Tumor treating fields: a novel and effective therapy for glioblastoma: mechanism, efficacy, safety and future perspectives
Introduction
Glioblastoma (GBM) is the most common and aggressive primary malignant brain tumor with grim prognosis. As reported by the Central Brain Tumor Registry of the United States (CBTRUS) (1), the incidence rate of GBM is the highest (3.20 per 100,000 population) in malignant central nervous system (CNS) tumors and has the lowest 1-year survival rate (35.7%) and 5-year survival rate (5.1%), compared to the other types of brain tumors. Even with maximum safe resection, radiation, and chemotherapies, including temozolomide (TMZ), the median OS is 14.6 months in the patients participated in clinical trial (2) and 10 to 11 months in general GBM population (3).
Tumor treating fields (TTF) are low-intensity alternating electric fields, which have been demonstrated to disrupt mitosis, induce cell cycle arrest, and induce apoptosis with antimitotic properties in a variety of tumor types (4-7). An external and transportable TTF therapeutic medical device, Optune® (Novocure Ltd., Haifa, Israel), was developed to deliver 200 KHz alternating electric fields via two pairs of flexible transducer array panels. Each panel has nine insulated electrodes placed on the shaved scalp and is connected to a portable electric field generator in a non-invasive and continuous administration (Figure 1). On April 8, 2011, the Food and Drug Administration (FDA) of the United States approved TTF as a mono-therapeutic modality for recurrent GBM based on the results of EF-11 trial, “NovoTTF-100A Versus Physician’s Choice Chemotherapy in Recurrent Glioblastoma: A Randomised Phase III Trial of A Novel Treatment Modality.” (8) (https://www.accessdata.fda.gov/cdrh_docs/pdf10/P100034a.pdf). Subsequently, the findings based on EF-14 trial, “Maintenance Therapy with Tumor-Treating Fields Plus Temozolomide vs Temozolomide Alone for Glioblastoma: A Randomized Clinical Trial”, revealed that newly diagnosed GBM patients experienced significantly prolonged PFS and OS by adding TTF to standard maintenance chemotherapy (TMZ) without systemic adverse events, except “itchy skin” (9). Therefore, the FDA expanded the indication of Optune® to newly diagnosed GBMs on October 5, 2015 (https://www.accessdata.fda.gov/cdrh_docs/pdf10/P100034S013b.pdf). TTF therapy is not only effective as a new armamentarium against GBM, but may also be combined with other systemic or local chemotherapeutic drugs or other treatment modalities (such as biologic therapies or radiation) that may to enable a more effective and synergistic therapeutic response in GBM. In this review, we will discuss the mechanism of TTF, summarize the results of pre-clinical and clinical trials, and discuss future potentials of this new anti-cancer treatment modality.
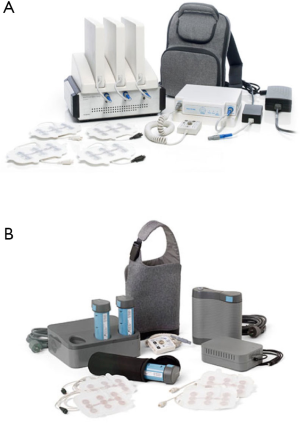
Fundamental physics and mechanism of action of TTF
Fundamental principles of electromagnetism
The physical mechanisms of TTF for mitotic disruption in tumor cells and subsequent antitumor effects are governed by Coulomb’s Law, Gauss’ Law, Ohm’s Law, and the continuity equation (10,11). Coulomb’s Law (Law of Electrostatics, 1785) states that the magnitude of the electric field () is inversely proportional to the square of the radial distance (r2) between the source point charge (q) and the test point (Eq. [1] and Figure 2).
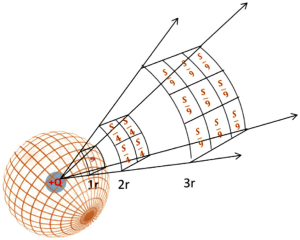
Suppose that we surround the charge q (positive or negative) by a spherical surface area. The flux of the electric field (ΦE) through the surface is given by Eq. [2] and Eq. [3] (Figure 3). The integral form defines the flux of electric field (ΦE) as an integral of the electric field ()where dA represents the vector of an infinitesimal surface element. These equations collectively represent Gauss’ Law, regardless of the surface shape, meaning that the flux of electric field (ΦE) through an enclosed surface area is equal to 1/ε0 times of the total electric charge (q) enclosed by the surface area, where ε0 represents the electric constant (11).
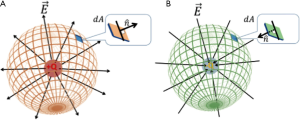
A differential equation of Eq. [3] derived from Eq. [2] states that the divergence of the electric field (), is proportional to the total density of electric charge (ρ), but inversely proportional to the electric constant (ε0). Therefore, the magnitude of TTF that traverses through various tissues (normal tissue or tumor tissue) within the brain varies by the charge density and dielectric properties.
Another important equation is Ohm’s Law (Eq. [4]), which is used to determine the penetration and distribution of electric fields due to the various electrical properties of conductors (such as brain tissue during TTF therapy). This law states that the electric current (I) through the conductor is inversely proportional to the electric resistance (R) of the conductor at a measured electric voltage (V) (Eq. [4]). Furthermore, Ohm’s Law is generalized by replacing the current (I), the electric resistance (R), and the electric potential (V), with the magnitude of electric field , the conductivity (σ), and the electric field density
, respectively (Eq. [5]). Hence, the density of electric field is proportional to the magnitude of electric field, which indicates that the change in electric potential on the scalp is higher than that in intracranial tissue (11).
