Targets for therapy in biliary tract cancers: the new horizon of personalized medicine
Biliary tract cancers (BTCs) have traditionally been classified according to their location. Intrahepatic cholangiocarcinoma (IH-CCA) arises from the peripheral bile ducts within the liver beyond the secondary biliary radicals while extrahepatic cholangiocarcinoma (EH-CCA) arises from bile ducts outside the liver and can be subclassified into either hilar cholangiocarcinoma or distal cholangiocarcinoma. Gallbladder carcinoma (GBC) arise from the gallbladder. While these anatomic distinctions continue to be highly relevant for the diagnosis, prognosis, and management of BTC, an increasing awareness of their molecular heterogeneity has paved the way for new therapeutic approaches in these diseases.
In China, BTC is the 24th most common cancer and the 17th leading cause of cancer death (1). The incidence of BTC appears to be increasing globally, due in large part to a rising incidence of IH-CCA (2,3). In fact, BTCs were the most rapidly rising malignancy in Shanghai, China between 1972 and 1994, with an increase of 119% in men and 124% in women and an incidence rate of ~5.5 per 100,000 people (4,5). The mortality rate of BTC is 1.8/100,000 in China versus 1.0/100,000 in the United States (6). Epidemiologic variance between Eastern and Western populations is thought to be due in part to a difference in risk factors. For example, obesity and chronic inflammation from primary sclerosing cholangitis (PSC) are more prevalent in Western countries (7), whereas the prevalence of the liver parasite Opisthorchis viverrini is significantly higher in Thailand, Laos, and Cambodia (8,9). The liver fluke Clonorchis sinensis has also been associated with the development of cholangiocarcinoma and is endemic to rural China, especially in the northeastern province of Heilongjiang and the southern provinces of Guangdong and Guangxi (10,11). Other risk factors for CCA include diabetes, chronic viral hepatitis B or C, anatomical abnormalities in the biliary tract such as choledochal cysts, and Lynch syndrome. Risk factors for GBC include polyps, Salmonella Typhi, and chronic cholecystitis (12,13).
Unresectable or advanced BTC is associated with a 5-year survival rate of 5–10% (14). The current standard of care for unresectable BTCs is systemic chemotherapy. Gemcitabine was established as palliative therapy in 1996 (15), and remained the standard of care until the phase III ABC-02 trial demonstrated a survival advantage for the combination of cisplatin and gemcitabine over gemcitabine in the front-line setting for advanced disease [median overall survival (OS) 11.7 vs. 8.1 months, respectively, hazard ratio 0.64, P<0.001] (16). The survival advantage of the combination over gemcitabine alone was confirmed in a Japanese population (17). Recently, the ABC-06 trial demonstrated a modest survival benefit with oxaliplatin/5-fluoruracil (mFOLFOX) and active symptom control (ASC) after progression on cisplatin and gemcitabine (compared to ASC alone), with a median OS of 6.2 vs. 5.3 months, respectively (adjusted hazard ratio 0.69 (P=0.03) (18).
While cytotoxic regimens continue to be evaluated in clinical trials, several genotyping efforts have identified specific potentially targetable molecular aberrations in BTC and the investigational paradigm for unresectable BTC now includes targeted therapy in the front-line and treatment-refractory settings. An understanding of these evolving treatment paradigms requires an understanding of the molecular and genomic underpinnings of the disease. In this review we identify the molecular aberrations identified in BTC and the emerging therapies which target these molecular mutations (Table 1).
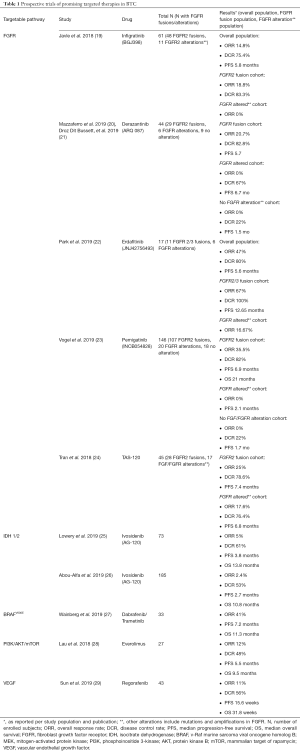
Full table
Molecular and genomic characteristics
The complex molecular pathogenesis of BTC involves alterations in multiple pathways including protein kinases (FGFR2, BRAF, HER2), epigenetic modification (IDH1/IDH2), and DNA damage repair (BRCA2, TP53) (30-33). The advent of genomic tumor profiling via next-generation sequencing (NGS) has allowed for the characterization of specific somatic aberrations. In addition, improved detection of circulating plasma cell-free DNA may aid in genomic profiling when inadequate tissue is obtained at biopsy (34). The anatomic location of the tumor—intrahepatic, extrahepatic, or gallbladder—greatly influences the mutational profile likely to be found within the tumor. In one series, potentially targetable genetic alterations were identified in 39% of 239 analyzed BTC cases (35). FGFR2 fusions and IDH1/2 mutations were observed most commonly in IH-CCA, PRKACA/PRKACB fusions were most common in EH-CCA, and ERBB2/3, TSC1, and PTEN alterations were seen in gallbladder cancer.
Exposure to specific risk factors may be associated with differing BTC molecular profiles. A study of 209 patients with CCA in Asia and Europe reported higher rates of TP53 mutations in O. viverrini-related CCA compared to non-O. viverrini-related CCA (40% vs. 9%), along with higher rates of SMAD4 (19.4% vs. 5.8%) and GNAS (5.6% vs. 0%) mutations (36). Conversely, there were lower rates of BAP1 in O. viverrini-related CCA (2.8% vs. 10.5%) along with lower rates of IDH1/2 (2.8% vs. 9%) mutations. In another study, TP53 mutations in IH-CCA were more likely to be associated with Hepatitis B surface antigen (HBsAg) seropositivity and KRAS mutations associated with HBsAg-seronegative patients (31). Hepatitis B or C positivity may also be associated with an increased likelihood of FGFR alteration (37). Finally, genomic sequencing of over 500 CCA tumors demonstrated four distinct molecular clusters (33). The clusters with higher rates of fluke infections had significantly more somatic mutations, including higher rates of aberrations in ERBB2, TP53, and BRCA 1/2 and lower rates of FGFR and IDH 1/2 mutations, and were associated with a poorer survival compared to the clusters with lower rates of fluke-associated tumors (P<0.001). Further research is needed to further characterize the link between risk factors, pathogenesis and mutational drivers of the various BTCs.
