Re-irradiation for recurrent glioblastoma multiforme
Introduction
The use of radiotherapy (RT) is standard in the management of newly diagnosed glioblastoma multiforme (GBM), whether in the postoperative or primary treatment setting, with temozolomide (TMZ) typically given during and after RT. Unfortunately, the large majority of patients develop intracranial recurrence, most often within or just outside the high-dose radiation field (1,2). Treatment options in the setting of local recurrence include supportive care, re-resection, re-irradiation, systemic therapies, or a combination approach.
Diagnosis of recurrence
Radionecrosis (RN) and recurrent tumor are often indistinguishable by magnetic resonance imaging (MRI) and present with similar neurological symptoms, making the diagnosis of local recurrence in GBM difficult. A combination of diffusion- and perfusion-weighted MRI can improve diagnostic accuracy by exploiting differences in tissue cellularity and microvasculature respectively (3). For example, a low apparent diffusion coefficient in a hyperintense lesion is characteristic of tumor recurrence whereas low cerebral blood flow and volume are characteristic of RN. Additional functional imaging including magnetic resonance spectroscopy (MRS), single photon emission computed tomography (SPECT) or positron emission tomography (PET) can help better characterize the biology of the lesions, though each of these modalities has their limitations.
MRS characterizes tissues using ratios of choline (Ch), creatinine (Cr) and N-acetylaspartate (NAA); high Ch/NAA (>1.11) and Ch/Cr (>1.17) ratios and a low NAA/Cr ratio are typically seen in tumor recurrence. Results for MRS are wide ranging, with reported sensitivities and specificities of 61–94% and 82–100%, respectively (4-7). These inconsistent results are largely due to differences in spectroscopy, a factor that should be considered during clinical decision making. For instance, single-voxel MRS only samples at one location, possibly mischaracterizing a heterogeneous entity such as recurrent GBM, whereas multivoxel MRS obtains samples throughout a lesion, better defining spatial heterogeneity (7).
Tracer dependent studies (SPECT, PET) are mostly limited by poor spatial resolution, steroid use, and significant normal tissue tracer uptake (8). Fluorodeoxyglucose (FDG)-PET in particular displays this issue given the elevated glucose metabolism in normal brain tissues. A search continues for highly sensitive and specific alternatives to FDG that do not accumulate within the normal brain parenchyma. Some promising candidates remain under investigation such as fluoroethyltyrosine (FET), fluorothymidine (FLT), fluoro-dihydroxyphenylalanine (FDOPA) and other amino acid analogues; however, none are widely available to date.
The Response Assessment in Neuro-Oncology criteria are the current standard for treatment response assessment and definition of tumor recurrence used for the majority of clinical trials. The application of these criteria have been previously described (9).
Summary of non-RT options for recurrent glioblastoma
There is minimal data from randomised controlled in the treatment of recurrent GBM. As mentioned, treatment options for recurrence have typically included further surgery, systemic therapy, and more recently re-irradiation, but currently, there is no established standard of care.
Further neurosurgical intervention may be limited by the infiltrative nature of these tumors, and is usually avoided in the presence of multifocal disease or when eloquent tissues are involved. Novel resection techniques are also being explored to improve the rate of complete resection. For example, protoporphyrin IX (PpIX) is a fluorescent compound that preferentially concentrates in malignant glioma cells, allowing for successful intra-operative tumor visualization to guide surgical resection (10,11). Despite such advances, the principle of maximum safe resection is not always optimal in the setting of recurrent GBM, as the benefit of reoperation remains in question (12-14). In general, surgery seems to be most beneficial when there is a discrete, well-defined lesion in a non-eloquent location and resection is expected to relieve symptomatic mass effect. Placement of resection cavity carmustine wafers can afford a modest improvement in survival as well (15). Repeat resection should be offered in the setting of discrete, resectable disease.