The continuity equation in electromagnetic theory describes the electric charge conservation within a certain volume and time, where the divergence of current density is equal to the rate of the charge density change over time (Eq. [6]), including the loss of charge density (expressed as a negative rate of change) and the gain of charge density (expressed as a positive rate of change). The continuity equation plays an important role during the computer modeling of electric field distribution within the brain, which performs a variety of simulations to generate a visualized association between electric field distribution and the position of tumor tissue (11,12).
Furthermore, considering that TTF therapy is applied continuously on the patient’s scalp, the equations discussed above and the related derivatives can be used to measure time-varying distribution and their subsequent effect of TTF within the brain over time. Additionally, the degree of penetration and distribution of TTF from the surface of patient’s scalp traversing into the brain are associated with the properties of the tissues, such as conductivity and relative permittivity (10,11). Due to the fact that the majority of brain tissues are dielectric materials with different conductivity and permittivity, the varied characteristics of capacitive reactance might make the electric field distribution non-uniform (enhanced in some spots, but weakened in others).
Biophysical effects of TTF on tumor cells
Ions, polar molecules, and organelles within living cells are expected to react to the net effect of local interactive forces generated in the applied electrical fields during biological processes. Therefore, externally applied electric fields to human organs are expected to affect or even alter cellular processes within the electric field, including DNA replication and cell division, which may influence cell viability, depending on the varied intensity and frequency of these electric fields (4).
The biophysical and biological effects of TTF include the forced dipole alignment and dielectrophoresis. The electric fields exerted by TTF can preferentially influence proteins with large dipole moments which are involved in spindle formation and mitosis, such as Septin 2, 6, and 7, α/β tubulin and microtubules of spindles (4,5,7), and disrupt the various processes during mitosis, especially during metaphase, anaphase, and telophase (5,7,13). During metaphase, the electric fields impair the formation of microtubules by forced dipole alignment (13). The disruption of microtubule assembly can impede midline localization of the cytokinetic band, induce cytoplasmic blebbing and mitotic failure, and result in abnormal chromosome segregation in anaphase (7). In telophase, TTF was demonstrated to induce intra-cellular dielectrophoresis of macro-molecules and organelles (13). Therefore, the biological effect of TTF eventually leads to subsequent asymmetric chromosome segregation, mitotic arrest, or at least delay in cell division of active dividing cells. Since GBM tumor cells are the most actively replicating and dividing cells in the CNS, they are significantly adversely affected by the presence of TTF, which results in subsequent apoptosis and cell death (Figure 4).
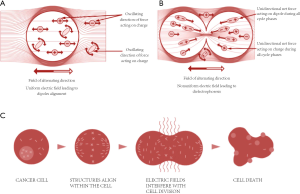
Furthermore, TTF-treated tumor cells exit the process of mitosis aberrantly and release cellular stress signals, such as the endoplasmic reticulum chaperonin calreticulin (CRT) and high mobility group box 1 protein (HMGB1). Obeid et al. demonstrated that CRT was important to induce antitumor immune responses because the blockade or knockdown of CRT (a chaperon protein with Ca++ binding capacity) inhibited the phagocytosis of tumor cells and immunogenicity (14,15). HMGB1, an endogenous chromatin-associated protein released from dying tumor cells, plays a critical role in the activation of HMGB1-mediated toll-like receptor 2 (TLR2) signaling and blockade of glycyrrhizin (a specific HMGB1 inhibitor) to induce tumor regression (16,17). The presence of those signals may facilitate immune activation, and immunogenic induced cell death, and eventually result in tumor destruction. The findings by Kirson et al. indicated that the anti-tumor immunogenicity in vivo might be up-regulated in the rabbit kidney injected with highly metastatic VX-2 tumors after TTF therapy, and found that the number of distant pulmonary metastatic mass was significantly reduced compared to the sham controls (P<0.05) (18).
Pre-clinical data and pilot study of TTF in GBM
A variety of human tumor models, such as colon adenocarcinoma (CT26), breast carcinoma (MDA-MB-231), GBM (intracranial F-98 glioma), non-small cell lung cancer (NSCLC) (Lewis lung carcinoma, H1299), pancreatic cancer, malignant melanoma (intradermal B16F1 melanoma), and VX-2 carcinoma have been studied in animal models (mice, rats, or rabbits) with TTF, and beneficial effects of tumor regression and inhibition of metastatic seeding due to TTF therapy were observed (5,6,18,19). Pre-clinical studies demonstrated that TTF disrupts the formation of mitotic spindles during metaphase and induces mitotic arrest and apoptosis of brain tumor cells. During cytokinesis, TTF plays a critical role in causing dielectrophoretic movement of polar molecules (4,5,13,20). Kirson et al. (5) concluded that the anti-tumor effect of TTF (inhibiting cell proliferation and inducing cell death) can be extended in a variety of cultured tumor cell lines as well as animal tumor models. They also found that TTF therapy could reduce the tumor volumes in Fischer rat models inoculated with glioma cells intracranially, but the difference in the reduced tumor volume between the treated group and sham control group was not significant. The amount of field directions significantly influenced this effect of inhibition (5). Kirson et al. further demonstrated a synergistic antitumor effect with cytotoxic chemotherapy (paclitaxel, doxorubicin, cyclophosphamide, or dacarbazine) combined with, TTF in vitro, in vivo, and using animal tumor models (6).