Multiple studies have found prognostic implications for specific genetic alterations in BTC (38-41). Mutations in TP53, KRAS, and the MAP/ERK pathway mutation have been associated with a poor prognosis, while FGFR pathway alterations, and specifically FGFR fusions, have been associated with a favorable prognosis and improved survival compared to those without fusions (41-43). Data on the prognostic implication of aberrations in IDH1/IDH2, HER2/neu, c-MET, and the PI3K/AKT/mTOR pathway are conflicting (32,44-48). While the data are still maturing regarding the possible prognostic implications of specific genomic mutations, emerging data suggest that certain mutations drive cancer growth and progression, thus making them prime targets for therapeutic intervention.
FGFR2
The complex fibroblast growth factor (FGF) pathway is involved in a wide array of biological processes from embryonal development to angiogenesis and wound repair (49). It consists of four transmembrane tyrosine kinase receptors (FGFR 1-4) and 22 FGFs, with downstream activation of RAS/RAF/MEK, JAK/STAT, PI3K/AKT pathways (40). Dysregulated FGF signaling has been associated with tumor proliferation, migration, and angiogenesis in a variety of malignancies (42,50). In BTC, the most common FGFR pathway aberrations are gene fusions involving FGFR2, which are seen at a frequency of 10–16% in IH-CCA, although point mutations and gene amplifications are also observed (46,51,52). FGFR2-BICC1 was the first fusion reported in cholangiocarcinoma in 2013 (53), and several subsequent studies have uncovered more than 50 additional fusion partners. FGFR pathway abnormalities are rarely seen in EH-CCA or GBC (46,51). In addition, studies in North American cohorts have shown a significantly higher proportion of FGFR2 aberrations among women (39,40), a trend not seen in Asian cohorts (54).
FGFR inhibitors have demonstrated efficacy in patients with FGFR-altered advanced refractory cholangiocarcinoma in multiple phase I and II studies. Infigratinib (BGJ398), an oral selective pan-FGFR kinase inhibitor, showed an overall response rate (ORR) of 14.8%, disease control rate (DCR) of 75.4%, and a median PFS of 5.8 months in a phase II single arm trial (19). This was driven by patients with FGFR2 fusions where the ORR was 18.8% and the DCR was 83.3%. No responses were seen in patients with FGFR amplifications or mutations. Adverse events included hyperphosphatemia (72.1%), fatigue (36.1%), stomatitis (29.5%), and alopecia (26.2%). Derazantinib demonstrated an ORR of 21% and DCR of 83%, with a median progression-free survival of 5.7 months, in patients with FGFR fusions (20,21). While no responses were seen in the FGFR mutated or amplified group, 67% achieved disease control with a median progression-free survival of 6.7 months and OS has not been reached in either arm (20).
Preliminary data on pemigatinib, an FGFR-selective inhibitor of FGFR 1-3, demonstrated an ORR of 35.5%, including a partial response rate of 32.7% in patients with FGFR2 fusions, in the recently reported FIGHT-202 study (23). DCR in the fusion population was 82%, with a duration of response of 7.5 months, and a median progression-free survival and preliminary OS of 6.9 and 21.1 months, respectively. Similar to infigratinib, no objective responses were seen with pemigatinib in patients with FGFR amplified or mutated patients (DCR was 40%, consisting of stable disease only), and median progression-free survival was 2.1 months. The adverse event profiles of pemigatinib and derazantinib were similar to that of infigratinib (20,23). Additional studies of futibatinib (TAS120) (55), Debio 1347 (56), and Erdafitinib (57) are ongoing. Phase III studies comparing single agent FGFR inhibitor to standard cisplatin/gemcitabine in the first line metastatic setting are underway with infigratinib (NCT03773302) and pemigatinib (NCT03656536).
A limitation of the ATP-competitive FGFR inhibitors is the development of resistance after exposure. In-vitro, resistance develops via FGFR2 kinase domain mutations (58). Goyal et al. reported that three patients with IH-CCA harboring an FGFR2 translocation who initially responded to treatment with infigratinib subsequently acquired resistance via polyclonal recurrent mutations in the FGFR2 kinase domain (50). Of note, FGFR2 V564F mutation, a gatekeeper mutation that leads to steric clash between the FGFR2 binding domain and infigratinib, emerged in all three patients. The irreversible pan-FGFR inhibitor, TAS120, covalently binds to the P-loop cysteine residue of FGFR and has shown potency in preclinical models against acquired FGFR2 kinase domain mutations (59). Goyal et al. recently showed proof of concept of TAS120 overcoming acquired resistance to the ATP-competitive inhibitors infigratinib and Debio1347 in four patients with FGFR2-fusion positive IH-CCA (60). Functional assessment and modeling of the acquired FGFR2 kinase domain mutations showed that each of the three inhibitors has different spectrums of activity, with strategically sequencing of FGFR inhibitors potentially prolonging duration of benefit from an FGFR inhibition strategy in these patients.
IDH 1/2
Isocitrate dehydrogenase 1 and 2 (IDH1/2) are enzymes which regulate metabolism, DNA repair, and epigenetic modulation (61). IDH1/2 normally catalyze the conversion of isocitrate to α-ketoglutarate. However, mutations result in neomorphic activity of IDH, leading to increased production of 2-hydroxyglutarate (2HG), a suspected oncometabolite that can lead to aberrant DNA and histone methylation and epigenetic changes (51,62,63). 2-hydroxygluatarate can be measured in serum and may have a role as a possible biomarker as significantly higher levels of 2HG have been found in patients with IDH1/2 mutations than patients with wildtype IDH1/2 (64,65). Initially discovered in hematologic malignancies, mutations in IDH1/2 occur in approximately 20–25% of IH-CCA without significant frequency in EH-CCA or gallbladder cancer. Unlike FGFR2 fusions, there appears to be no consistent report of difference in age, sex, or OS between patients with IDH mutant and IDH wild-type tumors (48).
Ivosidenib, an IDH1 inhibitor, was studied in a phase I trial of multiple solid tumors including cholangiocarcinoma, chondrosarcoma, and glioma (66). In the cholangiocarcinoma cohort (25), 6% of patients had a confirmed PR while 56% experienced stable disease; the PFS at 6 months was 40% with no dose-limiting toxicities. Adverse events included fatigue (21%), nausea (18%), vomiting (12%), diarrhea (10%). The subsequent ClarIDHy study is a randomized phase III trial of 185 patients with IDH-1 mutated, treatment-refractory cholangiocarcinoma who received either ivosidenib or placebo with crossover allowed for the placebo arm at disease progression. Preliminary data show a DCR of 53% vs. 28% for placebo and a median PFS of 2.7 vs. 1.4 months (P<0.001); no patients on the placebo arm were progression-free at 6 or 12 months. Median OS showed a trend towards benefit with ivosidenib (10.8 vs. 9.7 months, P=0.06) and all subgroups favored ivosidenib (26).