A comprehensive review of all trials investigating different systemic therapies for the treatment of recurrent GBM is beyond the scope of this review, however, a brief history and important recent results will be discussed to give context to re-irradiation decision making. In 1998 Huncharek and Muscat authored a systematic review of 40 trials evaluating earlier outcomes in recurrent high-grade gliomas; seven were RT trials and the remainder addressed cytotoxic chemotherapy outcomes (16). In their review, nitrosoureas were associated with significantly improved time to progression (26.9 weeks), with the use of nitrosoureas or platinum agents found to improve overall survival (OS) as well (32 weeks). Average median survival for patients receiving re-irradiation was 44.7 weeks. Comparisons were not made between chemotherapy and RT studies given the inherent selection bias. Since then, more systemic agents have emerged, the most studied of which are TMZ and bevacizumab. No single or combination drug therapy has shown obvious survival superiority, thus, there is no standard regimen for GBM in the recurrent setting. Although, bevacizumab is most often utilized due to improvement in progression free survival (PFS) and its anti-steroidal effects improve symptoms in many patients.
The biology of late neurotoxicity
When considering treatment options for recurrent glioblastoma, one must balance the efficacy with the toxicity of each option, especially in view of the relatively poor prognosis. Three phases of toxicity are normally considered following central nervous system (CNS) irradiation—early (days to weeks), early delayed (1 to 6 months) and late (>6 months). Early toxicity is often self-limited or managed conservatively, but late toxicity is typically progressive and irreversible.
Demyelination, microvascular changes, and necrosis are the pathologic hallmarks of late injury. This injury is considered a multifaceted process, involving various cell types and interactions. Given this complexity, efforts to devise effective preventive or treatment strategies have been unsuccessful to date (17,18). However, conservative estimates based on animal and other preclinical data suggest that the spinal cord and perhaps other CNS normal tissues may recover up to 60% from sub-tolerance doses over 1–3 years (19). Normal tissue complication models in rats have also suggested that the CNS behaves as a serial structure (20). As such, the toxicity of re-irradiation is likely also dependent on volume irradiated, in addition to dose and time interval to re-treatment. Modern re-irradiation techniques are capable of optimizing these parameters so as to reduce the risk of clinically apparent late toxicity.
Patient selection for re-irradiation
Individual patient and tumor characteristics should be used to estimate prognosis and tailor management in the recurrent setting. In 2007 Carson et al. (21) defined seven prognostic groups in patients with recurrent high-grade glioma by performing recursive partitioning analysis (RPA) of data from ten phase I and II trials. Significant prognostic factors for recurrent GBM were age (>50), Karnofsky performance status (KPS >90 vs. 60–80), and baseline steroid requirement. A similar study reviewing data from 300 patients with recurrent GBM recruited in eight phase I or II trials conducted by the European Organization for Research and Treatment of Cancer (EORTC) showed poor performance status (PS) and >1 target lesion were prognostic for decreased PFS and OS. Lesions ≥4.2 cm and baseline steroid requirement were also associated with shorter OS whereas frontal tumor locations conferred a survival advantage (12). In patients undergoing fractionated stereotactic radiation (FSRT) for re-irradiation of high-grade gliomas, Combs et al. showed that a time to re-irradiation <12 months negatively impacted survival (22). A combination of these data can help individualize the potential benefits of re-irradiation and guide patient counselling.
The decision for retreatment should only be made when the risks are outweighed by potential benefits of treatment. High-dose brain irradiation can have a number of side effects that can substantially impact quality of life (QOL) including focal neurologic deficits, seizure, memory and/or cognitive impairment, and personality change. In the event of RN, reoperation is often necessary. Due to a paucity of data addressing QOL in GBM retreatment, extrapolations from primary treatment of GBM can be made. Prospective trials in primary treatment of GBM have correlated poor post-treatment QOL and symptom burden with decreased rates of survival (23,24). This data further underscores the importance of patient selection for re-irradiation.