A single-arm pilot study was carried out to evaluate the efficacy and safety of TTF for two groups: recurrent GBMs (5,6) (N=10) and newly diagnosed GBMs (6) (N=10). PFS and OS in TTF treated group (N=10) were compared to a matched concurrent control group (received salvage chemotherapy at recurrence) and historical controls (5,6). They found that both median PFS (26.1 weeks) and OS (62.2 weeks) in the TTF group were more than 2 times that of the prior reported medians of historical controls (the average historical time to tumor progression and OS were 9.5±1.6 and 29.3±6 weeks, respectively) (5). The newly diagnosed GBM patients that were treated by TTF-TMZ combined therapy (N=10) vs. TMZ alone (N=32) had an improved PFS (TTF-TMZ: 155 weeks, concurrent controls: 31 weeks, P=0.0002) and OS (TTF-TMZ: >39 months, historical controls: 14.7 months, P=0.0018), respectively (6). These findings suggest that the efficacy and sensitivity of TMZ (chemotherapy) could be improved by combining it with adjuvant TTF in vitro (U-118 cell lines), in vivo (animal tumor models), and in newly diagnosed GBM patients. The most common side effect due to TTF was reversible contact dermatitis (itchy skin). No systemic toxicity or brain function impairment, such as seizures, from TTF alone or from TTF with TMZ, was observed.
TTF therapy for recurrent GBM
Phase III clinical trial for recurrent GBM: the EF-11 trial
Based on the synergistic antitumor effect of the combined TTF therapy with chemotherapy in the pilot study (6), Stupp et al. conducted a randomized open-label phase III clinical trial (EF-11 trial) to compare the efficacy of TTF monotherapy vs. standard of care in the form of chemotherapies chosen by physicians in recurrent GBM patients (8). A total of 237 patients (randomized ratio: 1:1, TTF arm: N=120, control arm: N=117) were included in this trial. As shown in Table 1, baseline characteristics of patients were balanced between the two groups. The majority of patients were enrolled at the second or higher recurrence. More than 80% of patients had received at least two lines of prior chemotherapies and 18–19% of patients were treated with bevacizumab (BEV) prior to the enrollment in either arm.
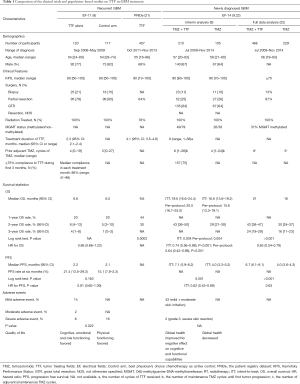
Full table
TTF therapy was administered continuously with 200 kHz alternating electric fields in two perpendicular directions and >0.7 V/cm of field intensity set at the center of the brain. Treatment breaks, while not recommended, were allowed up to an hour, twice per day, to maintain near-normal daily life. The minimal active therapy of TTF treatment was required, which means the patients were allowed to take more than two or three days off at the end of each 4 weeks of treatment upon satisfaction of the minimal required duration. A good compliance to TTF therapy with a mean daily usage of 20.6 hours was achieved in the EF-11 trial, and 78% of patients completed 4 weeks of TTF therapy (1 cycle).
The primary outcome of the EF-11 trial was OS. PFS at 6-month, 1-year survival rate, radiological response rate (RR), health-related quality of life (HRQoL) and safety were set as the secondary endpoints. The results showed that there was no statistical difference on OS between the intent-to-treat (ITT) group and control group [TTF: 6.6 months, control: 6.0 months; hazard ratio (HR): 0.86, 95% CI: 0.66–1.12, P=0.27]. There was no statistical difference of secondary endpoints detected between the two arms: PFS at 6-month (TTF: 21.4%, control: 15.1%, Chi square P=0.13), median PFS (TTF: 2.2 months, control: 2.1 months; HR 0.81, 95% CI: 0.60–1.09; log rank P=0.16), 1-year survival rate (TTF: 20%, active control: 20%), and RR (TTF: 14%, control: 9.6%, Chi square P=0.19) (Table 1). Therefore, the EF-11 trial failed to demonstrate the significant superiority of TTF over chemotherapy in recurrent GBM. However, TTF therapy was shown to be equivalent to active chemotherapy since the HR for death in TTF group was 0.86 (95% CI: 0.66–1.12) compared to the control group, but without the systemic side effects of chemotherapy. During post hoc subgroup analysis, it was found that the OS of TTF treatment group per protocol had improved when patients who did not receive TTF per protocol (<28 days) were removed.
Adverse events and HRQoL
Considering the physical mechanism and localized delivery of TTF therapy, the most frequent adverse events were found to be skin toxicities, including mild to moderate allergic dermatitis, irritant contact dermatitis, folliculitis, and erosions (23) (Figure 5, the pictures of skin toxicities from two of our patients as examples). All the skin reactions were reversible with simple dermatological treatments or by exposing the skin changes to air for a few days (Figure 5E presented the patient wearing the Novocure with wound uncovered). In the EF-11 trial, the most frequent TTF-related adverse events were mild (14%) to moderate (2%) scalp dermatitis in 16% of TTF patients, which were easily treated by topical corticosteroids or fully resolved after abstaining from TTF treatment for 24 to 48 hours. The occurrence of severe adverse events (grade 3) in TTF group was significantly lower than that of chemotherapy group (TTF: 6%, active chemotherapy: 16%, P=0.022), and more gastrointestinal, hematological and infectious adverse events were observed in control arm than that in TTF arm. Since long-term and continuous device wearing is required for TTF therapy, the longitudinal HRQoL was evaluated in the trial. Comparing the available HRQoL data between the two arms in the EF-11 trial (63/237, 27%), cognitive, emotional and role functioning favored TTF, while the patients in control arm performed better in physical functioning than those treated with TTF. No significant difference was observed in the global health scale and social functioning between the two arms.