Other inhibitors of IDH1/2 are currently being evaluated in trials of IDH1 or IDH2 mutant advanced solid tumors including cholangiocarcinoma. The IDH1 inhibitor FT2012 is being studied alone and in combination with other drugs in a phase I/II trial in relapsed/refractory patients with IDH1 mutations across multiple tumor types (NCT03684811). The IDH1 inhibitor BAY1436032 has shown efficacy in AML (67) and is currently undergoing a phase I trial in advanced solid tumors (NCT02746081). Additionally, the PARP inhibitor olaparib is being studied in a phase II trial of relapsed/refractory advanced solid tumors with IDH1/2 mutations NCT03212274).
Work to identify resistance mechanisms to ivosidenib and other IDH1 inhibitors is ongoing. In the phase I study of ivosidenib in cholangiocarcinoma, 37 (59%) of patients had paired pre-treatment and post-treatment sequencing of tumor (68). Six patients, including four with stable disease and one with a partial response, developed new oncogenic mutations. These included mutations in IDH1 and IDH2 (IDH2-R172V, IDH1-R132F) and mutations in TP53, ARID1A. POLE, PIK3R1, and TBX3. Further work to define potential resistance mechanisms will be necessary to better understand optimal treatment strategies and additional targets in this patient population.
BRAF/MEK
The mitogen-activated protein kinase (MAPK)/extracellular signal-regulated kinase (ERK), or MEK pathway, is involved in cell proliferation and survival and is frequently mutated in tumorigenesis (69). One of the strongest activators of the MEK pathway is a mutation in v-Raf murine sarcoma viral oncogene homolog B (BRAF); the most common mutation in BRAF is an activating mutation resulting from glutamic acid substituting for valine at amino acid 600 (V600E) (69,70). Although common in melanoma and papillary thyroid cancer, the incidence of this mutation is BTC is low at about 1–6% with a preponderance of cases seen in IH-CCA (52,71).
The ROAR trial, a phase II basket trial of 178 patients with BRAF V600E mutations, evaluated the combination of the BRAF inhibitor dabrafenib and the MEK inhibitor trametinib across multiple diseases including 33 patients with advanced refractory BTC (27). With a median follow-up of 8 months, the ORR was 41%, with a median PFS of 7.2 months and a median OS of 11.3 months, suggesting that this may be an active regimen in this small population. A phase I/IIa basket trial in patients with relapsed/refractory solid tumors including BTC using PLX8394, an oral inhibitor of mutant and wild-type BRAF, is ongoing (NCT02428712).
MEK inhibitors have also been evaluated in patients with cholangiocarcinoma and have shown modest activity. A phase II study of the MEK inhibitor selumetinib in 28 patients with advanced BTC, including 39% of whom had received prior therapy, showed a median PFS of 3.7 months and median OS of 9.8 months with a favorable safety profile (72). The phase 1b ABC-04 study of gemcitabine/cisplatin/selumetinib showed a median PFS of 6.4 months and manageable toxicities at the established dose but the regimen had insufficient efficacy to be developed further (73).
DNA damage repair proteins
Alterations in DNA damage repair pathways have also been associated with BTC, providing a potential target for therapy. BRCA2 mutation carriers have an increased risk of BTC, with an incidence of ~4% (52). Olaparib is a PARP inhibitor approved for germline BRCA1/2 mutation carriers with breast or ovarian cancers and activity in BRCA-associated unresectable or metastatic pancreatic cancer after front-line platinum-based chemotherapy. Olaparib has demonstrated clinical activity in case reports of 2 patients with advanced BTC and a BRCA-1 and BRCA-2 mutation, respectively, although further work is needed to define any potential benefit (74,75). Mismatch repair deficiency has also been noted in BTCs, potentially identifying a subset of patients susceptible to immune checkpoint inhibition (76). Studies of PARP inhibitors alone or in combination with immune checkpoint inhibitors in platinum-sensitive BTC patients are ongoing (NCT04042831, NCT03639935).
HER2/EGFR pathway
The HER pathway consists of four receptors (HER1/EGFR, HER2, HER3, HER4) that homo- or hetero-dimerize, resulting in downstream activation of multiple pathways including the MAPK and PI3K/AKT pathways (77). Approximately 5–15% of BTC tumors are HER2-positive by IHC and/or FISH, and are more commonly expressed in gallbladder cancers and EH-CCA with no differences in expression by geographic region (78,79). HER2 expression has not been shown to have clear prognostic significance in advanced BTC (78,80).
The importance of targeting the HER2 pathway in BTC is emerging, with some retrospective data suggesting efficacy of HER2-targeted therapy in HER2 amplified tumors (81). Preliminary activity of pertuzumab + trastuzumab in HER2-positive metastatic BTC has been reported in a study of 11 patients, with 4 PRs and 3 SDs (82). The pan-HER TKI neratinib was studied in a solid tumor basket trial involving 9 patients with HER-2 mutant BTC and showed a response in 2 of these patients at 8 weeks and a median PFS of 2.8 months (83). Surprisingly, other pan-HER TKIs including lapatinib and apatinib have not shown significant efficacy in advanced BTC (84,85). In a pooled analysis of three phase I studies of patients with refractory BTC, varlitinib, a pan-HER TKI, demonstrated a PR of 27%, SD of 43%, and a DCR of 70% across 37 evaluable patients (86). Trials of varlitinib, trastuzumab plus pertuzumab, and trastuzumab emtansine in are ongoing in patients with HER2-altered BTC or in basket trials of HER2 altered solid tumors (NCT03093870, NCT03613168, NCT02693535, NCT02992340).
While epidermal growth factor receptor (EGFR) is overexpressed in 11–27% of IH-CCA, 5–20% of EH-CCA, and 1–5% of GBC (79-81,87), studies are mixed regarding EGFR expression and prognosis (79,80). However, the clinical utility of targeting this pathway has been disappointing (88). A phase III study of gemcitabine and oxaliplatin, with or without the EGFR-targeted TKI erlotinib, showed an improved response rate but no difference in PFS or OS (89). A recent meta-analysis of trials evaluating gemcitabine and oxaliplatin plus EGFR-targeted therapy showed an overall improvement in PFS with both TKIs and antibodies [hazard ratio (HR) of 0.8 (P=0.03)], although there were higher rates of adverse events and no difference in OS (90). While combining EGFR inhibitors with cytotoxic therapy should not be considered standard of care, these analyses suggest that further work to define the optimal EGFR-targeted combination or BTC subset may be warranted.