Evidence for re-irradiation
Numerous institutions have reported their re-irradiation disease control and toxicity outcomes (Table 1). Almost all reports are retrospective in nature and as such there is little consistency in treatment technique, total dose, and volume treated. These differences make it difficult to establish a standard approach to re-irradiation. Regardless of the methods used, prognosis remains poor with median survival ranging from 7–15 months (Table 1).
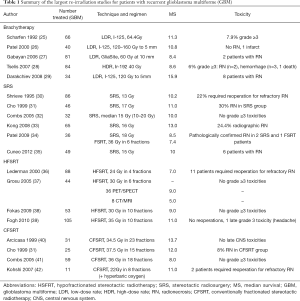
Full table
Conventionally fractionated radiotherapy
In multifocal, diffuse, or unusually large recurrent tumors, a more generous treatment volume may be required. In contrast to stereotactic radiosurgery (SRS) or hypofractionated stereotactic RT (HFSRT), the smaller fraction sizes associated with conventional fraction may allow for treating larger target volumes while still maintaining acceptable rates of toxicity. For example, with a median time between primary RT (median 60 Gy) of 10 months, Combs et al. retreated 172 high-grade gliomas (GBM =59) to a median dose of 36 Gy in 2 Gy daily fractions using 0.5–1 cm margin. Median survival after re-irradiation for the GBM group was 8 months and only one patient developed RN.
SRS and HFSRT
When recurrences are discrete and retreatment volumes small, HFSRT and SRS can be considered. These methods offer certain technical and dosimetric advantages compared to conventional fractionation, while also affording the patient-friendly benefit of consolidating re-irradiation into fewer days.
The high accuracy and conformality associated with SRS allows for delivering a high dose of radiation to a brain lesion in a single outpatient treatment. The steep SRS dose gradient is best suited for discrete recurrences or when there are nearby critical CNS structures. In FSRT, the dose is divided into several semi high–dose fractions; this offers the radiobiologic advantage of allowing normal tissues to heal between fractions, thus reducing the potential for normal tissue toxicity compared to single fraction treatment. As such, this technique is often useful when the target volume is felt to be too large for SRS, or when single fraction normal tissue constraints cannot be met because the target is located too close to a critical structure.
Both SRS and FSRT have been shown to be effective with similar rates of toxicity (Table 1), as such there is currently no standardized preference for one over the other in the recurrent GBM setting. In a more recent study, Patel et al. treated 38 GBM patients with either SRS (single 18 Gy fraction) or FSRT (36 Gy in 6 Gy fractions) with reported median survivals of 8.5 and 7.4 months respectively and a radiological response rate of 40%. Pathologically confirmed RN was found in 2 SRS and 1 FSRT patients (34). The National Cancer Institute has an ongoing HFSRT dose escalation trial (NCT02709226) which should provide much needed re-irradiation dosimetric and toxicity data that can serve as a framework for future clinical trial design.
Treatment fields
Gadolinium enhanced, thin-sliced MRI remains the standard imaging modality for target definition. New or progressive contrast-enhancing lesions should be delineated as gross tumor volume. The use of a rigid head frame and same-day imaging for target delineation can minimize geometric and image fusion error, thereby reducing the need for PTV expansion. When applying these principles for SRS or HFSRT, elimination of PTV expansion can be considered; however, a small 1–2 mm margin may be added based on physician preferences. Ideally, adequate head immobilization should be used to where PTV expansion is at most 5 mm.
