Radiation for skull base meningiomas: review of the literature on the approach to radiotherapy
Introduction
Meningiomas are indolent, slow growing tumours that generally arise from the arachnoid cap cells, which surround and adhere to the dura mater and so these tumors can arise from various anatomic regions within the central nervous system (CNS). Skull base meningiomas (SBM) are of particular challenge for radiotherapy due to their proximity to the brainstem, optic apparatus and a number of cranial nerves, posing risk of symptoms as the tumor progresses and greater risk of functional toxicities following local therapies such as surgery and radiation (1,2). SBM can be categorized as being from the anterior or middle cranial fossa (olfactory groove, tuberculum sellae, clinoid, sphenoid, wing and pure cavernous sinus) and from the posterior fossa (petrous, petroclival, jugular foramen, and foramen magnum) (3,4).
The main approaches for management of benign (WHO grade 1) and non-benign (WHO grade 2 and 3) meningiomas include active surveillance/observation, radical surgery, radical or partial surgery followed by radiotherapy, and radiotherapy alone (5). Definitive radiotherapy using conventionally fractionated external beam radiotherapy or stereotactic radiosurgery (SRS) are generally considered as the primary therapy when the risks outweigh the benefits for surgical resection due to patient comorbidities or if gross total resection is not feasible due to location (proximity to important critical structures). Adjuvant radiotherapy following surgery is recommended for grade 3 tumours and selected cases of grade 1 or 2 tumours. The aim of radiation treatment is to stop or stabilize the growth of the tumor in order to minimize symptom progression. The introduction of intensity modulated radiotherapy (IMRT) and single fraction SRS approaches have played an important role in improving the therapeutic ratio of radiotherapy for SBM by enabling delivery of conformal doses of radiation to cover the tumor while sparing the surrounding normal tissues (6-12) (Figures 1,2).
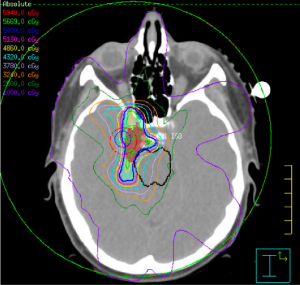
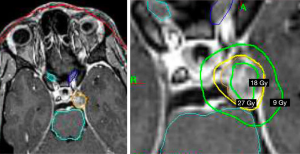
In this article, we provide a review of the published literature around radiotherapy for SBM, to clarify the role of EBRT, proton radiotherapy, SRS and stereotactic radiotherapy (SRT).
Fractionated radiotherapy
Improvements in RT technology and imaging have allowed precise delivery of high dose radiation to CNS lesions. Conventional RT, which typically uses 1.8–2.0 Gy fractions over a 5- to 7-week course, has been dramatically improved with the use of intensity-modulated radiotherapy (IMRT) or volumetric arch therapy (VMAT). These new techniques have improved the conformality of radiation dose delivery so that high-dose coverage can be achieved for the tumor while simultaneously providing better dose sparing of the chiasm, cranial nerves, brainstem, and other adjacent critical organs (e.g., optics nerves, globes or lens) (13,14) (Figure 1).
Evidence to guide the appropriate utilization of radiotherapy in the management of meningiomas remains limited. In clinical practice a number of factors are considered in the decision to proceed with radiation treatment including tumor size, resectability, tumor grade and whether the tumor has recurred following surgery. Additionally patient comorbidities and patient preference is taken into account when deciding whether the initial treatment is with radiation or surgical resection, given the lack of data to suggest one treatment provides better outcomes over the other.
Fractionated radiotherapy is often delivered with SRT immobilization and/or image-guided radiotherapy (IGRT) approaches to optimize the precision of radiation delivery in combination with IMRT techniques to improve the dose shaping around complex targets in close vicinity to critical normal structures. Several studies have shown durable tumor control with 10-year local control (LC) rates of 80% or higher following treatment with fractionated radiotherapy as primary or adjuvant treatment (5,15). In Table 1 we summarize data from published articles on three-dimensional (3-D) conformal or IMRT outcomes for SBM of all grades (7,10,16-21).
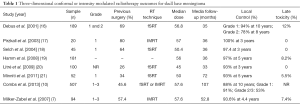
Full table
Milker-Zabel et al. published outcomes in 94 patients treated with IMRT for SBM (WHO grade 1-3) (7). In this case series, 26 patients had IMRT as primary treatment, 14 patients as postoperative treatment for residual disease and 54 patients as salvage radiotherapy for recurrent disease after initial surgical resection. With a median follow-up of 4.4 years (range, 1.6–82.7 months), the overall LC was 93.6% [69 patients (73.4%) with stable; 19 (20.2%) with a reduction of tumor volume and 6 patients with local tumor progression on MRI]. Pre-existing neurological deficits improved in 39.8% of patients, and worsened in 7.4% of patients (7). Combs et al. reported the long-term outcomes for 507 patients with SBM (WHO grade 1–3) who were treated with high precision radiotherapy defined as radiation treatment delivered either in stereotactic set-up or using IGRT that allowed for planning target volume margins (PTV) of 1–2 mm. A median total dose of 57.6 Gy (range, 25–68 Gy) was prescribed in median single doses of 1.8 Gy (range, 1.6–5 Gy). With a median follow-up time of 107 months, LC for the full cohort was 95% at 5 years and 88% at 10 years. Quality of life was unchanged or improved in 47.7% or 37.5% of the patients, respectively (10).