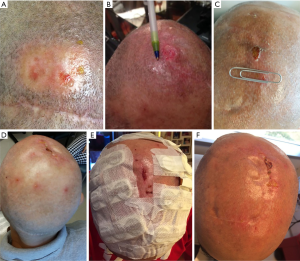
Strength and limitation
The EF-11 trial is the first prospective randomized open-label phase III clinical trial to evaluate the efficacy and safety of TTF therapy in comparison to chemotherapy in recurrent GBM patients. Although no significant survival improvement in the TTF arm compared to the control arm was observed, the absence of safety issues, reasonable compliance, minimal systematic toxicity, and stable HROoL of TTF therapy in comparison to chemotherapy makes this novel and non-invasive antitumor treatment modality very promising. According to the findings in the EF-11 trial, the US FDA approved TTF for recurrent GBM patients in 2011. The two arms were well balanced with craniotomy vs. biopsy prior to enrollment as a stratifying factor. Prior lower grade gliomas were balanced at 8% in TTF and 8% in control arm. It is known now that de novo GBM vs. secondary GBM had significantly different prognosis and should be stratified between the two groups. However, the association of Isocitrate dehydrogenase 1 and 2 (IDH1/2) as a glioma biomarker was not discovered until February 2009 (24). In addition, the blinded central radiology review of all MRIs according to Macdonald criteria is critical to maintain homogenous evaluation of all subject’s MRIs.
Several limitations exist in the EF-11 trial. First, considering the absence of commonly accepted standard treatment for recurrent GBM, the enrolled patients were heavily treated by several lines of prior chemotherapy before participating the EF-11 trial for both arms and different treatment drugs received for the control group (33% BEV, 25% nitrosoureas, and 11% TMZ, etc.) during the trial. This characteristic of patient population might subsequently contribute to the absence of significant difference in OS between the two groups. Second, 27 patients stopped TTF therapy in the beginning of treatment, often within a few days, due to noncompliance or inability to handle the device. The median duration of TTF administration was 2.3 months with tumor progression as the primary reason for stopping TTF. The premature discontinuation of TTF may have a negative effect on GBM survival control because the positive treatment response of TTF could be clinically demonstrated after a sufficient period of treatment duration. Third, MGMT, an important predictive factor for benefit of TMZ chemotherapy in GBM, was not examined. Fourth, 21 patients in the control group did not return to the investigational sites for treatment or follow up due to unknown reasons, thus details on disease progression and toxicity were not available, which reduced the volume of information regarding the control arm. One may speculate that these 21 patients probably experienced early disease progression and had transitioned to hospice care.
Post-approval clinical practice of TTF: patient registry dataset (PRiDe)
PRiDe was initiated to evaluate the efficacy and safety of TTF therapy in clinical practice between October 2011 and November 2013 after FDA approval of TTF for recurrent adult GBM patients. A total of 457 patients were analyzed and compared to the phase III clinical trial (EF-11). Demographic characteristics at baseline, such as age, gender and KPS were similar between PRiDe and the two arms in the EF-11 trial. However, the patients in PRiDe started TTF therapy sooner than those who participated in the EF-11 trial (TTF initiated at first recurrence: PRiDe: 33.3%, the EF-11 trial: 9%). In addition, 55.1% of patients in PRiDe were treated with prior BEV, whereas only 19% patients received BEV as prior treatment in the EF-11 trial (8,21). Median treatment duration of TTF in PRiDe was 4.1 months (95% CI: 3.5–4.8), whereas the median duration of TTF in the EF-11 trial was 2.3 months (95% CI: 2.1–2.4) for the TTF arm.
Median OS in PRiDe was 9.6 months, which was 3 months longer than the 6.6 months in TTF arm of the EF-11 trial (8,21). After stratification by the number of recurrence, compliance, KPS, and prior BEV usage in PRiDe, the median OS of the patients who were treated at first recurrence, with ≥75% compliance, 90–100 KPS, and without prior BEV treatment were 20, 13.5, 14.8, and 13.4 months, respectively (21). One-year and 2-year OS rates were both remarkably higher in PRiDe than those of the TTF arm in the EF-11 trial (1-year OS rate, PRiDe: 44%, EF-11 trial: 20%; 2-year OS rate, PRiDe: 30%, EF-11 trial: 9%) (8,21) (Table 1).
Adverse events and HRQoL
The most common TTF-related adverse events in PRiDe included skin reactions (24.3%), heat sensations (11.3%) and neurological disorders (10.4%). Heat sensations were described as “warm” which can be expected because heat is produced as a low current travels through the brain. The “warm” sensation never caused discomfort in patients and was not present when the device was off. The “tingling” sensation can be either related to the enhanced awareness of paresthesia from nerve injury during craniotomy in the presence of TTF, which were not reported in the EF-11 trial. The reported systemic adverse events in PRiDe, such as gastrointestinal disorders (2.9%) and infections (1.4%), were very rarely reported. No measurement of HRQoL was performed in PRiDe (8,21).