Brachytherapy
Similar to SRS or FSRT, brachytherapy allows for a sharp dose gradient with placement of a radiation source within the treatment volume. This is typically done in the postoperative setting, and, as such, patients selected for brachytherapy are usually those with good PS who have small-volume, resectable tumors. Different approaches include placement of permanent iodine 125 (I-125) seeds or a temporary intracranial balloon catheter filled with an I-125 containing solution in the resection cavity. A number of retrospective studies have reported favorable outcomes with median survival times of 8.4 to 15.9 months (Table 1). It should be noted, however, that comparisons to other re-irradiation modalities should be made with caution given the selection biases mentioned above. One disadvantage of brachytherapy is that the effectiveness and toxicity of treatment is highly dependent on the quality of implant; accordingly, high re-operation rates and RN incidence have been reported (Table 1).
Conclusions based on clinical evidence
Considering the variety of techniques and disease/toxicity outcomes discussed above, it is no wonder that despite a large body of clinical work, no standard practice exists for re-irradiation of GBM. Prospective data and clinical trials are needed to eliminate the many confounding factors inherent in retrospective studies. Despite these limitations, the existing data suggests that with thoughtful patient selection, re-irradiation can be a safe and effective treatment for recurrent GBM.
Re-irradiation fields should be highly conformal and target volumes minimized in an effort to reduce late side effects. A general guideline would be to keep volumes <4–5 cm and cumulative dose <100 Gy to prevent high rates of toxicity (43). Total dose and dose per fraction can be reduced as needed to compensate for an oversized re-treatment volume. The most commonly employed regimens are 35 Gy in 10 fractions and 24–36 Gy in 4–6 fractions.
The patient’s PS and effect of re-irradiation on QOL should be weighed, as should the impact of a drawn out treatment course in the setting of poor prognosis.
Combination treatments and future directions
The inclusion of TMZ to re-irradiation has been well-studied and shown to be safe and effective with median survival ranging from 5.1 to 10.1 months after combination therapy (44-47). Darakchiev et al. placed Gliadel wafers in the resection cavity following surgical resection and I-125 seed implants in 34 patients with recurrent GBM. Median survival was 15.9 months, however, RN was observed in 24% of cases when tumor volume was >30 cm3 (29). Bevacizumab in combination with radiation retreatment has also been evaluated in a number of prospective studies. These studies have reported median survival ranging from 7.4 to 18 months with acceptable rates of toxicity (35,48-51). Of note, there are case reports of bevacizumab reversing radiation-induced necrosis and (52,53) in a small (n=14) randomized crossover study, all bevacizumab-treated patients (n=13 after crossover) developed improvement in neurologic signs and symptoms (54). The NRG 1205 trial randomizes patients to bevacizumab alone vs. HFSRT (35 Gy in 10 fractions) with bevacizumab (NCT02671981); it completed accrual in 2016, and we await the results. Given their success in preclinical glioma models and other solid tumors, immune modulators such as nivolumab, ipilimumab, and pembrolizumab are also being evaluated in combination with re-irradiation. Nivolumab and pembrolizumab are both PD-1 inhibitors and ipilimumab targets CTLA-4. The results of a phase I evaluation of combination nivolumab and ipilimumab in recurrent glioblastoma (CHECKMATE-143 trial) were recently updated at the American Society of Clinical Oncology 2016 annual meeting, showing that the combination is relatively safe with no treatment-related deaths. In addition, cohort 1 with nivolumab alone had no grade 3–5 toxicity, however the combination arms of nivolumab 1 mg/kg and ipilimumab 3 mg/kg had 9 (90%) of patients experienced grade 3–4 treatment related toxicity and 7 (70%) had serious treatment related toxicity. The combination of nivolumab 3 mg/kg and ipilimumab 1 mg/kg had 5 (25%) grade 3–4 toxicity with 2 (10%) serious treatment related toxicity. The 12 month OS was 40% for the nivolumab 3 mg/kg alone, 30% for the nivolumab 1 mg/kg plus ipilimumab 3 mg/kg and 25% for the nivolumab 3 mg/kg plus ipilimumab 1 mg/kg (55). There are additional ongoing safety and efficacy immunotherapy studies, such as NRG-BN002, which introduces ipilimumab and nivolumab into maintenance TMZ therapy, or another study which combines HSFRT re-irradiation with pembrolizumab and bevacizumab (NCT02313272). Promising preclinical data has also shown that glioma cell growth can be inhibited through changes in diet. These effects are amplified with the addition of RT and together may increase longevity of life. More specifically, a ketogenic low-calorie diet is recommended and has been shown to be safe and feasible during primary chemoradiation and in the recurrent setting. However, poor compliance related to palatability of such a diet could be a major drawback to implementation in the clinical setting. A less strict variant, high-fat, low-carbohydrate diet may be a more practical alternative with similar beneficial biological effects. Clinical trials assessing the integration of dietary manipulation with GBM re-irradiation are under way (NCT02149459, ERGO2-NCT01754350). Synergistic alternative therapies can only help in widening the window of application for re-irradiation in recurrent GBM, especially if/when the aforementioned novel combination therapies produce improved clinical outcomes.