WHO grade 1 (benign) SBM
For benign SBM, fractionated radiotherapy is clinically most commonly used for large volume, growing and/or symptomatic tumours that are either unresectable or for patients who are inoperable. Following surgical resection, there is controversy about early post-operative radiation versus delayed radiotherapy at the time of tumor recurrence. Data support that early adjuvant radiotherapy improves LC, particularly following partial resection (8). In contrast, delaying radiotherapy until tumor recurrence can help spare toxicities associated with radiation and may be the favored approach for patients at low risk for tumor recurrence following gross total resection (10). Factors associated with higher risk of postsurgical progression/recurrence include the incomplete resection, prior recurrence following surgical resection, mitotic index, and possibly presence of peritumoral edema (22-26).
For benign tumours (WHO grade I) that are planned for fractionated radiotherapy, the gross target volume (GTV) is typically the enhancing tumor and/or the surgical bed. There is typically no microscopic margin added to intact benign meningiomas and minimal margin added following surgical resection. The conventional radiation doses generally range between 50–54 Gy delivered in 1.8–2 Gy daily fractions (Figure 1).
WHO grade 2–3 SBM
For non-benign meningiomas (WHO grade II–III), with greater concern for brain invasion, the target volume usually includes additional margin for microscopic spread. Additionally, due to the more aggressive biology, higher doses of radiation ranging between 60–66 Gy are typically used (23,27,28). Although the literature is limited, retrospective studies suggested that the use of higher doses may result in better tumor control. Goldsmith et al. analyzed 140 patients with intracranial meningioma (23 non-benign meningioma) to assess the impact of postoperative radiotherapy after subtotal resection (STR). With a median follow-up of 40 months, 5-year PFS was 89% and 85%, for benign and non-benign meningioma, respectively. There was a significant improvement on PFS with radiation doses >53 Gy (63%) when compared to ≤52 Gy (17%) for malignant meningioma (P=0.01) (22). In a series of 24 patients with non-benign (79% grade II) meningioma patients treated with combined proton and photon radiotherapy (mean total radiation dose of 65.01 CGE (range, 56–68 CGE) following STR (18/24 patients), the 5-year LC and OS for all patients was 46.7% and 53.2%, respectively. Authors also reported that combined proton and photons radiation dose >60 Gy significantly improved PFS (P=0.05) and OS (P=0.05) in their patients cohort (29).
Retrospective studies have demonstrated that early adjuvant radiotherapy for non-benign meningiomas results in mean improvement in 5-year PFS of 18% (range, 12–24%) (27,30-32). However, the majority of these studies have evaluated grade II and III meningiomas together, and the benefit may reflect selection bias of higher risk cases to receive adjuvant RT (23,24,33-36). Following STR of non-benign meningioma, patients are at high risk of further tumor progression and therefore are likely to have an improvement in LC with the addition of adjuvant radiotherapy. Aghi et al. reported the findings of 108 patients following STR for atypical grade II meningioma. Of 100 patients followed after STR alone, 41% experienced tumor progression at 5-years. This series reported that for the 8 patients who received adjuvant fractionated radiotherapy with a mean dose of 60.2 Gy to the resection bed plus a 1-cm margin, no recurrence was reported (23). Mair et al. reported that of 114 consecutive patients who underwent first-time resection of WHO Grade II atypical meningiomas, the use of postoperative radiotherapy didn’t demonstrate significant difference in outcomes. However in a subgroup analysis, for patients who had undergone STR, postoperative RT provided a significant PFS benefit when excluding the 5 patients who had undergone postoperative radiosurgery for a tumor remnant but no radiotherapy (P=0.043) (34).
As the goals of treatment shift towards optimizing functional outcome and minimizing treatment-related toxicity, close observation may be more frequently considered if a GTR is achieved for a grade II meningioma. Mirimanoff et al. reported long-term follow up (median follow up was not reported) outcomes of 225 benign meningiomas patients who underwent surgery alone. The PFS after GTR at 10, and 15 years was 80%, and 68%, respectively. By contrast PFS after STR at 10, and 15 years only was 45%, and 9%, respectively (35). Other series have reported local recurrence rates following GTR ranging from 7–23% at 5 years and 20–39% at 10 years (24,29,35-38).
For atypical (WHO grade II) meningiomas, considerable controversy exists regarding the optimal timing of radiotherapy after a GTR (30). Currently, two ongoing Phase II trials (RTOG 0539: Phase II Trial of Observation for Low-Risk Meningiomas and of Radiotherapy for Intermediate- and High-Risk Meningiomas—ClinicalTrials.gov identifier: NCT00895622 and EORTC 22042: Adjuvant Postoperative High-Dose Radiotherapy for Atypical and Malignant Meningioma: a Phase-II and Observation Study—ClinicalTrials.gov identifier: NCT00626730) are assessing the role of radiotherapy in the management of patients with non-benign meningioma using either 3DCRT or IMRT. RTOG 0539 is an observational study for low risk meningioma (group 1) patients and a phase II trial for intermediate risk meningioma patient (groups 2) and high-risk meningioma patients (group 3). Group 1 is an observation arm (65 patients) and is composed by grade 1 post GTR or STR. Group 2 encompasses patients with grade 1 recurrent disease or grade 2 post-GTR to 3D conformal radiotherapy or IMRT or protons 54 Gy/30 fractions. Group 3 includes grade 2 recurrent disease or grade 2 post STR or any grade 3 to IMRT only 60 Gy/30 fractions. The study enrolment is complete (n=244) and it is currently awaiting the primary endpoint, which is progression-free survival at 3 years. Preliminary results of RTOG 0539 presented at the ASTRO annual meeting in 2015 reported on one of the 3 study arms, group 2, which included patients with recurrent grade 1 meningioma after GTR or STR or new WHO Grade 2 after GTR. In this study arm, the 3-years LC was 98.0%. (Median follow-up was not published) Among 44 patients receiving IMRT, 4 (9%) had developed grade 2 acute toxicity, and 11 (25%) grade 2 late toxicity. No acute or late toxicity >2 was reported. The EORTC 22042 is an observational (group 1) and phase II (group 2) with a primary outcome of progression free survival. Group 1 includes grade 2 and 3 meningioma post GTR to 60 Gy in 30 fractions and group 2 grade 2 and 3 post STR to 70 Gy in 35 fractions. The study has completed accrual (n=78).