Vascular endothelial growth factor (VEGF)
VEGF signaling leads to tumorigenesis via neovascularization and cell proliferation. VEGF overexpression is common in CCA and associated with intrahepatic metastases in IH-CCA (79). Single arm studies of the humanized anti-VEGF monoclonal IgG1 antibody bevacizumab combined with chemotherapy have demonstrated a median PFS of 6–7 months (91,92) with variable OS, while a single arm phase II study combining bevacizumab with erlotinib in patients with no prior treatment for advanced disease demonstrated a high rate of disease control (12% PR, 51% SD) and a median OS of 9.9 months (93).
Small molecule TKIs directed against the VEGF pathway have also demonstrated mixed results. The randomized phase II ABC-03 study evaluated gemcitabine/cisplatin with or without cediranib in advanced BTC; no survival benefit was shown with the addition of cediranib and the rates of grade 3 or 4 toxicity were higher (94). Studies of cabozantinib, sunitinib, vandetanib, and sorafenib (with or without chemotherapy) have not demonstrated significant activity (95-100). However, a recent single-arm phase II trial of regorafenib in advanced refractory BTC showed modest activity with an objective response of 11% but a DCR of 56% and a median OS of 32 weeks (29). In addition, the combination of VEGF inhibitors and immune checkpoint inhibitors are currently being tested. A retrospective, real-world Chinese study of lenvatinib and nivolumab or pembrolizumab in 60 previously treated patients with BTC demonstrated an objective response rate of 29.4% and DCR of 86.3%, signaling synergy in targeting these two pathways simultaneously, with promising median progression-free survival of 5.0 months and median OS of 13.0 months (101).
Further work is needed to understand the optimal strategy for targeting angiogenesis in BTC. A meta-analysis of 964 patients across 7 trials showed an improvement in ORR when anti-VEGF therapy was added to chemotherapy, but did not detect a difference in PFS or OS (102). Newer agents are now being explored, including an ongoing phase III study of a combination of ramucirumab, a monoclonal antibody against VEGFR2 (NCT02520141), and apatinib, a VEGFR2 TKI, in the second line setting for IH-CCA (NCT03521219). Ramucirumab has also been evaluated with cisplatin and gemcitabine in a randomized global phase II study compared to cisplatin and gemcitabine alone, and results are awaited (NCT02711553). The combination of VEGF inhibition and immune checkpoint inhibition is also being prospectively evaluated in BTC, including lenvatinib/pembrolizumab (NCT03895970). Identifying susceptible subgroups or optimal patient populations who will benefit from antiangiogenic-based therapy will be necessary before this approach can be incorporated into routine standards of care.
PI3K/AKT/mTOR pathway
The PI3K/AKT/mTOR pathway regulates cell cycle progression, proliferation, and angiogenesis and interacts with the RAS/RAF/MEK pathway through mTOR signaling (103). PIK3CA mutations have been found in 0–6% of EH-CCA and 0–8% of IH-CCA, and in 5–15% of GBC (31,52,104), although mutations in the PI3K/AKT/mTOR pathway occur up to 40% in EH-CCA and 25% in IH-CCA (42,105). Although dysregulation of the PI3K/AKT/mTOR pathway is thought to contribute to tumor progression, no clear prognostic value of these mutations has been demonstrated in BTC (52,106-108). Preclinical studies of PI3K pathway inhibitors in BTC have led to clinical studies of both mTOR inhibitors and PI3K inhibitors in patients with BTC.
A small phase II study of everolimus monotherapy in previously treated BTC demonstrated a modest PFS of 3.2 months and OS of 7.7 months (109), while a phase II study of the same agent in the first-line setting demonstrated a PFS of 5.5 months and OS of 9.5 months, with disease control lowest for GBC (28). A phase I study of copanlisib, an PI3K inhibitor, in combination with gemcitabine and cisplatin in advanced malignancies including BTC reported a response rate of 17% in patients with BTC, although numbers were small (110). A phase II study of copanlisib (BAY 80-6946) in combination with gemcitabine and cisplatin in advanced cholangiocarcinoma is ongoing (NCT02631590).
c-MET
c-MET and its ligand hepatocyte growth factor (HGF), involved with cell proliferation, migration, and invasion (111). c-MET is overexpressed in 11–58% in IH-CCA and 0–16% in EH-CCA (80,112,113). The prognostic value of c-MET overexpression is unclear, with some studies associating c-Met overexpression with a worse overall 5-year OS (112) while another study found no association with survival (113). Studies of small molecule multikinase inhibitors that target MET have been evaluated in early phase studies in advanced BTC. A phase II study of cabozantinib, a multi-kinase inhibitor of MET, AXL, and VEGFR, demonstrated a median PFS of 1.8 months and median OS of 5.2 months in patients with previously treated advanced CCA but had high rates of grade 3/4 toxicity (114). Tivantinib, another oral c-MET inhibitor, has shown activity in combination with gemcitabine; out of 56 evaluable patients 11 (19%) patients had a PR and 26 (46%) had SD, and 10 of these patients with PR or SD had prior gemcitabine exposure (115). A phase I study of multiple tumor types treated with merestinib, a small molecule inhibitor of MET and several other kinases, showed one CR and three PRs in patients with cholangiocarcinoma (116). A randomized global phase II trial of gemcitabine and cisplatin with or without merestinib in the first line setting has completed accrual and results are awaited (NCT02711553).
Conclusions
Once considered to be three diseases under one umbrella, BTC has been shown to have heterogeneity in presentation, prognosis, and response to treatment beyond anatomic subgroups. The advent of next generation sequencing has allowed for the classification of BTC into molecular subsets with distinct genomic profiles that have influenced the evolving treatment paradigms. Many of the mutations have proven to be ripe therapeutic targets, demonstrating efficacy in the refractory setting. Thus, early tumor molecular profiling is critical for patients with newly-diagnosed advanced BTC. Studies are currently underway to evaluate the targeting of driver mutations to the front-line setting and also as maintenance after initial clinical benefit on cytotoxic therapy (Table 2); the role of these targeted therapies in the adjuvant setting remains to be determined. If targeted therapies become the preferred approach for subsets of BTCs, cumulative chemotherapy-related toxicity could be delayed or spared for many patients.
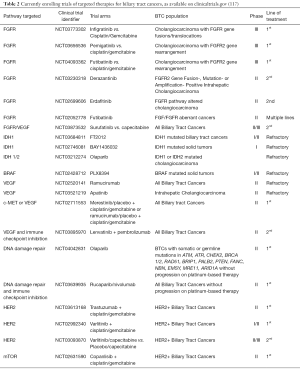
Full table
Molecular sequencing at the time of progression is also identifying mechanisms of resistance and potential therapeutic targets, as third-generation inhibitors are being developed to overcome point mutations that arise with initial targeted therapy. As this field evolves, it may be necessary to sample a tumor’s genome sequentially with either repeat tumor biopsy or liquid biopsy platforms or both. As technologies advance to more comprehensively and more economically interrogate the tumor genome and transcriptome, we anticipate the identification of additional potential therapeutic targets and mechanisms of resistance ripe for investigation. For now, we await the results of a bevy of exciting trials in the refractory and front-line metastatic setting that will hopefully redefine how we approach BTCs in the years to come.