Strength and limitation
After FDA approval of TTF for recurrent GBM patients, TTF was available as standard of care clinical practice by certified physicians across 91 cancer centers in the US. Mrugala et al. analyzed the data retrospectively (PRiDe) and compared this real-world setting dataset with the EF-11 trial. The findings provide further evidence for the efficacy and absence of safety issues associated with of TTF therapy in recurrent GBMs in a larger population. Due to the fact that PRiDe is a retrospective study and EF-11 trial is a randomized phase III clinical trial, the results between PRiDe and EF-11 trial might not be comparable to some extent. Nevertheless, it is interesting to do a customary simple comparison.
The difference in survival between PRiDe and the EF-11 trial was likely due to the earlier initiation of TTF (TTF at first recurrence: 33% in PRiDe vs. 9% in the EF-11 trial) and longer durations of TTF treatments (PRiDe: 4.1 months, the EF-11 trial: 2.3 months). Furthermore, survival analysis was performed by stratification in PRiDe and demonstrated that the number of fewer recurrences, better compliance, higher KPS, fewer prior BEV treatment, and other factors that were not explored in the EF-11 trial, were significantly associated with better survival. Other limitations of the PRiDe analysis include: (I) concurrent chemotherapy or other treatments received (chemotherapy drugs, salvage radiation, radiosurgeries, or craniotomies) while on or after TTF therapy or subsequent therapies that were not taken into account; (II) Other potential prognostic factors that were not collected, such as MGMT and IDH1/2 statuses, marital status (to estimate family/emotional support), and HRQoL measurement.
TTF for newly diagnosed GBM
Phase III trial for newly diagnosed GBM: the EF-14 trial
The EF-14 trial was an open-label, randomized phase III clinical trial in patients with newly diagnosed GBM that investigated maintenance therapy with TTF plus TMZ vs. TMZ alone randomized in a 2:1 ratio. It was a sponsor initiated and funded international trial that involved 83 sites. The interim analysis was a pre-specified step of the trial when the first 315 subjects were followed for at least 18 months. The eligible adult patients included histologically confirmed supratentorial GBM, KPS ≥70%, normal function of bone marrow, liver, heart and kidney with stable clinical status without radiographic evidence of GBM disease progression 4 weeks after the completion of standard TMZ-based chemoradiation (9).
In the TTF plus TMZ group, the median duration of TTF administration was 9 months with a median of 6 cycles of TMZ, whereas the patients in TMZ alone group received a median of 4 cycles of TMZ. Sixty-seven percent (141/210) of the patients in TTF plus TMZ group continued TTF therapy after the first tumor progression and 75% (157/210) of patients had good compliance to TTF therapy during the first 3 treatment months. In addition, the percentage and type of second-line chemotherapy [such as nitrosoureas (40%), TMZ and BEV (40%)] offered at recurrence was balanced between two arms (TTF plus TMZ: 67%, TMZ alone: 57%).
The primary endpoint was PFS and OS was set as the secondary outcome. In the interim analysis, the ITT median PFS in TTF plus TMZ (N=210) was longer than those treated with TMZ alone (N=105) (TTF plus TMZ: 7.1 months, TMZ alone: 4.0 months; HR: 0.62, 98.7% CI: 0.43–0.89; log-rank P=0.001). Also, the ITT median OS in TTF plus TMZ group was 3 months longer than that of TMZ alone group (TTF plus TMZ: 19.6 months, TMZ alone: 16.6 months; HR: 0.74, 95% CI: 0.56–0.98; log-rank P=0.03), as well as the per-protocol population (TTF plus TMZ: 20.5 months, TMZ alone: 15.6 months; HR: 0.64, 99.4% CI: 0.42–0.98; log-rank P=0.004). The two-year survival rate was 14% higher in TTF plus TMZ group than that of TMZ alone group (TTF plus TMZ: 43%, TMZ alone: 29%) (9) (Table 1). An example of serial MRIs from a patient who was enrolled in the trial and was randomized to the TTF plus TMZ arm is presented (Figure 6). These findings demonstrated that TTF in combination with TMZ improved PFS and OS significantly in newly diagnosed GBM.
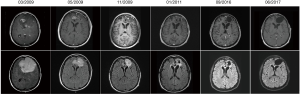
Adverse events and HRQoL
The patients in TTF plus TMZ group had a higher incidence of localized skin reactions or irritation than those in the TMZ alone group. Mild (grade 1) to moderate (grade 2) skin toxicity were reported in 43% of patients, and only 2% of patients reported severe skin reactions (grade 3) in the TTF plus TMZ group. The skin toxicities usually resolved following a temporary cessation of TTF treatment or by applying topical corticosteroids (Figure 5). Disorders in nervous system were more frequently reported in TTF plus TMZ arm, including grade 1–2 headache (TTF plus TMZ: 21%, TMZ alone: 14%), insomnia, confusion, and mild anxiety. The incidence of seizure was almost identical in the two arms (TTF plus TMZ: 7%, TMZ alone: 8%). For systemic adverse events, no significant changes in the incidence, distribution, and severity of systemic side effects, such as hematological toxicities (grade 3 and 4) (TTF plus TMZ: 12%, TMZ alone: 9%), gastrointestinal disorders (TTF plus TMZ: 5%, TMZ alone: 2%), and convulsions (TTF plus TMZ: 7%, TMZ alone: 7%) were observed in TTF plus TMZ group compared to TMZ alone group. The quality of life (EORTC QLQ C-30/BN20), KPS, and Mini-Mental Status Exam (MMSE) were evaluated and reported from the interim analysis data. Global health status improved in the TTF plus TMZ group (baseline to 3-month, baseline to 6-month), while a reduction was detected in TMZ alone group during the same intervals. Also, the addition of TTF to TMZ therapy had no negative effect on cognitive and functional capabilities (25).