Malignant or anaplastic grade III meningiomas are aggressive and carry considerably higher recurrence risk and lower survival than their benign counterparts. For these tumours, radiation treatment is generally recommended following surgical resection for improved tumor control. Dziuk et al. compared surgery alone to surgery plus RT in 38 patients with WHO grade III tumours. Nineteen (50%) received initial postoperative RT and presented with significant better 5-year PFS (80% vs. 15% for surgery alone (P=0.002) (27). Several publications have reported that radiotherapy can also shrink the remaining tumour burden in addition to preventing tumor recurrence or progression (5,26,33).
Similar to grade II meningiomas, additional margin is added for grade III meningiomas for microscopic tumor extension. The radiation doses have generally ranged between 54–60 Gy delivered in 1.8–2 Gy daily fractions with an optional boost to gross disease up to 66–70 Gy. DeVries et al. and Hug et al. found an improvement in LC and overall survival (OS) with radiation dose greater than 60 Gy (39,40). Similarly, Boskos et al. reported improved OS with doses exceeding 60 Gy and a trend toward further improvement beyond 65 Gy (41).
Katz et al. reported the results of radiotherapy delivered using accelerated fractionation with and without SRS in patients with atypical and malignant meningioma. The study assessed 36 patients in the following 3 groups: (I) accelerated fractionation group (n=22) treated with 60 Gy at 1.5 Gy twice per day with or without SRS; (II) SRS alone (n=3) treated with 10–17.5 Gy (mean 14 Gy, median 15 Gy) in a single fraction; and (III) conventional radiation (n=7) 50–64.8 Gy (mean 57 Gy) plus SRS (n=2, 10 Gy for both). The study revealed that patients treated with accelerated fractionation (group 1) had a higher rate of grade 3–5 toxicities when compared to conventional radiation (group 3) (grade 3–5: 55% vs. 0%; P<0.05) with no improvement in PFS (45 vs. 55%; P=0.99) (42).
Recurrent meningioma
Recurrent meningiomas, including those that remain WHO grade I, have a considerably higher rate of recurrence after surgery alone than do newly diagnosed tumours (43). Despite more aggressive biologic behaviour these tumours often retain their original histopathologic grade(23), therefore despite the grade of the recurrent tumor, postoperative RT is typically offered to improve LC of the tumor. One series reported that the 5-year LC with postoperative radiotherapy at the time of first recurrence was 88% vs. 30% without radiation (P=0.01) and this translated into a 5-year OS outcome of 90% vs. 45% (P= not reported) (24).
Radiosurgery
SRS typically delivers a highly conformal, high dose of radiation in a single fraction. SRS has been applied more frequently to the practice over the last 2–3 decades. It has been used after STR or at the time of recurrence and as a definitive primary treatment for presumed benign meningiomas (44-52). SRS is usually considered effective and safe for meningioma that is limited in size up to 3cm in maximal diameter or 10 cc in volume and with sufficient distance from critical structures such as the optic chiasm, optics nerves, or brainstem. We present a summary of selected series on the use of SRS for skull base meningioma (Table 2).
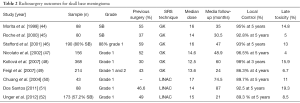
Full table
For mainly SBM and grade 1 meningiomas, 10 studies reported high LC rates with minor toxicities. With a median follow up of 46–86 months, PFS at 5 and 10 years was 89.7–99% and 79–83%, respectively. Treatment related toxicities, including radionecrosis, new onset or progression of cranial neuropathies, decline in cognition or memory, cerebellar deficits, alterations in body sensation, symptomatic edema, from SRS were largely under 10% (range, 1–17%). Based on retrospective series, high rates of LC have been achieved with a prescription dose of 12–16 Gy prescribed at the 50–80% isodose line, depending on the mode of SRS delivery (50,53-61). The largest series from Starke et al. analyzed 255 SBM and reported outcomes with a median follow-up of 78 months and median SRS prescribed doses of 14 Gy at mean prescription isodose line of 41% (range, 28–80%). Progression free survival at 5 and 10 years was 96% and 79%, respectively. Tumor regression and complication was reported in 49% and 10.6% of the cases, respectively. Treatment-related toxicities included new onset or progression of cranial neuropathies, which occurred in 22 patients (8.6%) and other neurological signs or symptoms occurred in an additional 6 patients (2%) (61).
Our review of the literature highlights 8 small series that focused on SRS for grade 2 meningiomas, mostly delivered after STR or at recurrence. With a median or mean follow up of 26–82 months, median or mean SRS dose of 12.4–18 Gy, PFS at 5 years was 58–83% based on 3 of the identified studies, respectively (46,62-68). These series suggested that lower prescription dose and tumor grade were the major predictors of recurrence following SRS (66,69). Kano et al. reported outcomes on 12 meningiomas (10 atypical and 2 anaplastic) with a median follow up of 43 months. Five year PFS was significantly higher for tumors treated with greater than 20 Gy compared with tumors treated with less than 20 Gy (P=0.0139) (64). By contrast, Stafford et al. found no dose correlation and reported a 5-year PFS of 68% with median SRS dose of 16 Gy (range, 12–36 Gy) and median follow-up of 47 months (46).