Acknowledgments
Funding: This manuscript was funded in part through The Cholangiocarcinoma Foundation Andrea Lynn Scott Memorial Research Fellowship (L Goyal) and the NIH/NCI Cancer Center Support Grant P30 CA006927 (CS Denlinger, P Iyer).
Footnote
Conflicts of Interest: Dr. CS Denlinger has received advisory board honoraria from Astellas, Bayer, Merck, Bristol Myer Squibb, Eli Lilly & Co, EMD Serono, Exelixis, and BeiGene. Dr. Denlinger has received institutional grant support from Array BioPharma, Amgen, Advaxis, Bristol Myer Squibb, Astra Zeneca, Sanofi, BeiGene, Merrimack Pharmaceuticals, Roche/Genentech, Macrogenics, Lycera, Zymeworks, and Agios Pharmaceuticals. Dr. L Goyal is a consultant/advisory board member for Debiopharm, H3 Biomedicine, Agios Pharmaceuticals, Taiho Pharmaceuticals, QED, Klus Pharmaceuticals, and Pieris Pharmaceuticals. Dr. MH Chen has received advisory board honoraria from Bayer, Sanofi, Merck, Bristol Myer Squibb and Ono, Eli Lilly & Co, and IPSEN. Dr. P Iyer has no conflicts of interest to declare.
Ethical Statement: The authors are accountable for all aspects of the work in ensuring that questions related to the accuracy or integrity of any part of the work are appropriately investigated and resolved.
References
- Fitzmaurice C, Akinyemiju TF, Al Lami FH, et al. Global, Regional, and National Cancer Incidence, Mortality, Years of Life Lost, Years Lived With Disability, and Disability-Adjusted Life-Years for 29 Cancer Groups, 1990 to 2016: A Systematic Analysis for the Global Burden of Disease Study. JAMA Oncol 2018;4:1553-68. [Crossref] [PubMed]
- Van Dyke AL, Shiels MS, Jones GS, et al. Biliary tract cancer incidence and trends in the United States by demographic group, 1999‐2013. Cancer 2019;125:1489-98. [Crossref] [PubMed]
- Saha SK, Zhu AX, Fuchs CS, et al. Forty-Year Trends in Cholangiocarcinoma Incidence in the U.S.: Intrahepatic Disease on the Rise. Oncologist 2016;21:594-9. [Crossref] [PubMed]
- Marcano-Bonilla L, Mohamed EA, Mounajjed T, et al. Biliary tract cancers: epidemiology, molecular pathogenesis and genetic risk associations. Chin Clin Oncol 2016;5:61. [Crossref] [PubMed]
- Randi G, Malvezzi M, Levi F, et al. Epidemiology of biliary tract cancers: an update. Ann Oncol 2009;20:146-59. [Crossref] [PubMed]
- Torre LA, Siegel RL, Islami F, et al. Worldwide Burden of and Trends in Mortality From Gallbladder and Other Biliary Tract Cancers. Clin Gastroenterol Hepatol 2018;16:427-37. [Crossref] [PubMed]
- Kirstein MM, Vogel A. Epidemiology and Risk Factors of Cholangiocarcinoma. Visc Med 2016;32:395-400. [Crossref] [PubMed]
- Chaiteerakij R, Pan-ngum W, Poovorawan K, et al. Characteristics and outcomes of cholangiocarcinoma by region in Thailand: A nationwide study. World J Gastroenterol 2017;23:7160-7. [Crossref] [PubMed]
- Qian MB, Zhou XN. Human liver flukes in China and ASEAN: Time to fight together. PLoS Negl Trop Dis 2019;13:e0007214. [Crossref] [PubMed]
- Lun ZR, Gasser RB, Lai DH, et al. Clonorchiasis: a key foodborne zoonosis in China. Lancet Infect Dis 2005;5:31-41. [Crossref] [PubMed]
- Sithithaworn P, Yongvanit P, Duenngai K, et al. Roles of liver fluke infection as risk factor for cholangiocarcinoma. J Hepatobiliary Pancreat Sci 2014;21:301-8. [Crossref] [PubMed]
- Tyson GL, El-Serag HB. Risk factors for cholangiocarcinoma. Hepatology 2011;54:173-84. [Crossref] [PubMed]
- Chapman RW. Risk factors for biliary tract carcinogenesis. Ann Oncol 1999;10 Suppl 4:308-11. [Crossref] [PubMed]
- Banales JM, Cardinale V, Carpino G, et al. Cholangiocarcinoma: current knowledge and future perspectives consensus statement from the European Network for the Study of Cholangiocarcinoma (ENS-CCA). Nat Rev Gastroenterol Hepatol 2016;13:261-80. [Crossref] [PubMed]
- Raderer M, Hejna MHL, Valencak JB, et al. Two Consecutive Phase II Studies of 5-Fluorouracil/Leucovorin/Mitomycin C and of Gemcitabine in Patients with Advanced Biliary Cancer. Oncology 1999;56:177-80. [Crossref] [PubMed]
- Valle J, Wasan H, Palmer DH, et al. Cisplatin plus Gemcitabine versus Gemcitabine for Biliary Tract Cancer. N Engl J Med 2010;362:1273-81. [Crossref] [PubMed]
- Okusaka T, Nakachi K, Fukutomi A, et al. Gemcitabine alone or in combination with cisplatin in patients with biliary tract cancer: a comparative multicentre study in Japan. Br J Cancer 2010;103:469-74. [Crossref] [PubMed]
- Lamarca A, Palmer DH, Wasan HS, et al. ABC-06 | A randomised phase III, multi-centre, open-label study of active symptom control (ASC) alone or ASC with oxaliplatin / 5-FU chemotherapy (ASC+mFOLFOX) for patients (pts) with locally advanced / metastatic biliary tract cancers (ABC) previously-treated with cisplatin/gemcitabine (CisGem) chemotherapy. J Clin Oncol 2019;37:4003. [Crossref]
- Javle M, Lowery M, Shroff RT, et al. Phase II Study of BGJ398 in Patients With FGFR-Altered Advanced Cholangiocarcinoma. J Clin Oncol 2018;36:276-82. [Crossref] [PubMed]
- Mazzaferro V, El-Rayes BF. Derazantinib (ARQ 087) in advanced or inoperable FGFR2 gene fusion-positive intrahepatic cholangiocarcinoma. Br J Cancer 2019;120:165-71. [Crossref] [PubMed]
- Droz Dit Busset M, El-Rayes BF, Harris WP, et al. Efficacy of derazantinib (DZB) in patients (pts) with intrahepatic cholangiocarcinoma (iCCA) expressing FGFR2-fusion or FGFR2 mutations/amplifications. In: ESMO Congress. Barcelona; 2019.