Strength and limitation
The EF-14 trial is a multicenter, open-label, randomized phase III clinical trial designed to evaluate the efficacy and safety of TTF in combination with TMZ for newly diagnosed GBM treatment starting after chemoradiation. Based on the pre-specified interim analysis results in the ITT population (N=315), the patients in TTF plus TMZ had a median PFS of 3.1 months longer than those who were treated with TMZ alone (TTF plus TMZ: 7.1 months, TMZ alone: 4.0 months, P=0.001). After removing those patients who did not meet the pre-specified criteria of the protocol in both arms, the analysis for OS was performed in the per-protocol population (N=280). The results show a significant superiority of TTF plus TMZ on OS over TMZ alone (TTF plus TMZ: 20.5 months, TMZ alone: 15.6 months, P=0.004), as well as in the ITT population (TTF plus TMZ: 19.6 months, TMZ alone: 16.6 months, P=0.03). Subsequently, an analysis of the full data (N=695) was conducted and the results supported the prior conclusions (Table 1) (22).
Several limitations should be noted in the EF-14 trial: (I) only the progression-free patients after the completion of chemoradiation were enrolled, which may introduce variations in the delivery of TMZ and radiotherapy and exclude those who were more likely to have a poor prognosis; (II) second line therapies (chemotherapies, salvage radiation, radiosurgeries or craniotomies) after tumor progression in both groups were not reported while the TTF plus TMZ group allowed patients to continue TTF for up to 24 months or after the second GBM progression; (III) molecular analyses of tumor tissue were not performed, such as IDH1/2 status; (IV) for the perfect clinical trial, a sham group with patients wearing the TTF while the machine is not on therapeutic mode would be the ideal control group. Different from drug trials, as we all know, it is unethical to do many surgical trials with sham surgery in order to rule out placebo effect. The same argument holds true here. It is unethical for patients to shave head and wear the device for at least 18 hours out of 24 hours for up to 12 months or 24 months without obtaining potential benefit. Finally, it is understandable that the trial is still ongoing and limitations (II) and (III) listed above may be available when the full trial is completed. It is also expected that the subgroup analyses on causes of treatment failure as well as long-term survivors will be performed and presented in future full dataset publications.
Future perspectives on TTF
Based on the additive or synergistic therapeutic benefit with chemotherapy reported in preclinical data and the EF-14 trial, the concurrent usage of TTF with TMZ represents a novel treatment modality and strategy for GBM patients. Furthermore, the advantages of TTF therapy, including its localized and non-invasive mechanism of action, absence of systemic toxicity, and biophysical antitumor effect, render this device an appealing supplement in monotherapy or in combination with other current GBM treatments options, including surgery (a phase I pilot study on TTF + targeted surgical skull remodeling for recurrent GBMs, NCT02893137), radiation [a pilot study on TTF + BEV + Hypofractionated Stereotactic Irradiation (HSRT) for BEV-naive recurrent GBMs, NCT01925573], angiogenesis inhibitor (three phase II clinical trials for BEV + TTF for recurrent GBMs, NCT02663271, NCT02743078, and NCT01894061), and double-chemotherapy (a phase II trial for TMZ + BEV + TTF for newly diagnosed GBMs, NCT02343549).
In addition to these on-going trials, the impact of combining TTF with new treatment modalities of GBM therapies could also be explored to improve OS and PFS of this deadly disease. Recently, our group presented a retrospective study of our hospital data at the annual Society for Neuro-Oncology (SNO) scientific meeting, US, 2016 that the median OS of GBM patients treated with triple-chemotherapy (TMZ, BEV, and Irinotecan) with TTF was 32.8 months without significant systemic side effects (26). Furthermore, we performed post mortem examinations of patients who received the triple chemotherapies with TTF for extended period and found no permanent end-organ damage [presented at the World Federation of Neuro-Oncology Societies (WFNOS), Zurich, 2017] (27). Targeted therapy [e.g., EGFR-target therapy, such as Erlotinib (Tarceva) or Afatinib (Gilotrif)] with TTF is a good candidate regimen to try for EGFR mutation or amplified GBMs. Considering the success of immunotherapy in many cancers (melanoma and lung cancer) and the interactions between the brain and the immune system, the potential impact of immune checkpoint inhibitors seems appealing in GBM treatment. Several clinical trials have been ongoing to evaluate the efficacy and safety of avelumab, pembrolizumab, nivolumab and ipilimumab. However, the findings of a phase III trial (Nivolumab vs. BEV in recurrent GBM, NCT02017717) revealed that immunotherapy had no survival benefit comparing BEV for recurrent GBM patients (28). Currently, there is no trial focused on the combination of TTF and immunotherapy, but the synergistic effect may exist because TTF-treated tumor cells induced aberrant mitosis and exhibited cellular stress signals, such as CRT and HMGB1 (14-17), which may facilitate or enhance immune activation and immunogenic induced cell death.