The role of SRS in the treatment of grade 3 meningioma is controversial (70). The largest series of SRS for patients with grade 3 meningioma reported the outcomes of 50 patients of which 40% patients had recurrent meningiomas (despite prior external beam radiation therapy to a median dose of 54.0 Gy). With a median follow up 38 months, the 5 years LC was 40% (71). Kondziolka et al. reported outcomes on 29 grade III meningioma patients managed with post-operative SRS. The mean SRS dose was 14 Gy and found PFS rates of 17% at 15 months (72).
In summary, for benign and non-benign tumours that are planned for SRS, the GTV is typically the enhancing tumor (Figure 2). There is typically no microscopic margin added to GTV and inclusion of the dural tail is controversial. The SRS doses generally range between 12–24 Gy delivered in a single fraction. While this has shown good LC for benign SBM, there is limited data on the outcomes of SRS for grade 2 and 3 SBMs.
Particle therapy
Emerging treatment opportunities with protons and other heavy particle beams have the ability to potentially improve dose sparing of normal tissues through the exploitation of the Bragg peak phenomenon, which results in an extremely steep dose fall-off at the end of the beam. At the present time, the ability to generate an accurate highly conformal proton radiotherapy plan is dependent on the treating team and institution due to variability in accurate modeling of the physical properties of proton therapy. Ongoing studies to better model the linear energy transfer and estimate the true radiobiological effective dose of proton therapy for integration of this information into treatment planning systems will greatly improve our ability to optimize use of this treatment modality (73-75).
Thus far, published outcomes reported for proton therapy have been comparable to photon radiotherapy. There are now outcomes published for over 200 patients treated with protons, but these studies have generally mixed treatments of photon and proton treatment, inclusion of all tumor grades and a range of clinical scenarios including primary treatment, adjuvant treatment and salvage therapy for recurrence (60-62,76-81). Noël et al. reported on fifty-one patients with SBM treated with proton and photon radiation to a median total dose was 60.6 CGE (range, 54–64 CGE) delivered with photons once daily, 5 days a week, to a median dose of 30.6 Gy (25–54 Gy) in 1.8–2 Gy fractions and the proton component to a median dose of 30 CGE (10–31 CGE) with 1.8–2 CGE fractions. Median tumor volume was 17 mL (1 to 120 mL), median tumor diameter of 52 mm (13 to 100 mm) and the CTV included the GTV plus 5–10 mm safety margin. With a mean follow up of 25.4 months, 4-year LC and OS rates were, 98% and 100%, respectively. No severe toxicity was reported (82). Wenkel et al. assessed PFS and toxicity of combined proton and photon radiation treatment for incompletely resected or recurrent meningioma. Patients were treated with a median of 25 (range, 8–34) fractions for protons in 1.92 CGE fractions and a median of 6 (range, 0–23) fractions for photons in 1.8 Gy fractions. Median tumor gross volume was 34 cc (range, 2–243 cc) and the CTV included the possible microscopic disease. With a median follow-up of 53 months, OS at 5 and 10 years was 93 and 77%, respectively, and PFS at 5 and 10 years was 100% and 88%, respectively. Eight patients developed severe long-term toxicity, ophthalmologic (4 patients), neurologic (4 patients), and otologic (2 patients) complications, which appeared to be dose related (receiving doses higher than those allowed) (80). Weber et al. reported the outcomes on 39 patients with histologically proven meningioma (34/39) treated with spot scanning proton radiotherapy to a median dose of 56.0 CGE (range, 52.2–66.6) at 1.8–2.0 CGE per fraction. Gross tumor volume ranged from 0.76 to 546.5 cc (median, 21.5) and CTV included the GTV plus regions of suspected microscopic spread (0–10 mm). With a mean follow-up time of 62.0 months, 5-year actuarial LC and OS rates were 84.8% and 81.8%, respectively, for the entire cohort (78).
Halasz et al. published on 50 patients treated with proton SRS [median dose 13 CGE (range, 10.0–15.5 Gy) (RBE) prescribed to the 90% isodose line] for benign meningioma. Median tumor volume was 2.1 cc (range, 0.3–9.7 cc). The median dose delivered was 13 CGE prescribed to the 90% isodose line. With a median follow-up of 32 months, 3-year LC rate was 94% and symptoms were improved in 47% (16/34) of patients (79). Gudjonsson et al. reported no signs of tumor progression after 36 months of follow-up following hypofractionated proton radiotherapy using doses between 14 Gy in 3 fractions to 24 Gy in 4 fractions, to the GTV plus 5 mm of margin, in 19 patients with partially-resected grade I (n=15) or unresectable (n=4) meningiomas (83).
There has been limited published data on heavy particle therapy. Combs et al. reported clinical outcome after a carbon ion boost in combination with high conformal photon radiation for high-risk meningioma, defined as atypical or anaplastic meningioma with ≥1 prior surgical resection. Carbon ion treatment was delivered with a median dose of 18 GyE, and photon radiation was applied with a median dose of 50.4 Gy. CTV including all macroscopic tumor, perifocal edema, as well as a safety margin of 2–3 cm. Median volume of the CTV was 217 mL With a median follow-up of 77 months and 10 patients treated in this phase 1/2 trial actuarial LC rates after primary radiotherapy were 86% and 72% at 5 and 7 years (84,85).
In summary particle therapy shows promising results in patients with SBM in terms of local tumor control and treatment-related toxicities. In addition, it has been proposed that data supports that protons are associated with a lower risk of secondary malignancies (86).
Cognitive functioning late toxicities in meningioma
With the advances on treatment modalities that enable high dose coverage of the tumor while sparing surrounding tissues, the potential capability to lower the treatment related side effects has become an important focus of research. Cognitive toxicity can impact meningioma patients substantially due to their long expected life expectancy. While this is recognized in the clinical community, there is a paucity of literature on changes in cognitive function and its impact on health related quality of life in this population (87-89).