- Park JO, Feng YH, Chen YY, et al. Updated results of a phase IIa study to evaluate the clinical efficacy and safety of erdafitinib in Asian advanced cholangiocarcinoma (CCA) patients with FGFR alterations. J Clin Oncol 2019;37:4117. [Crossref]
- Vogel A, Sahai V, Hollebecque A, et al. LBA40 - FIGHT-202: A phase II study of pemigatinib in patients (pts) with previously treated locally advanced or metastatic cholangiocarcinoma (CCA). In: ESMO Congress. Barcelona; 2019.
- Tran B, Meric-Bernstam F, Arkenau H, et al. Efficacy of TAS-120, an Irreversible Fibroblast Growth Factor Receptor Inhibitor (FGFRi), in Patients With Cholangiocarcinoma and FGFR Pathway Alterations. In: ESMO Congress [Internet]. 2018. Available online: https://academic.oup.com/annonc/article/doi/10.1093/annonc/mdy432/5200162
- Lowery MA, Burris HA, Janku F, et al. Safety and activity of ivosidenib in patients with IDH1-mutant advanced cholangiocarcinoma: a phase 1 study. Lancet Gastroenterol Hepatol 2019;4:711-20. [Crossref] [PubMed]
- Abou-Alfa G, Maraculla T, Javle M, et al. ClarIDHy: A global, phase 3, randomized, double-blind study of ivosidenib (IVO) vs placebo in patients with advanced cholangiocarcinoma (CC) with an isocitrate dehydrogenase 1 (IDH1) mutation. In: 2019 ESMO Congress. Barcelona, Spain; 2019.
- Wainberg ZA, Lassen UN, Elez E, et al. Efficacy and safety of dabrafenib (D) and trametinib (T) in patients (pts) with BRAF V600E–mutated biliary tract cancer (BTC): A cohort of the ROAR basket trial. J Clin Oncol 2019;37:187. [Crossref]
- Lau DK, Tay RY, Yeung YH, et al. Phase II study of everolimus (RAD001) monotherapy as first-line treatment in advanced biliary tract cancer with biomarker exploration: the RADiChol Study. Br J Cancer 2018;118:966-71. [Crossref] [PubMed]
- Sun W, Patel A, Normolle D, et al. A phase 2 trial of regorafenib as a single agent in patients with chemotherapy‐refractory, advanced, and metastatic biliary tract adenocarcinoma. Cancer 2019;125:902-9. [Crossref] [PubMed]
- Fujimoto A, Furuta M, Shiraishi Y, et al. Whole-genome mutational landscape of liver cancers displaying biliary phenotype reveals hepatitis impact and molecular diversity. Nat Commun 2015;6:6120. [Crossref] [PubMed]
- Zou S, Li J, Zhou H, et al. Mutational landscape of intrahepatic cholangiocarcinoma. Nat Commun 2014;5:5696. [Crossref] [PubMed]
- Jiao Y, Pawlik TM, Anders RA, et al. Exome sequencing identifies frequent inactivating mutations in BAP1, ARID1A and PBRM1 in intrahepatic cholangiocarcinomas. Nat Genet 2013;45:1470-3. [Crossref] [PubMed]
- Jusakul A, Cutcutache I, Yong CH, et al. Whole-Genome and Epigenomic Landscapes of Etiologically Distinct Subtypes of Cholangiocarcinoma. Cancer Discov 2017;7:1116-35. [Crossref] [PubMed]
- Zandvakili I, Lazaridis KN. Cell-free DNA testing: future applications in gastroenterology and hepatology. Therap Adv Gastroenterol 2019;12:1756284819841896. [Crossref] [PubMed]
- Nakamura H, Arai Y, Totoki Y, et al. Genomic spectra of biliary tract cancer. Nat Genet 2015;47:1003-10. [Crossref] [PubMed]
- Chan-On W, Nairismägi ML, Ong CK, et al. Exome sequencing identifies distinct mutational patterns in liver fluke–related and non-infection-related bile duct cancers. Nat Genet 2013;45:1474-8. [Crossref] [PubMed]
- Ikeda M, Maruki Y, Ueno M, et al. Frequency and clinicopathological characteristics of biliary tract carcinomas harboring the FGFR2-fusion gene: a prospective observational study. Available online: https://academic.oup.com/annonc/article/doi/10.1093/annonc/mdz247/5577841
- Simbolo M, Vicentini C, Ruzzenente A, et al. Genetic alterations analysis in prognostic stratified groups identified TP53 and ARID1A as poor clinical performance markers in intrahepatic cholangiocarcinoma. Sci Rep 2018;8:7119. [Crossref] [PubMed]
- Graham RP, Barr Fritcher EG, Pestova E, et al. Fibroblast growth factor receptor 2 translocations in intrahepatic cholangiocarcinoma. Hum Pathol 2014;45:1630-8. [Crossref] [PubMed]
- Jain A, Borad MJ, Kelley RK, et al. Cholangiocarcinoma With FGFR Genetic Aberrations: A Unique Clinical Phenotype. JCO Precis Oncol 2018;(2):1-12.