Conclusions
There are myriad treatment options available to patients with recurrent glioblastoma, including repeat surgery, systemic therapy, and re-irradiation, or some combination thereof. Re-irradiation appears to be beneficial in a subgroup of patients. The data presented in this review shows re-irradiation to be safe in a well-selected group of patients, although there are certainly limitations in the available literature. The impact of re-irradiation on QOL is not well documented and will be an important component of future prospective studies. The combination of re-irradiation with novel systemic agents is a promising future direction in this patient population, which warrants prospective study in clinical trials.
Acknowledgements
None.
Footnote
Conflicts of Interest: The authors have no conflicts of interest to declare.
References
- Hochberg FH, Pruitt A. Assumptions in the radiotherapy of glioblastoma. Neurology 1980;30:907-11. [Crossref] [PubMed]
- Wallner KE, Galicich JH, Krol G, et al. Patterns of failure following treatment for glioblastoma multiforme and anaplastic astrocytoma. Int J Radiat Oncol Biol Phys 1989;16:1405-9. [Crossref] [PubMed]
- Kim HS, Goh MJ, Kim N, et al. Which Combination of MR Imaging Modalities Is Best for Predicting Recurrent Glioblastoma? Study of Diagnostic Accuracy and Reproducibility. Radiology 2014;273:831-43. [Crossref] [PubMed]
- Amin A, Moustafa H, Ahmed E, et al. Glioma residual or recurrence versus radiation necrosis: accuracy of pentavalent technetium-99m-dimercaptosuccinic acid [Tc-99m (V) DMSA] brain SPECT compared to proton magnetic resonance spectroscopy (1H-MRS): initial results. J Neurooncol 2012;106:579-87. [Crossref] [PubMed]
- Lichy MP, Henze M, Plathow C, et al. Metabolic imaging to follow stereotactic radiation of gliomas -- the role of 1H MR spectroscopy in comparison to FDG-PET and IMT-SPECT. ROFO. Fortschr. Geb. Rontgenstr. Nuklearmed 2004;176:1114-21. [Crossref] [PubMed]
- Träber F, Block W, Flacke S, et al. 1H-MR Spectroscopy of brain tumors in the course of radiation therapy: Use of fast spectroscopic imaging and single-voxel spectroscopy for diagnosing recurrence. Rofo 2002;174:33-42. [PubMed]
- Zeng QS, Li CF, Zhang K, et al. Multivoxel 3D proton MR spectroscopy in the distinction of recurrent glioma from radiation injury. J Neurooncol 2007;84:63-9. [Crossref] [PubMed]
- Herholz K, Coope D, Jackson A. Metabolic and molecular imaging in neuro-oncology. Lancet Neurol 2007;6:711-24. [Crossref] [PubMed]
- Vogelbaum MA, Jost S, Aghi MK, et al. Application of Novel Response/Progression Measures for Surgically Delivered Therapies for Gliomas: Response Assessment in Neuro-Oncology (RANO) Working Group. Neurosurgery 2012;70:234-43. [Crossref] [PubMed]
- Nguyen QT, Tsien RY. Fluorescence-guided surgery with live molecular navigation — a new cutting edge. Nat Rev Cancer 2013;13:653-62. [Crossref] [PubMed]
- Quick-Weller J, Lescher S, Forster MT, et al. Combination of 5-ALA and iMRI in re-resection of recurrent glioblastoma. Br J Neurosurg 2016;30:313-7. [Crossref] [PubMed]