A recent systematic review on cognitive function in meningioma patients prior to and/or following surgery with or without radiotherapy identified 1,012 potential articles, 11 that met inclusion criteria. This review highlighted the limitations of analyzing this data due to various methodological differences across studies (e.g., lack of pre-treatment assessment or standard neurocognitive tests). Nonetheless, this review reported that meningioma patients frequently present with cognitive issues in multiple domains prior to any treatment. Following treatment (surgery with or without radiotherapy), the majority of patients appeared to show improvement in their cognitive functioning, although continuing to have mild impairments when compared to healthy controls (90).
Suggestion for potential studies and research topics moving forward
With the growing appreciation for variability in tumor behaviour despite similar histological classification, there are strong efforts to discover novel driver mutations that may predict for tumor behaviour and may be a treatment target (91,92). Advances in imaging, such as the use of novel PET tracers or functional MRI, are presenting an opportunity to non-invasively interrogate the tumor and surrounding tissues to improve our treatment selection and provide treatment guidance. For instance, including improved tumor delineation for radiation treatment. This is of particular value to skull-base meningiomas, as surgery may not be offered as initial therapy and therefore tissue is not always collected for diagnostic confirmation or tumor characterization.
Acknowledgements
None.
Footnote
Conflicts of Interest: The authors have no conflicts of interest to declare.
References
- Oya S, Kim SH, Sade B, et al. The natural history of intracranial meningiomas. J Neurosurg 2011;114:1250-6. [Crossref] [PubMed]
- Whittle IR, Smith C, Navoo P, et al. Meningiomas. Lancet 2004;363:1535-43. [Crossref] [PubMed]
- Goto T, Ohata K. Surgical Resectability of Skull Base Meningiomas. Neurol Med Chir (Tokyo) 2016;56:372-8. [Crossref] [PubMed]
- Starke RM, Przybylowski CJ, Sugoto M, et al. Gamma Knife radiosurgery of large skull base meningiomas. J Neurosurg 2015;122:363-72. [Crossref] [PubMed]
- Maclean J, Fersht N, Short S. Controversies in Radiotherapy for Meningioma. Clin Oncol (R Coll Radiol) 2014;26:51-64. [Crossref] [PubMed]
- Bloch O, Kaur G, Jian BJ, et al. Stereotactic radiosurgery for benign meningiomas. J Neurooncol 2012;107:13-20. [Crossref] [PubMed]
- Milker-Zabel S, Bois AZ, Huber P, et al. Intensity-Modulated Radiotherapy for Complex-Shaped Meningioma of the Skull Base: Long-Term Experience of a Single Institution. Int J Radiat Oncol Biol Phys 2007;68:858-63. [Crossref] [PubMed]
- Compter I, Zaugg K, Houben RM, et al. High symptom improvement and local tumor control using stereotactic radiotherapy when given early after diagnosis of meningioma. Strahlenther Onkol 2012;188:887-93. [Crossref] [PubMed]
- Maclean J, Fersht N, Bremner F, et al. Meningioma Causing Visual Impairment: Outcomes and Toxicity After Intensity Modulated Radiation Therapy. Int J Radiat Oncol Biol Phys 2013;85:e179-86. [Crossref] [PubMed]
- Combs SE, Adeberg S, Dittmar JO, et al. Skull base meningiomas: Long-term results and patient self-reported outcome in 507 patients treated with fractionated stereotactic radiotherapy (FSRT) or intensity modulated radiotherapy (IMRT). Radiother Oncol 2013;106:186-91. [Crossref] [PubMed]
- Adeberg S, Welzel T, Rieken S, et al. Prior surgical intervention and tumor size impact clinical outcome after precision radiotherapy for the treatment of optic nerve sheath meningiomas (ONSM). Radiat Oncol 2011;6:117. [Crossref] [PubMed]
- Santacroce A, Walier M, Régis J, et al. Long-term tumor control of benign intracranial meningiomas after radiosurgery in a series of 4565 patients. Neurosurgery 2012;70:32-9; discussion 39. [Crossref] [PubMed]
- Klein EE, Hanley J, Bayouth J, et al. Task Group 142 report: quality assurance of medical accelerators. Med Phys 2009;36:4197-212. [Crossref] [PubMed]
- Low DA, Moran JM, Dempsey JF, et al. Dosimetry tools and techniques for IMRT. Med Phys 2011;38:1313-38. [Crossref] [PubMed]
- Marta GN, Correa SF, Teixeira MJ. Meningioma: review of the literature with emphasis on the approach to radiotherapy. Expert Rev Anticancer Ther 2011;11:1749-58. [Crossref] [PubMed]
- Debus J, Wuendrich M, Pirzkall A, et al. High efficacy of fractionated stereotactic radiotherapy of large base-of-skull meningiomas: long-term results. J Clin Oncol 2001;19:3547-53. [Crossref] [PubMed]
- Pirzkall A, Debus J, Haering P, et al. Intensity modulated radiotherapy (IMRT) for recurrent, residual, or untreated skull-base meningiomas: preliminary clinical experience. Int J Radiat Oncol Biol Phys 2003;55:362-72. [Crossref] [PubMed]