- Javle MM, Catenacci D, Jain A, et al. Precision medicine for gallbladder cancer using somatic copy number amplifications (SCNA) and DNA repair pathway gene alterations. J Clin Oncol 2017;35:4076. [Crossref]
42. Churi CR, Shroff R, Wang Y, et al. Mutation Profiling in Cholangiocarcinoma: Prognostic and Therapeutic Implications. Creighton C, editor. PLoS One 2014;9:e115383. - Almquist D, Javle M, Ciombor K, et al. FGFR2 fusions and its effect of patient (pt) outcomes in intrahepatic cholangiocarcinoma (iCCA). Available online: https://academic.oup.com/annonc/article/doi/10.1093/annonc/mdz247/5577841
- Wang P, Dong Q, Zhang C, et al. Mutations in isocitrate dehydrogenase 1 and 2 occur frequently in intrahepatic cholangiocarcinomas and share hypermethylation targets with glioblastomas. Oncogene 2013;32:3091-100. [Crossref] [PubMed]
- Moeini A, Sia D, Bardeesy N, et al. Molecular Pathogenesis and Targeted Therapies for Intrahepatic Cholangiocarcinoma. Clin Cancer Res 2016;22:291-300. [Crossref] [PubMed]
- Lowery MA, Ptashkin R, Jordan E, et al. Comprehensive Molecular Profiling of Intrahepatic and Extrahepatic Cholangiocarcinomas: Potential Targets for Intervention. Clin Cancer Res 2018;24:4154-61. [Crossref] [PubMed]
- Zhu AX, Borger DR, Kim Y, et al. Genomic Profiling of Intrahepatic Cholangiocarcinoma: Refining Prognosis and Identifying Therapeutic Targets. Ann Surg Oncol 2014;21:3827-34. [Crossref] [PubMed]
- Goyal L, Govindan A, Sheth RA, et al. Prognosis and Clinicopathologic Features of Patients With Advanced Stage Isocitrate Dehydrogenase (IDH) Mutant and IDH Wild-Type Intrahepatic Cholangiocarcinoma. Oncologist 2015;20:1019-27. [Crossref] [PubMed]
- Turner N, Grose R. Fibroblast growth factor signalling: from development to cancer. Nat Rev Cancer 2010;10:116-29. [Crossref] [PubMed]
- Goyal L, Saha SK, Liu LY, et al. Polyclonal Secondary FGFR2 Mutations Drive Acquired Resistance to FGFR Inhibition in Patients with FGFR2 Fusion–Positive Cholangiocarcinoma. Cancer Discov 2017;7:252-63. [Crossref] [PubMed]
- Jain A, Javle M. Molecular profiling of biliary tract cancer: a target rich disease. J Gastrointest Oncol 2016;7:797-803. [Crossref] [PubMed]
- Valle JW, Lamarca A, Goyal L, et al. New Horizons for Precision Medicine in Biliary Tract Cancers. Cancer Discov 2017;7:943-62. [Crossref] [PubMed]
- Wu YM, Su F, Kalyana-Sundaram S, et al. Identification of Targetable FGFR Gene Fusions in Diverse Cancers. Cancer Discov 2013;3:636-47. [Crossref] [PubMed]
- Arai Y, Totoki Y, Hosoda F, et al. Fibroblast growth factor receptor 2 tyrosine kinase fusions define a unique molecular subtype of cholangiocarcinoma. Hepatology 2014;59:1427-34. [Crossref] [PubMed]
- Goyal L, Liu LY, Lennerz JK, et al. Abstract LB-092: TAS120, a covalently-binding FGFR inhibitor (FGFRi), overcomes resistance to BGJ398 in patients with FGFR2 fusion positive cholangiocarcinoma. Cancer Res 2018;78:LB-092.
- Voss MH, Hierro C, Heist RS, et al. Debio 1347, an oral FGFR inhibitor: Results from a first-in-human, phase I dose-escalation study in patients with FGFR genomically activated advanced solid tumors. J Clin Oncol 2017;35:2500. [Crossref]
- Chen YY, Park JO, Su WC, et al. Preliminary results of a ph2a study to evaluate the clinical efficacy and safety of erdafitinib in Asian patients with biomarker-selected advanced cholangiocarcinoma (CCA). Ann Oncol 2018. Available online: https://academic.oup.com/annonc/article/doi/10.1093/annonc/mdy282.008/5140646
- Byron SA, Chen H, Wortmann A, et al. The N550K/H mutations in FGFR2 confer differential resistance to PD173074, dovitinib, and ponatinib ATP-competitive inhibitors. Neoplasia 2013;15:975-88. [Crossref] [PubMed]
- Sootome H, Fujioka Y, Miura A, et al. Abstract A271: TAS-120, an irreversible FGFR inhibitor, was effective in tumors harboring FGFR mutations, refractory or resistant to ATP competitive inhibitors. In: Therapeutic Agents: Small Molecule Kinase Inhibitors [Internet]. American Association for Cancer Research; 2013 [cited 2019 Jul 20]. p. A271–A271. Available online: http://mct.aacrjournals.org/lookup/doi/10.1158/1535-7163.TARG-13-A271
- Goyal L, Shi L, Liu LY, et al. TAS-120 Overcomes Resistance to ATP-Competitive FGFR Inhibitors in Patients with FGFR2 Fusion-Positive Intrahepatic Cholangiocarcinoma. Cancer Discov 2019;9:1064-79. [Crossref] [PubMed]
- Molenaar RJ, Maciejewski JP, Wilmink JW, et al. Wild-type and mutated IDH1/2 enzymes and therapy responses. Oncogene 2018;37:1949-60. [Crossref] [PubMed]
- Fujii T, Khawaja MR, DiNardo CD, et al. Targeting isocitrate dehydrogenase (IDH) in cancer. Discov Med 2016;21:373-80. [PubMed]
- Zhang X, Miao R, Liu T, et al. IDH1 as a frequently mutated gene has potential effect on exosomes releasement by epigenetically regulating P2RX7 in intrahepatic cholangiocarcinoma. Biomed Pharmacother 2019;113:108774. [Crossref] [PubMed]
- Delahousse J, Verlingue L, Broutin S, et al. Circulating oncometabolite D-2-hydroxyglutarate enantiomer is a surrogate marker of isocitrate dehydrogenase–mutated intrahepatic cholangiocarcinomas. Eur J Cancer 2018;90:83-91. [Crossref] [PubMed]
- Borger DR, Goyal L, Yau T, et al. Circulating Oncometabolite 2-Hydroxyglutarate Is a Potential Surrogate Biomarker in Patients with Isocitrate Dehydrogenase-Mutant Intrahepatic Cholangiocarcinoma. Clin Cancer Res 2014;20:1884-90. [Crossref] [PubMed]
- Fan B, Mellinghoff IK, Wen PY, et al. Pharmacokinetics/pharmacodynamics (PK/PD) of ivosidenib in patients with IDH1-mutant advanced solid tumors from a phase 1 study. J Clin Oncol 2018;36:2577. [Crossref]
- Heuser M, Herbst L, Pusch S, et al. Pan-Mutant-IDH1 Inhibitor Bay-1436032 Is Highly Effective Against Human IDH1 Mutant Acute Myeloid Leukemia In Vivo. Blood 2016. Available online: http://www.bloodjournal.org/content/128/22/745
