“Cor Occidere”: a novel strategy of targeting the tumor core by radiosurgery in a radio- and chemo-resistant intracranial hemangiopericytoma
Introduction
Hemangiopericytoma (HPC) is a rare neoplasm originating from Zimmerman pericytes, which are localized at the musculoskeletal and skin tissues; it can also present with intracranial involvement in 1% of cases (1). HPC is notoriously resistant to chemotherapy and radiotherapy (RT). As such, control rates even despite combination surgery and adjuvant RT have been dismal, with 50% reported in some series (2,3).
Here, we present a unique case of recurrent intracranial HPC in an adolescent female, who had received two prior courses of high dose RT (as adjuvant post-surgical resection, and subsequently for salvage), and had to be considered for a 3rd course due to tumor progression. The ability to dose escalation was however limited by the tumor locality in the cavernous sinus and the cumulative large doses of RT to the critical structures. This demanded a novel approach, which we termed “Cor Occidere” (targeting the tumor core, Latin); we intentionally compromised on dose coverage to the tumor margin, delivering a large ablative radiation dose to the tumor core using stereotactic radiosurgery (SRS). With this novel technique, we achieved sustained control of her treatment-refractory disease, outperforming all lines of systemic treatment.
Case presentation
Our patient was a 36-year old female who presented to the SRS service with aggravating clinical symptoms of multiple left cranial nerve palsies over the III and V2, 3 distributions, which corresponded to her diagnosis of a recurrent HPC localized at the left cavernous sinus; she was referred for consideration of re-irradiation following two previous courses of high dose RT (Figure 1). These were consistent with her presenting symptoms at the time of initial diagnosis 13 years prior in 2000, which were a 6-month history of diplopia and left facial numbness, with MRI of the brain revealing a homogeneously enhancing extra-axial lesion located in the left cavernous sinus. Differential diagnoses at that time included HPC and atypical meningioma. She was thus managed by gross total surgical resection, followed by adjuvant RT of 54 Gy in 30 fractions using 2-dimensional technique (to surgical bed and R1 disease) upon histopathological confirmation of a HPC. Post-treatment, she endorsed residual facial numbness, but annual MRI surveillance indicated stable radiological findings. Unfortunately, 8 years post-treatment, she developed asymptomatic radiological progression with a 1.1×1.7 cm2 left parasellar lesion that was detected on MRI. The tumor, in spite of its small size, was deemed to be unresectable due to its adherence to the cavernous sinus bed. She was thus dealt with a 2nd course of stereotactic RT (SRT) of 50.4 Gy in 28 fractions delivered to the MRI-defined gross tumor volume (GTV), which achieved tumor control based on MRI for 4 years until the presentation in 2013 (Figure 1).
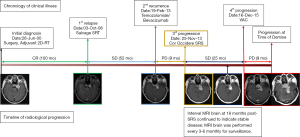
Prior to the consult for SRS, patient had progressed through a trial of 8 cycles of bevacizumab/temozolomide to no effect. In this instance when the tumor is juxta-opposed to the brain stem, optic nerve, and internal carotid artery, notwithstanding the fact that these tissues have been multiply irradiated, optimal dose coverage of the GTV with a single dose of 18 Gy was not feasible. We therefore designed a novel SRS plan to intentionally only target the tumor core (defined by 2 mm-margin from the GTV margin) with 18 Gy, while compromising coverage of the tumor margin, restricting the doses to the critical organs to <10 Gy. Dosimetric indices of our SRS plan are illustrated in Figure 2.
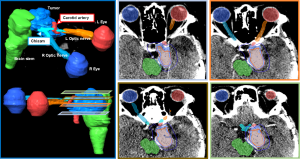
Remarkably, over a period of 25 months post-SRS, we observe persistent disease stability based on 3-month interval MRI. Interestingly, the tumor remained homogenously enhancing, without appearance of central necrosis. Of note, patient did not report onset of any RT-related toxicities. Eventually, following the unexpected prolonged response to SRS, the patient developed a 4th episode of tumor progression. She failed to respond to a trial of vincristine, adriamycin, and cyclophosphamide (3 cycles); she subsequently demised 9 months after from disseminated leptomeningiomatosis (Figure 1).