- Selch MT, Ahn E, Laskari A, et al. Stereotactic radiotherapy for treatment of cavernous sinus meningiomas. Int J Radiat Oncol Biol Phys 2004;59:101-11. [Crossref] [PubMed]
- Hamm K, Henzel M, Gross MW, et al. Radiosurgery/stereotactic radiotherapy in the therapeutical concept for skull base meningiomas. Zentralbl Neurochir 2008;69:14-21. [Crossref] [PubMed]
- Litré CF, Colin P, Noudel R, et al. Fractionated Stereotactic Radiotherapy Treatment of Cavernous Sinus Meningiomas: A Study of 100 Cases. Int J Radiat Oncol Biol Phys 2009;74:1012-7. [Crossref] [PubMed]
- Minniti G, Armosini V, Salvati M, et al. Fractionated stereotactic reirradiation and concurrent temozolomide in patients with recurrent glioblastoma. J Neurooncol 2011;103:683-91. [Crossref] [PubMed]
- Goldsmith BJ, Wara WM, Wilson CB, et al. Postoperative irradiation for subtotally resected meningiomas. A retrospective analysis of 140 patients treated from 1967 to 1990. J Neurosurg 1994;80:195-201. [Crossref] [PubMed]
- Aghi MK, Carter BS, Cosgrove GR, et al. Long-term recurrence rates of atypical meningiomas after gross total resection with or without postoperative adjuvant radiation. Neurosurgery 2009;64:56-60; discussion 60. [Crossref] [PubMed]
- Taylor BW, Marcus RB, Friedman WA, et al. The meningioma controversy: postoperative radiation therapy. Int J Radiat Oncol Biol Phys 1988;15:299-304. [Crossref] [PubMed]
- Olar A, Wani KM, Sulman EP, et al. Mitotic index is an independent predictor of recurrence-free survival in meningioma. Brain Pathol 2015;25:266-75. [Crossref] [PubMed]
- Kim YJ, Ketter R, Steudel WI, et al. Prognostic significance of the mitotic index using the mitosis marker anti-phosphohistone H3 in meningiomas. Am J Clin Pathol 2007;128:118-25. [Crossref] [PubMed]
- Dziuk TW, Woo S, Butler EB, et al. Malignant meningioma: an indication for initial aggressive surgery and adjuvant radiotherapy. J Neurooncol 1998;37:177-88. [Crossref] [PubMed]
- Engenhart-Cabillic R, Farhoud A, Sure U, et al. Clinicopathologic features of aggressive meningioma emphasizing the role of radiotherapy in treatment. Strahlenther Onkol 2006;182:641-6. [Crossref] [PubMed]
- Stafford SL, Perry A, Suman VJ, et al. Primarily resected meningiomas: outcome and prognostic factors in 581 Mayo Clinic patients, 1978 through 1988. Mayo Clin Proc 1998;73:936-42. [Crossref] [PubMed]
- Kaur G, Sayegh ET, Larson A, et al. Adjuvant radiotherapy for atypical and malignant meningiomas: a systematic review. Neuro Oncol 2014;16:628-36. [Crossref] [PubMed]
- Yang SY, Park CK, Park S-H, et al. Atypical and anaplastic meningiomas: prognostic implications of clinicopathological features. J Neurol Neurosurg Psychiatry 2008;79:574-80. [Crossref] [PubMed]
- Adeberg S, Hartmann C, Welzel T, et al. Long-term outcome after radiotherapy in patients with atypical and malignant meningiomas--clinical results in 85 patients treated in a single institution leading to optimized guidelines for early radiation therapy. Int J Radiat Oncol Biol Phys 2012;83:859-64. [Crossref] [PubMed]
- Stessin AM, Schwartz A, Judanin G, et al. Does adjuvant external-beam radiotherapy improve outcomes for nonbenign meningiomas? A Surveillance, Epidemiology, and End Results (SEER)-based analysis. J Neurosurg 2012;117:669-75. [Crossref] [PubMed]
- Mair R, Morris K, Scott I, et al. Radiotherapy for atypical meningiomas. J Neurosurg 2011;115:811-9. [Crossref] [PubMed]
- Mirimanoff RO, Dosoretz DE, Linggood RM, et al. Meningioma: analysis of recurrence and progression following neurosurgical resection. J Neurosurg 1985;62:18-24. [Crossref] [PubMed]
- Condra KS, Buatti JM, Mendenhall WM, et al. Benign meningiomas: primary treatment selection affects survival. Int J Radiat Oncol Biol Phys 1997;39:427-36. [Crossref] [PubMed]
- Soyuer S, Chang EL, Selek U, et al. Radiotherapy after surgery for benign cerebral meningioma. Radiother Oncol 2004;71:85-90. [Crossref] [PubMed]
- Milosevic MF, Frost PJ, Laperriere NJ, et al. Radiotherapy for atypical or malignant intracranial meningioma. Int J Radiat Oncol Biol Phys 1996;34:817-22. [Crossref] [PubMed]
- DeVries A, Munzenrider JE, Hedley-Whyte T, et al. The role of radiotherapy in the treatment of malignant meningiomas. Strahlenther Onkol 1999;175:62-7. [Crossref] [PubMed]
- Hug EB, Devries A, Thornton AF, et al. Management of atypical and malignant meningiomas: role of high-dose, 3D-conformal radiation therapy. J Neurooncol 2000;48:151-60. [Crossref] [PubMed]
- Boskos C, Feuvret L, Noel G, et al. Combined Proton and Photon Conformal Radiotherapy for Intracranial Atypical and Malignant Meningioma. Int J Radiat Oncol Biol Phys 2009;75:399-406. [Crossref] [PubMed]
- Katz TS, Amdur RJ, Yachnis AT, et al. Pushing the limits of radiotherapy for atypical and malignant meningioma. Am J Clin Oncol 2005;28:70-4. [Crossref] [PubMed]
