare of Animals Used for Scientific Purposes: Areas Identified for Further Discussion. Animals (Basel) 2023;13:2367. [Crossref] [PubMed]
- Rocha R, Fiorelli RK, Buogo G, et al. Robotic-assisted laparoscopic prostatectomy (RALP): a new way to training. J Robot Surg 2016;10:19-25. [Crossref] [PubMed]
- Lovegrove C, Novara G, Mottrie A, et al. Structured and Modular Training Pathway for Robot-assisted Radical Prostatectomy (RARP): Validation of the RARP Assessment Score and Learning Curve Assessment. Eur Urol 2016;69:526-35. [Crossref] [PubMed]
- Bravi CA, Balestrazzi E, De Loof M, et al. Robot-assisted Radical Prostatectomy Performed with Different Robotic Platforms: First Comparative Evidence Between Da Vinci and HUGO Robot-assisted Surgery Robots. Eur Urol Focus 2024;10:107-14. [Crossref] [PubMed]
- Paciotti M, Bravi CA, Mottaran A, et al. Nerve-sparing robot-assisted radical prostatectomy with the HUGO™ robot-assisted surgery system using the 'Aalst technique'. BJU Int 2023;132:227-30. [Crossref] [PubMed]
- Bravi CA, Mottaran A, Sarchi L, et al. Transitioning from Da Vinci Si to Xi: assessing surgical outcomes at a high-volume robotic center. World J Urol 2023;41:3737-44. [Crossref] [PubMed]
- De Groote R, Puliatti S, Amato M, et al. Proficiency-based progression training for robotic surgery skills training: a randomized clinical trial. BJU Int 2022;130:528-35. [Crossref] [PubMed]
- De Groote R, Puliatti S, Amato M, et al. Discrimination, Reliability, Sensitivity, and Specificity of Robotic Surgical Proficiency Assessment With Global Evaluative Assessment of Robotic Skills and Binary Scoring Metrics: Results From a Randomized Controlled Trial. Ann Surg Open 2023;4:e307. [Crossref] [PubMed]
- Hung AJ, Chen J, Shah A, et al. Telementoring and Telesurgery for Minimally Invasive Procedures. J Urol 2018;199:355-69. [Crossref] [PubMed]