- Gorlia T, Stupp R, Brandes AA, et al. New prognostic factors and calculators for outcome prediction in patients with recurrent glioblastoma: A pooled analysis of EORTC Brain Tumour Group phase I and II clinical trials. Eur J Cancer 2012;48:1176-84. [Crossref] [PubMed]
- Brandes AA, Bartolotti M, Franceschi E. Second surgery for recurrent glioblastoma: advantages and pitfalls. Expert Rev Anticancer Ther 2013;13:583-7. [Crossref] [PubMed]
- Palmer JD, Siglin J, Yamoah K, et al. Re-resection for recurrent high-grade glioma in the setting of re-irradiation: more is not always better. J Neurooncol 2015;124:215-21. [Crossref] [PubMed]
- Brem H, Piantadosi S, Burger PC, et al. Placebo-controlled trial of safety and efficacy of intraoperative controlled delivery by biodegradable polymers of chemotherapy for recurrent gliomas. The Polymer-brain Tumor Treatment Group. Lancet 1995;345:1008-12. [Crossref] [PubMed]
- Huncharek M, Muscat J. Treatment of recurrent high grade astrocytoma; results of a systematic review of 1,415 patients. Anticancer Res 1998;18:1303-11. [PubMed]
- Tofilon PJ, Fike JR. The radioresponse of the central nervous system: a dynamic process. Radiat Res 2000;153:357-70. [Crossref] [PubMed]
- Wong CS, Van der Kogel AJ. Mechanisms of radiation injury to the central nervous system: implications for neuroprotection. Mol Interv 2004;4:273-84. [Crossref] [PubMed]
- Ang KK, Jiang GL, Feng Y, et al. Extent and kinetics of recovery of occult spinal cord injury. Int J Radiat Oncol Biol Phys 2001;50:1013-20. [Crossref] [PubMed]
- Philippens ME, Pop LA, Visser AG, et al. Dose-volume effects in rat thoracolumbar spinal cord: an evaluation of NTCP models. Int J Radiat Oncol Biol Phys 2004;60:578-90. [Crossref] [PubMed]
- Carson KA, Grossman SA, Fisher JD, et al. Prognostic Factors for Survival in Adult Patients With Recurrent Glioma Enrolled Onto the New Approaches to Brain Tumor Therapy CNS Consortium Phase I and II Clinical Trials. J Clin Oncol 2007;25:2601-6. [Crossref] [PubMed]
- Combs SE, Edler L, Rausch R, et al. Generation and validation of a prognostic score to predict outcome after re-irradiation of recurrent glioma. Acta Oncol 2013;52:147-52. [Crossref] [PubMed]
- Armstrong TS, Wefel JS, Wang M, et al. Net Clinical Benefit Analysis of Radiation Therapy Oncology Group 0525: A Phase III Trial Comparing Conventional Adjuvant Temozolomide With Dose-Intensive Temozolomide in Patients With Newly Diagnosed Glioblastoma. J Clin Oncol 2013;31:4076-84. [Crossref] [PubMed]
- Taphoorn M, Bottomley A, Coens C, et al. QLIF-07. Health-Related Quality of Life (HRQoL) in Patients With Progressive Glioblastoma Treated With Combined Bevacizumab and Lomustine Versus Lomustine Only (Randomized Phase III EORTC Study 26101). Neuro Oncol 2016;18:vi157. [Crossref]
- Scharfen CO, Sneed PK, Wara WM, et al. High activity iodine-125 interstitial implant for gliomas. Int J Radiat Oncol Biol Phys 1992;24:583-91. [Crossref] [PubMed]