Discussion
Intracranial HPC is often indistinguishable from extra-axial meningioma on radiology, but despite the semblance on appearance, both tumors have widely disparate clinical behavior. HPC is intrinsically resistant to chemotherapy and RT (3-5), which is consistent with the high rates of recurrence that are often observed following first-line surgery with or without adjuvant RT and limited systemic therapeutic options (3,6,7). Upon recurrence, salvage RT is thus often the modality of choice. The best outcomes with salvage RT have also largely been observed in retrospective small case series using SRS (80–90% response rates), but several caveats of these studies ought to be discussed (8-12); in particular, prescribed doses vary between studies. It is also unclear if radioresistant HPCs are sensitive to variations in fraction size, which would influence the choice between profoundly hypofractionated SRS (>6 Gy per fraction) or conventionally fractionated RT (1.8–2 Gy per fraction). While effective, the feasibility of a repeat course of RT is dependent on a number of factors, including recurrent tumor volume, critical organs at risk, time interval from previous RT, and patient functional status (13-15).
Here, we present a recurrent HPC case whose clinical circumstances called for an innovative approach to RT delivery. Our treatment planning considerations included the following: (I) SRS or SRT for delivery; (II) the minimum dose for efficacy; and (III) the cumulative exposure to the critical organs, specifically the brain stem, optic nerves, and the internal carotid artery. Foremost, a 3rd course of conventionally fractionated RT would have led to extremely high cumulative doses to the critical organs (BED =167.0 Gy, alpha/beta =3 Gy), along with the uncertainty of the degree of normal tissue repair in the time-interval between treatments. Next, given the variation of SRS doses (16–20 Gy) in the few reported series, we preferred an intermediate dose of 18 Gy in a single fraction for planning feasibility. Lastly, in accordance with the absolute principle of “first do no harm”, we prescribed conservative dose constraints of D1cc <10 Gy for the critical organs (16-18). Nonetheless, given that the tumor was juxta-opposed to these organs (Figure 2), achieving full dose coverage while fulfilling the preset dose constraints was impossible. Our only solution was therefore to spatially modulate the RT dose to the tumor; we targeted the tumor core, which was arbitrarily defined by a geometric-based concept of 2 mm from the margin. Our simplistic rationale was that SRS would arrest the most proliferative cells that were closest to the tumor center that is enriched for oxygen and pro-survival cellular signals (19,20). We termed this—Cor Occidere.
Our observations in this unique case raised several scientific possibilities. The fact that partial irradiation of the tumor resulted in sustained control of a radioresistant HPC would suggest the relevance of the tissue microenvironment in modulating RT-response. In this instance, transmission of pro-death and cellular checkpoint signals to the tumor periphery, which were trigged by ionizing radiation, could have resulted in overall tumor growth arrest (20). Additionally, large single doses of RT are also known to activate the anti-tumor immune signaling cascade, and such tumor-microenvironmental interactions could have been also been in play in this instance (19-21).
Conclusions
In summary, our case of recurrent HPC highlights the limited therapeutic options and unfavorable outcomes for this aggressive disease. Novel strategies are therefore desperately needed. Here, we employed an innovative approach of Cor Occidere SRS; while suboptimal by conventional standards, we achieved a better than expected outcome and substantially prolonged the survival of the patient.
Acknowledgements
Funding: MLK Chua is supported by the National Medical Research Council Singapore Transition Award-#NMRC/TA/0030/2014, and the Duke-NUS Oncology Academic Program Proton Philanthropic Fund.
Footnote
Conflicts of Interest: The authors have no conflicts of interest to declare.
Informed Consent: Written informed consent was obtained from the patient for publication of this manuscript and any accompanying images.