Since the excellent compatibility between TTF and chemotherapeutic agents was demonstrated in both NSCLC (29) and GBM (9), there are a variety of on-going clinical trials exploring the effect of TTF in other cancers, including NSCLC (TTF + pemetrexed for advanced NSCLC, NCT00749346; TTF + PD-1 inhibitors or docetaxel for NSCLC, NCT02973789), pleural mesothelioma (TTF + pemetrexed and cisplatin or carboplatin for malignant pleural mesothelioma, NCT02397928), pediatric brain tumors (TTF for recurrent or progressive pediatric supratentorial high-grade glioma and ependymoma, NCT03033992), pancreatic cancer (TTF + gemcitabine or gemcitabine plus nabpaclitaxel for front-line therapy for advanced pancreatic adenocarcinoma, NCT01971281), ovarian carcinoma (TTF + paclitaxel for recurrent ovarian carcinoma, NCT02244502), and brain metastases (TTF following radiosurgery for NSCLC with 1–10 brain metastases, NCT02831959; a phase II trial on TTF vs. supportive care following optimal standard local treatment for NSCLC with 1–5 brain metastases, NCT01755624).
The next goal is to find prognostic biomarkers associated with TTF, which will help physicians to triage patients who will benefit from TTF or not. With ever improving molecular technologies and their sensitivities, including next generation sequencing and advanced liquid biopsy capacities, we are hopeful that specific biomarkers will be found from tissue, blood, or CSF in the near future. In addition, there is plenty of room to improve the current TTF system (version 2.0) for GBM from physics and bioengineering perspectives to increase efficacy without jeopardizing safety and to improve convenience to use, such as more efficient field frequency, lighter device and battery, longer battery life, easier connectivity of wires, and tolerance to scalp temperature fluctuations, etc. Therefore, we believe that TTF is a novel and effective modality for both recurrent and newly diagnosed GBM. This modality will allow us to expand the horizon by integrating TTF with the current and future standard cancer treatment to achieve superior survival benefit not only for GBM and brain metastases patients, but also for many other solid cancer patients.
Summary
Based on the principles of physics and the respective cellular biophysical effects, TTF therapy has a well-established mechanism for cancer therapy. TTF, as a novel electric-physical treatment modality, delivers low-intensity electric fields that permeate from the scalp surface into the brain tissues, disrupt various cellular processes of active dividing cells, especially mitosis, and thereby inhibits tumor growth. Based on the pre-clinical data, pilot study, post-marketing PRiDe, and the two phase III clinical trials, the promising survival benefit with fewer systematic adverse events, superior additive or even synergistic effect with standard chemotherapy, and better QoL have been demonstrated in the treatments for both newly diagnosed GBM and recurrent GBM. In the near future, we expect results of the full EF-14 trial and sub-analysis of the data, many of the on-going trials mentioned above, and newer generations of the TTF system from the manufacturer. Further investigations will be necessary to study biomarkers related to TTF and explore any combinational therapy with this novel anticancer treatment modality for the patients with GBM, brain metastases, and other solid cancers systemically.
Acknowledgements
We are very grateful for the assistance and support provided by Mr. Guangrong Lu for providing the patients’ scalp lesions photos, Dr. Joanna S. O’Leary, and Mr. Collin Harlan for their critical review of this manuscript and valuable suggestions. This study is partially supported by the fund from Dr. Marnie Rose Foundation.
Footnote
Conflicts of Interest: Jay-Jiguang Zhu received clinical trials research grants from: Boston Biomedical Sumitomo Dainippon Pharma Global Oncology, DEKK-TEC, Inc., Diffusion Pharmaceuticals LLC, Five Prime Therapeutics, Inc., Immuno-Cellular Therapeutics LTD, NRG/RTOG/National Cancer Institute, Novocure Inc., Tocagen Inc. The other author has no conflicts of interest to declare.
References
- Ostrom QT, Gittleman H, Fulop J, et al. CBTRUS statistical report: primary brain and central nervous system tumors diagnosed in the United States in 2008-2012. Neuro Oncol 2015;17 Suppl 4:iv1-iv62. [Crossref] [PubMed]
- Stupp R, Mason WP, van den Bent MJ, et al. Radiotherapy plus concomitant and adjuvant temozolomide for glioblastoma. N Engl J Med 2005;352:987-96. [Crossref] [PubMed]
- Zhu P, Du XL, Lu GR, et al. Survival benefit of glioblastoma patients after FDA approval of temozomide concomitant with radiation and bevacizumab: A population-based study. Oncotarget 2017;8:44015-31. [PubMed]
- Kirson ED, Gurvich Z, Schneiderman R, et al. Disruption of cancer cell replication by alternating electric fields. Cancer Res 2004;64:3288-95. [Crossref] [PubMed]
- Kirson ED, Dbalý V, Tovaryš F, et al. Alternating electric fields arrest cell proliferation in animal tumor models and human brain tumors. Proc Natl Acad Sci U S A 2007;104:10152-7. [Crossref] [PubMed]
- Kirson ED, Schneiderman RS, Dbalý V, et al. Chemotherapeutic treatment efficacy and sensitivity are increased by adjuvant alternating electric fields (TTFields). BMC Med Phys 2009;9:1. [Crossref] [PubMed]
- Gera N, Yang A, Holtzman TS, et al. Tumor treating fields perturb the localization of septins and cause aberrant mitotic exit. Prigent C, editor. PLoS One 2015;10:e0125269.