- Rogers L, Barani I, Chamberlain M, et al. Meningiomas: knowledge base, treatment outcomes, and uncertainties. A RANO review. J Neurosurg 2015;122:4-23. [Crossref] [PubMed]
- Morita A, Coffey RJ, Foote RL, et al. Risk of injury to cranial nerves after gamma knife radiosurgery for skull base meningiomas: experience in 88 patients. J Neurosurg 1999;90:42-9. [Crossref] [PubMed]
- Roche PH, Régis J, Dufour H, et al. Gamma knife radiosurgery in the management of cavernous sinus meningiomas. J Neurosurg 2000;93 Suppl 3:68-73. [PubMed]
- Stafford SL, Pollock BE, Foote RL, et al. Meningioma radiosurgery: tumor control, outcomes, and complications among 190 consecutive patients. Neurosurgery 2001;49:1029-37; discussion 1037-8. [PubMed]
- Nicolato A, Foroni R, Alessandrini F, et al. Radiosurgical treatment of cavernous sinus meningiomas: experience with 122 treated patients. Neurosurgery 2002;51:1153-9; discussion 1159-61. [Crossref] [PubMed]
- Kollová A, Liscák R, Novotný J Jr, et al. Knife surgery for benign meningioma. J Neurosurg 2007;107:325-36. [Crossref] [PubMed]
- Feigl GC, Samii M, Horstmann GA. Volumetric follow-up of meningiomas: a quantitative method to evaluate treatment outcome of gamma knife radiosurgery. Neurosurgery 2007;61:281-6; discussion 286-7. [Crossref] [PubMed]
- Chuang CC, Chang CN, Tsang NM, et al. Linear accelerator-based radiosurgery in the management of skull base meningiomas. J Neurooncol 2004;66:241-9. [Crossref] [PubMed]
- Dos Santos MA, de Salcedo JB, Gutiérrez Diaz JA, et al. Long-Term Outcomes of Stereotactic Radiosurgery for Treatment of Cavernous Sinus Meningiomas. Int J Radiat Oncol Biol Phys 2011;81:1436-41. [Crossref] [PubMed]
- Unger KR, Lominska CE, Chanyasulkit J, et al. Risk factors for posttreatment edema in patients treated with stereotactic radiosurgery for meningiomas. Neurosurgery 2012;70:639-45. [Crossref] [PubMed]
- Eustacchio S, Trummer M, Fuchs I, et al. Preservation of cranial nerve function following Gamma Knife radiosurgery for benign skull base meningiomas: experience in 121 patients with follow-up of 5 to 9.8 years. Acta Neurochir Suppl 2002;84:71-6. [PubMed]
- Roche P-H, Pellet W, Fuentes S, et al. Gamma knife radiosurgical management of petroclival meningiomas results and indications. Acta Neurochir (Wien) 2003;145:883-8; discussion 888. [Crossref] [PubMed]
- Kreil W, Luggin J, Fuchs I, et al. Long term experience of gamma knife radiosurgery for benign skull base meningiomas. J Neurol Neurosurg Psychiatry 2005;76:1425-30. [Crossref] [PubMed]
- Zachenhofer I, Wolfsberger S, Aichholzer M, et al. Gamma-knife radiosurgery for cranial base meningiomas: experience of tumor control, clinical course, and morbidity in a follow-up of more than 8 years. Neurosurgery 2006;58:28-36; discussion 28-36. [Crossref] [PubMed]
- Davidson L, Fishback D, Russin JJ, et al. Postoperative Gamma Knife surgery for benign meningiomas of the cranial base. Neurosurg Focus 2007;23:E6. [Crossref] [PubMed]
- Han JH, Kim DG, Chung HT, et al. Gamma knife radiosurgery for skull base meningiomas: long-term radiologic and clinical outcome. Int J Radiat Oncol Biol Phys 2008;72:1324-32. [Crossref] [PubMed]
- Iwai Y, Yamanaka K, Ikeda H. Gamma Knife radiosurgery for skull base meningioma: long-term results of low-dose treatment. J Neurosurg 2008;109:804-10. [Crossref] [PubMed]
- Hayashi M, Chernov M, Tamura N, et al. Gamma knife robotic microradiosurgery for benign skull base meningiomas: tumor shrinkage may depend on the amount of radiation energy delivered per lesion volume (unit energy). Stereotact Funct Neurosurg 2011;89:6-16. [Crossref] [PubMed]
- Starke RM, Williams BJ, Hiles C, et al. Gamma knife surgery for skull base meningiomas. J Neurosurg 2012;116:588-97. [Crossref] [PubMed]
- Harris AE, Lee JY, Omalu B, et al. The effect of radiosurgery during management of aggressive meningiomas. Surg Neurol 2003;60:298-305; discussion 305. [Crossref] [PubMed]
- Huffmann BC, Reinacher PC, Gilsbach JM. Gamma knife surgery for atypical meningiomas. J Neurosurg 2005;102 Suppl:283-6. [Crossref]
- Kano H, Takahashi JA, Katsuki T, et al. Stereotactic radiosurgery for atypical and anaplastic meningiomas. J Neurooncol 2007;84:41-7. [Crossref] [PubMed]
- Attia A, Chan M, Seif D, et al. Treatment of Atypical Meningiomas with Gamma Knife Radiosurgery: The Role of Conformality Index and Margin Dose. Int J Radiat Oncol Biol Phys 2009;75:S226-S227. [Crossref]
- Skeie BS, Enger PO, Skeie GO, et al. Gamma knife surgery of meningiomas involving the cavernous sinus: long-term follow-up of 100 patients. Neurosurgery 2010;66:661-8; discussion 668-9. [Crossref] [PubMed]
- Choi CY, Soltys SG, Gibbs IC, et al. Cyberknife stereotactic radiosurgery for treatment of atypical (WHO grade II) cranial meningiomas. Neurosurgery 2010;67:1180-8. [Crossref] [PubMed]