References
- Goellner JR, Laws ER Jr, Soule EH, et al. Hemangiopericytoma of the meninges. Mayo Clinic experience. Am J Clin Pathol 1978;70:375-80. [Crossref] [PubMed]
- Rutkowski MJ, Jian BJ, Bloch O, et al. Intracranial hemangiopericytoma: clinical experience and treatment considerations in a modern series of 40 adult patients. Cancer 2012;118:1628-36. [Crossref] [PubMed]
- Rutkowski MJ, Sughrue ME, Kane AJ, et al. Predictors of mortality following treatment of intracranial hemangiopericytoma. J Neurosurg 2010;113:333-9. [Crossref] [PubMed]
- Kim YJ, Park JH, Kim YI, et al. Treatment Strategy of Intracranial Hemangiopericytoma. Brain Tumor Res Treat 2015;3:68-74. [Crossref] [PubMed]
- Chamberlain MC, Glantz MJ. Sequential salvage chemotherapy for recurrent intracranial hemangiopericytoma. Neurosurgery 2008;63:720-6; author reply 6-7. [Crossref] [PubMed]
- Kirn DH, Kramer A. Long-term freedom from disease progression with interferon alfa therapy in two patients with malignant hemangiopericytoma. J Natl Cancer Inst 1996;88:764-5. [Crossref] [PubMed]
- Glaholm J, Bloom HJ, Crow JH. The role of radiotherapy in the management of intracranial meningiomas: the Royal Marsden Hospital experience with 186 patients. Int J Radiat Oncol Biol Phys 1990;18:755-61. [Crossref] [PubMed]
- Veeravagu A, Jiang B, Patil CG, et al. CyberKnife stereotactic radiosurgery for recurrent, metastatic, and residual hemangiopericytomas. J Hematol Oncol 2011;4:26. [Crossref] [PubMed]
- Spitz FR, Bouvet M, Pisters PW, et al. Hemangiopericytoma: a 20-year single-institution experience. Ann Surg Oncol 1998;5:350-5. [Crossref] [PubMed]
- Vuorinen V, Sallinen P, Haapasalo H, et al. Outcome of 31 intracranial haemangiopericytomas: Poor predictive value of cell proliferation indices. Acta Neurochir (Wien) 1996;138:1399-408. [Crossref] [PubMed]
- Cho JM, Kim SH, Kim SH, et al. Recurred cerebellopontine angle haemangiopericytoma 5 years after stereotactic radiosurgery. Clin Neurol Neurosurg 2011;113:931-3. [Crossref] [PubMed]
- Chang SD, Sakamoto GT. The role of radiosurgery for hemangiopericytomas. Neurosurg Focus 2003;14:e14. [Crossref] [PubMed]
- Brunori A, Delitala A, Oddi G, et al. Recent experience in the management of meningeal hemangiopericytomas. Tumori 1997;83:856-61. [PubMed]
- Dufour H, Metellus P, Fuentes S, et al. Meningeal hemangiopericytoma: a retrospective study of 21 patients with special review of postoperative external radiotherapy. Neurosurgery 2001;48:756-62; discussion 62-3. [PubMed]
- Galanis E, Buckner JC, Scheithauer BW, et al. Management of recurrent meningeal hemangiopericytoma. Cancer 1998;82:1915-20. [Crossref] [PubMed]
- Marks LB, Yorke ED, Jackson A, et al. Use of normal tissue complication probability models in the clinic. Int J Radiat Oncol Biol Phys 2010;76:S10-9. [Crossref] [PubMed]
- Bentzen SM, Constine LS, Deasy JO, et al. Quantitative Analyses of Normal Tissue Effects in the Clinic (QUANTEC): an introduction to the scientific issues. Int J Radiat Oncol Biol Phys 2010;76:S3-9. [Crossref] [PubMed]
- Benedict SH, Yenice KM, Followill D, et al. Stereotactic body radiation therapy: the report of AAPM Task Group 101. Med Phys 2010;37:4078-101. [Crossref] [PubMed]
- Song CW, Park H, Griffin RJ, et al. Radiobiology of stereotactic radiosurgery and stereotactic body radiation therapy. In: Levitt S, Purdy J, Perez C, et al, editors. Technical Basis of Radiation Therapy. Medical Radiology. Berlin: Springer, 2011:51-61.
- Sperduto PW, Song CW, Kirkpatrick JP, et al. A hypothesis: indirect cell death in the radiosurgery era. Int J Radiat Oncol Biol Phys 2015;91:11-3. [Crossref] [PubMed]
- Song CW, Lee YJ, Griffin RJ, et al. Indirect tumor cell death after high-dose hypofractionated irradiation: implications for stereotactic body radiation therapy and stereotactic radiation surgery. Int J Radiat Oncol Biol Phys 2015;93:166-72. [Crossref] [PubMed]