- Patel S, Breneman JC, Warnick RE, et al. Permanent iodine-125 interstitial implants for the treatment of recurrent glioblastoma multiforme. Neurosurgery 2000;46:1123-8; discussion 1128-30. [Crossref] [PubMed]
- Gabayan AJ, Green SB, Sanan A, et al. GliaSite Brachytherapy for Treatment of Recurrent Malignant GliomasA Retrospective Multi-institutional Analysis. Neurosurgery 2006;58:701-9. [Crossref] [PubMed]
- Tselis N, Kolotas C, Birn G, et al. CT-Guided Interstitial HDR Brachytherapy for Recurrent Glioblastoma Multiforme. Strahlenther Onkol 2007;183:563-70. [Crossref] [PubMed]
- Darakchiev BJ, Albright RE, Breneman JC, et al. Safety and efficacy of permanent iodine-125 seed implants and carmustine wafers in patients with recurrent glioblastoma multiforme. J Neurosurg 2008;108:236-42. [Crossref] [PubMed]
- Shrieve DC, Alexander E, Wen PY, et al. Comparison of stereotactic radiosurgery and brachytherapy in the treatment of recurrent glioblastoma multiforme. Neurosurgery 1995;36:275-82; discussion 282-4. [Crossref] [PubMed]
- Cho KH, Hall WA, Gerbi BJ, et al. Single dose versus fractionated stereotactic radiotherapy for recurrent high-grade gliomas. Int J Radiat Oncol Biol Phys 1999;45:1133-41. [Crossref] [PubMed]
- Combs SE, Widmer V, Thilmann C, et al. Stereotactic radiosurgery (SRS): Treatment option for recurrent glioblastoma multiforme (GBM). Cancer 2005;104:2168-73. [Crossref] [PubMed]
- Kong DS, Lee JI, Park K, et al. Efficacy of stereotactic radiosurgery as a salvage treatment for recurrent malignant gliomas. Cancer 2008;112:2046-51. [Crossref] [PubMed]
- Patel M, Siddiqui F, Jin JY, et al. Salvage reirradiation for recurrent glioblastoma with radiosurgery: radiographic response and improved survival. J Neurooncol 2009;92:185. [Crossref] [PubMed]
- Cuneo KC, Vredenburgh JJ, Sampson JH, et al. Safety and efficacy of stereotactic radiosurgery and adjuvant bevacizumab in patients with recurrent malignant gliomas. Int J Radiat Oncol Biol Phys 2012;82:2018-24. [Crossref] [PubMed]
- Lederman G, Wronski M, Arbit E, et al. Treatment of recurrent glioblastoma multiforme using fractionated stereotactic radiosurgery and concurrent paclitaxel. Am J Clin Oncol 2000;23:155-9. [Crossref] [PubMed]
- Grosu AL, Weber WA, Franz M, et al. Reirradiation of recurrent high-grade gliomas using amino acid PET (SPECT)/CT/MRI image fusion to determine gross tumor volume for stereotactic fractionated radiotherapy. Int J Radiat Oncol Biol Phys 2005;63:511-9. [Crossref] [PubMed]
- Fokas E, Wacker U, Gross MW, et al. Hypofractionated Stereotactic Reirradiation of Recurrent Glioblastomas. Strahlenther Onkol 2009;185:235-40. [Crossref] [PubMed]
- Fogh SE, Andrews DW, Glass J, et al. Hypofractionated Stereotactic Radiation Therapy: An Effective Therapy for Recurrent High-Grade Gliomas. J Clin Oncol 2010;28:3048-53. [Crossref] [PubMed]
- Arcicasa M, Roncadin M, Bidoli E, et al. Reirradiation and lomustine in patients with relapsed high-grade gliomas. Int J Radiat Oncol Biol Phys 1999;43:789-93. [Crossref] [PubMed]