- Stupp R, Wong ET, Kanner AA, et al. NovoTTF-100A versus physician’s choice chemotherapy in recurrent glioblastoma: A randomised phase III trial of a novel treatment modality. Eur J Cancer 2012;48:2192-202. [Crossref] [PubMed]
- Stupp R, Taillibert S, Kanner AA, et al. Maintenance therapy with tumor-treating fields plus temozolomide vs temozolomide alone for glioblastoma: A randomized clinical trial. JAMA 2015;314:2535-43. [Crossref] [PubMed]
- Lok E, Swanson KD, Wong ET. Tumor treating fields therapy device for glioblastoma: physics and clinical practice considerations. Expert Rev Med Devices 2015;12:717-26. [Crossref] [PubMed]
- Lok E, Sajo E. Fundamental physics of tumor treating fields in: alternating electric fields therapy in oncology. 4 ed. Cham: Springer International Publishing; 2016:15-27.
- Lok E, Hua V, Wong ET. Computed modeling of alternating electric fields therapy for recurrent glioblastoma. Cancer Med 2015;4:1697-9. [Crossref] [PubMed]
- Gutin PH, Wong ET. Noninvasive application of alternating electric fields in glioblastoma: a fourth cancer treatment modality. Am Soc Clin Oncol Educ Book 2012.126-31. [PubMed]
- Chaput N, De Botton S, Obeid M, et al. Molecular determinants of immunogenic cell death: surface exposure of calreticulin makes the difference. J Mol Med 2007;85:1069-76. [Crossref] [PubMed]
- Obeid M, Tesniere A, Ghiringhelli F, et al. Calreticulin exposure dictates the immunogenicity of cancer cell death. Nat Med 2007;13:54-61. [Crossref] [PubMed]
- Candolfi M, Yagiz K, Foulad D, et al. Release of HMGB1 in response to proapoptotic glioma killing strategies: efficacy and neurotoxicity. Clin Cancer Res 2009;15:4401-14. [Crossref] [PubMed]
- Curtin JF, Liu N, Candolfi M, et al. HMGB1 mediates endogenous TLR2 activation and brain tumor regression. PLoS Med 2009;6:e10. [Crossref] [PubMed]
- Kirson ED, Giladi M, Gurvich Z, et al. Alternating electric fields (TTFields) inhibit metastatic spread of solid tumors to the lungs. Clin Exp Metastasis 2009;26:633-40. [Crossref] [PubMed]
- Giladi M, Weinberg U, Schneiderman RS, et al. Alternating electric fields (tumor-treating fields therapy) can improve chemotherapy treatment efficacy in non-small cell lung cancer both in vitro and in vivo. Semin Oncol 2014;41 Suppl 6:S35-41. [Crossref] [PubMed]
- Fonkem E, Wong ET. NovoTTF-100A: a new treatment modality for recurrent glioblastoma. Expert Rev Neurother 2012;12:895-9. [Crossref] [PubMed]
- Mrugala MM, Engelhard HH, Tran DD, et al. Clinical practice experience with NovoTTF-100A™ system for glioblastoma: The Patient Registry Dataset (PRiDe). Semin Oncol 2014;41 Suppl 6:S4-S13. [Crossref] [PubMed]
- Stupp R, Idbaih A, Steinberg DM, et al. LTBK-01: Prospective, multi-center phase III trial of tumor treating fields together with temozolomide compared to temozolomide alone in patients with newly diagnosed glioblastoma. Neuro Oncol 2016;18:i1. [Crossref] [PubMed]
- Lacouture ME, Davis ME, Elzinga G, et al. Characterization and management of dermatologic adverse events with the NovoTTF-100A System, a novel anti-mitotic electric field device for the treatment of recurrent glioblastoma. Semin Oncol 2014;41 Suppl 4:S1-14. [Crossref] [PubMed]
- Yan H, Parsons DW, Jin G, et al. IDH1 and IDH2 mutations in gliomas. N Engl J Med 2009;360:765-73. [Crossref] [PubMed]
- Zhu JJ, Pannullo S, Mehdorn M, et al. ATCT-35Quality of life, cognitive function and functional status in the EF-14 trial: A prospective, multi-center trial of ttfields with temozolomide compared to temozolomide alone in patients with newly diagnosed GBM. Neuro Oncol 2015;17:v9. [Crossref]
- Lu G, Rao M, Delumpa LC, et al. PDCT-16. Survival benefit with triple chemotherapy and TTFields for glioblastoma. Neuro Oncol 2016;18:vi149-9. [Crossref]
- Lu G, Rao M, Zhu P, et al. P09.63 Postmortem evaluation of end-organ toxicity in patients with glioblastoma treated with temozolomide, bevacizumab and irinotecan. Neuro Oncol 2017;19:iii84-4. [Crossref]
- Reardon DA, Omuro A, Brandes AA, et al. OS10.3 Randomized phase 3 study evaluating the efficacy and safety of nivolumab vs bevacizumab in patients with recurrent glioblastoma: CheckMate 143. Neuro Oncol 2017;19:iii21-1. [Crossref]
- Pless M, Droege C. A phase I/II trial of Tumor Treating Fields (TTFields) therapy in combination with pemetrexed for advanced non-small cell lung cancer. Lung Cancer 2013;81:445-50. [Crossref] [PubMed]