- Hardesty DA, Wolf AB, Brachman DG, et al. The impact of adjuvant stereotactic radiosurgery on atypical meningioma recurrence following aggressive microsurgical resection. J Neurosurg 2013;119:475-81. [Crossref] [PubMed]
- Kano H, Kondziolka D, Khan A, et al. Predictors of hearing preservation after stereotactic radiosurgery for acoustic neuroma: clinical article. J Neurosurg 2013;119 Suppl:863-73. [PubMed]
- Modha A, Gutin PH. Diagnosis and treatment of atypical and anaplastic meningiomas: a review. Neurosurgery 2005;57:538-50; discussion 538-50. [Crossref] [PubMed]
- Pollock BE, Stafford SL, Link MJ, et al. Stereotactic radiosurgery of World Health Organization grade II and III intracranial meningiomas: treatment results on the basis of a 22-year experience. Cancer 2012;118:1048-54. [Crossref] [PubMed]
- Kondziolka D, Mathieu D, Lunsford LD, et al. Radiosurgery as definitive management of intracranial meningiomas. Neurosurgery 2008;62:53-8; discussion 58-60. [Crossref] [PubMed]
- Mohan R, Mahajan A, Minsky BD. New Strategies in Radiation Therapy: Exploiting the Full Potential of Protons. Clin Cancer Res 2013;19:6338-43. [Crossref] [PubMed]
- Smith A, Gillin M, Bues M, et al. The M. D. Anderson proton therapy system. Med Phys 2009;36:4068-83. [Crossref] [PubMed]
- Tommasino F, Durante M. Proton Radiobiology. Cancers 2015;7:353-81. [Crossref] [PubMed]
- Coke CC, Corn BW, Werner-Wasik M, et al. Atypical and malignant meningiomas: an outcome report of seventeen cases. J Neurooncol 1998;39:65-70. [Crossref] [PubMed]
- Chan AW, Bernstein KD, Adams JA, et al. Dose escalation with proton radiation therapy for high-grade meningiomas. Technol Cancer Res Treat 2012;11:607-14. [Crossref] [PubMed]
- Weber DC, Schneider R, Goitein G, et al. Spot Scanning-Based Proton Therapy for Intracranial Meningioma: Long-Term Results From the Paul Scherrer Institute. Int J Radiat Oncol Biol Phys 2012;83:865-71. [Crossref] [PubMed]
- Halasz LM, Bussière MR, Dennis ER, et al. Proton Stereotactic Radiosurgery for the Treatment of Benign Meningiomas. Int J Radiat Oncol Biol Phys 2011;81:1428-35. [Crossref] [PubMed]
- Wenkel E, Thornton AF, Finkelstein D, et al. Benign meningioma: Partially resected, biopsied, and recurrent intracranial tumors treated with combined proton and photon radiotherapy. Int J Radiat Oncol Biol Phys 2000;48:1363-70. [Crossref] [PubMed]
- Vernimmen FJ, Harris JK, Wilson JA, et al. Stereotactic proton beam therapy of skull base meningiomas. Int J Radiat Oncol Biol Phys 2001;49:99-105. [Crossref] [PubMed]
- Noël G, Bollet MA, Calugaru V, et al. Functional outcome of patients with benign meningioma treated by 3D conformal irradiation with a combination of photons and protons. Int J Radiat Oncol 2005;62:1412-22. [Crossref] [PubMed]
- Gudjonsson O, Blomquist E, Nyberg G, et al. Stereotactic irradiation of skull base meningiomas with high energy protons. Acta Neurochir (Wien) 1999;141:933-40. [Crossref] [PubMed]
- Combs SE, Burkholder I, Edler L, et al. Randomised phase I/II study to evaluate carbon ion radiotherapy versus fractionated stereotactic radiotherapy in patients with recurrent or progressive gliomas: the CINDERELLA trial. BMC Cancer 2010;10:533. [Crossref] [PubMed]
- Combs SE, Hartmann C, Nikoghosyan A, et al. Carbon ion radiation therapy for high-risk meningiomas. Radiother Oncol 2010;95:54-9. [Crossref] [PubMed]
- Arvold ND, Niemierko A, Broussard GP, et al. Projected Second Tumor Risk and Dose to Neurocognitive Structures After Proton Versus Photon Radiotherapy for Benign Meningioma. Int J Radiat Oncol Biol Phys 2012;83:e495-500. [Crossref] [PubMed]
- Weitzner MA, Meyers CA. Cognitive functioning and quality of life in malignant glioma patients: a review of the literature. Psychooncology 1997;6:169-77. [Crossref] [PubMed]
- Van Nieuwenhuizen D, Ambachtsheer N, Heimans JJ, et al. Neurocognitive functioning and health-related quality of life in patients with radiologically suspected meningiomas. J Neurooncol 2013;113:433-40. [Crossref] [PubMed]
- Noll KR, Sullaway C, Ziu M, et al. Relationships between tumor grade and neurocognitive functioning in patients with glioma of the left temporal lobe prior to surgical resection. Neuro Oncol 2015;17:580-7. [Crossref] [PubMed]
- Meskal I, Gehring K, Rutten GJ, et al. Cognitive functioning in meningioma patients: a systematic review. J Neurooncol 2016;128:195-205. [Crossref] [PubMed]
- Louis DN, Perry A, Reifenberger G, et al. The 2016 World Health Organization Classification of Tumors of the Central Nervous System: a summary. Acta Neuropathol (Berl) 2016;131:803-20. [Crossref] [PubMed]
- Goldbrunner R, Minniti G, Preusser M, et al. EANO guidelines for the diagnosis and treatment of meningiomas. Lancet Oncol 2016;17:e383-91. [Crossref] [PubMed]