- Combs SE, Thilmann C, Edler L, et al. Efficacy of Fractionated Stereotactic Reirradiation in Recurrent Gliomas: Long-Term Results in 172 Patients Treated in a Single Institution. J Clin Oncol 2005;23:8863-9. [Crossref] [PubMed]
- Kohshi K, Yamamoto H, Nakahara A, et al. Fractionated stereotactic radiotherapy using gamma unit after hyperbaric oxygenation on recurrent high-grade gliomas. J Neurooncol 2007;82:297-303. [Crossref] [PubMed]
- Mayer R, Sminia P. Reirradiation tolerance of the human brain. Int J Radiat Oncol Biol Phys 2008;70:1350-60. [Crossref] [PubMed]
- Combs SE, Bischof M, Welzel T, et al. Radiochemotherapy with temozolomide as re-irradiation using high precision fractionated stereotactic radiotherapy (FSRT) in patients with recurrent gliomas. J Neurooncol 2008;89:205-10. [Crossref] [PubMed]
- Osman MA. Phase II trial of temozolomide and reirradiation using conformal 3D-radiotherapy in recurrent brain gliomas. Ann Transl Med 2014;2:44. [PubMed]
- Minniti G, Armosini V, Salvati M, et al. Fractionated stereotactic reirradiation and concurrent temozolomide in patients with recurrent glioblastoma. J Neurooncol 2011;103:683-91. [Crossref] [PubMed]
- Greenspoon JN, Sharieff W, Hirte H, et al. Fractionated stereotactic radiosurgery with concurrent temozolomide chemotherapy for locally recurrent glioblastoma multiforme: a prospective cohort study. Onco Targets Ther 2014;7:485-90. [Crossref] [PubMed]
- Gutin PH, Iwamoto FM, Beal K, et al. Safety and efficacy of bevacizumab with hypofractionated stereotactic irradiation for recurrent malignant gliomas. Int J Radiat Oncol Biol Phys 2009;75:156-63. [Crossref] [PubMed]
- Torcuator RG, Thind R, Patel M, et al. The role of salvage reirradiation for malignant gliomas that progress on bevacizumab. J Neurooncol 2010;97:401-7. [Crossref] [PubMed]
- Cabrera AR, Cuneo KC, Desjardins A, et al. Concurrent stereotactic radiosurgery and bevacizumab in recurrent malignant gliomas: a prospective trial. Int J Radiat Oncol Biol Phys 2013;86:873-9. [Crossref] [PubMed]
- Park KJ, Kano H, Iyer A, et al. Salvage gamma knife stereotactic radiosurgery followed by bevacizumab for recurrent glioblastoma multiforme: a case-control study. J Neurooncol 2012;107:323-33. [Crossref] [PubMed]
- Wong ET, Huberman M, Lu XQ, et al. Bevacizumab reverses cerebral radiation necrosis. J Clin Oncol 2008;26:5649-50. [Crossref] [PubMed]
- Torcuator R, Zuniga R, Mohan YS, et al. Initial experience with bevacizumab treatment for biopsy confirmed cerebral radiation necrosis. J Neurooncol 2009;94:63-8. [Crossref] [PubMed]
- Levin VA, Bidaut L, Hou P, et al. Randomized double-blind placebo-controlled trial of bevacizumab therapy for radiation necrosis of the central nervous system. Int J Radiat Oncol Biol Phys 2011;79:1487-95. [Crossref] [PubMed]
- Reardon DA, Sampson JH, Sahebjam S, et al. Safety and activity of nivolumab (nivo) monotherapy and nivo in combination with ipilimumab (ipi) in recurrent glioblastoma (GBM): Updated results from checkmate-143. Journal of Clinical Oncology 2016;34:suppl 2014.