- Lowery MA, Abou-Alfa GK, Burris HA, et al. Phase I study of AG-120, an IDH1 mutant enzyme inhibitor: Results from the cholangiocarcinoma dose escalation and expansion cohorts. J Clin Oncol 2017;35:4015. [Crossref]
- Cantwell-Dorris ER, O’Leary JJ, Sheils OM. BRAFV600E: implications for carcinogenesis and molecular therapy. Mol Cancer Ther 2011;10:385-94. [Crossref] [PubMed]
- Wan PTC, Garnett MJ, Roe SM, et al. Mechanism of activation of the RAF-ERK signaling pathway by oncogenic mutations of B-RAF. Cell 2004;116:855-67. [Crossref] [PubMed]
- Simbolo M, Fassan M, Ruzzenente A, et al. Multigene mutational profiling of cholangiocarcinomas identifies actionable molecular subgroups. Oncotarget 2014;5:2839-52. [Crossref] [PubMed]
- Bekaii-Saab T, Phelps MA, Li X, et al. Multi-institutional phase II study of selumetinib in patients with metastatic biliary cancers. J Clin Oncol 2011;29:2357-63. [Crossref] [PubMed]
- Bridgewater JA, Beare S, Dymphna L, et al. ABC-04: A phase 1b trial of cisplatin, gemcitabine, and selumetinib in patients with advanced biliary tract cancer. J Clin Oncol 2014;32:4090. [Crossref]
- Xie Y, Jiang Y, Yang XB, et al. Response of BRCA1-mutated gallbladder cancer to olaparib: A case report. World J Gastroenterol 2016;22:10254-9. [Crossref] [PubMed]
- Cheng Y, Zhang J. Treatment with olaparib monotherapy for BRCA2-mutated refractory intrahepatic cholangiocarcinoma: a case report. Onco Targets Ther 2018;11:5957-62. [Crossref] [PubMed]
- Silva VW, Askan G, Daniel TD, et al. Biliary carcinomas: pathology and the role of DNA mismatch repair deficiency. Chin Clin Oncol 2016;5:62. [Crossref] [PubMed]
- Moasser MM. The oncogene HER2: its signaling and transforming functions and its role in human cancer pathogenesis. Oncogene 2007;26:6469-87. [Crossref] [PubMed]
- Galdy S, Lamarca A, McNamara MG, et al. HER2/HER3 pathway in biliary tract malignancies; systematic review and meta-analysis: a potential therapeutic target? Cancer Metastasis Rev 2017;36:141-57. [Crossref] [PubMed]
- Yoshikawa D, Ojima H, Iwasaki M, et al. Clinicopathological and prognostic significance of EGFR, VEGF, and HER2 expression in cholangiocarcinoma. Br J Cancer 2008;98:418-25. [Crossref] [PubMed]
- Nakazawa K, Dobashi Y, Suzuki S, et al. Amplification and overexpression of c-erbB-2,epidermal growth factor receptor, and c-met in biliary tract cancers. J Pathol 2005;206:356-65. [Crossref] [PubMed]
- Javle M, Churi C, Kang HC, et al. HER2/neu-directed therapy for biliary tract cancer. J Hematol Oncol 2015;8:58. [Crossref] [PubMed]
- Lee SM, Falzon M, Blackhall F, et al. Randomized Prospective Biomarker Trial of ERCC1 for Comparing Platinum and Nonplatinum Therapy in Advanced Non-Small-Cell Lung Cancer: ERCC1 Trial (ET). J Clin Oncol 2017;35:402-11. [Crossref] [PubMed]
- Hyman DM, Piha-Paul SA, Won H, et al. HER kinase inhibition in patients with HER2- and HER3-mutantcancers. Nature 2018;554:189. [Crossref] [PubMed]
- Ramanathan RK, Belani CP, Singh DA, et al. A phase II study of lapatinib in patients with advanced biliary tree and hepatocellular cancer. Cancer Chemother Pharmacol 2009;64:777-83. [Crossref] [PubMed]
- Moehler M, Maderer A, Ehrlich A, et al. Safety and efficacy of afatinib as add-on to standard therapy of gemcitabine/cisplatin in chemotherapy-naive patients with advanced biliary tract cancer: an open-label, phase I trial with an extensive biomarker program. BMC Cancer 2019;19:55. [Crossref] [PubMed]
- Tan AC, Oh DY, Chao Y, et al. Efficacy and safety of varlitinib, a reversible pan-HER tyrosine kinase inhibitor, in combination with platinum-based regimens in biliary tract cancers: a pooled analysis from three phase 1 studies. Hepatobiliary Surg Nutr 2019;8:AB062. [Crossref]
- Chong DQ, Zhu AX. The landscape of targeted therapies for cholangiocarcinoma: current status and emerging targets. Oncotarget 2016;7:46750-67. [Crossref] [PubMed]
- Malka D, Cervera P, Foulon S, et al. Gemcitabine and oxaliplatin with or without cetuximab in advanced biliary-tract cancer (BINGO): a randomised, open-label, non-comparative phase 2 trial. Lancet Oncol 2014;15:819-28. [Crossref] [PubMed]
- Lee J, Park SH, Chang HM, et al. Gemcitabine and oxaliplatin with or without erlotinib in advanced biliary-tract cancer: a multicentre, open-label, randomised, phase 3 study. Lancet Oncol 2012;13:e49. [Crossref] [PubMed]
- Cai W, Yuan Y, Ge W, et al. EGFR Target Therapy Combined with Gemox for Advanced Biliary Tract Cancers: a Meta-analysis based on RCTs. J Cancer 2018;9:1476-85. [Crossref] [PubMed]
- Iyer RV, Groman A, Ma WW, et al. Gemcitabine (G), capecitabine (C) and bevacizumab (BV) in patients with advanced biliary cancers (ABC): final results of a multicenter phase II study. J Clin Oncol 2015;33:4078. [Crossref]
- Zhu AX, Meyerhardt JA, Blaszkowsky LS, et al. Efficacy and safety of gemcitabine, oxaliplatin, and bevacizumab in advanced biliary-tract cancers and correlation of changes in 18-fluorodeoxyglucose PET with clinical outcome: a phase 2 study. Lancet Oncol 2010;11:48-54. [Crossref] [PubMed]
- Lubner SJ, Mahoney MR, Kolesar JL, et al. Report of a Multicenter Phase II Trial Testing a Combination of Biweekly Bevacizumab and Daily Erlotinib in Patients With Unresectable Biliary Cancer: A Phase II Consortium Study. J Clin Oncol 2010;28:3491. [Crossref] [PubMed]
- Valle JW, Wasan H, Lopes A, et al. Cediranib or placebo in combination with cisplatin and gemcitabine chemotherapy for patients with advanced biliary tract cancer (ABC-03): a randomised phase 2 trial. Lancet Oncol 2015;16:967-78. [Crossref] [PubMed]
- El-Khoueiry AB, Rankin C, Siegel AB, et al. S0941: a phase 2 SWOG study of sorafenib and erlotinib in patients with advanced gallbladder carcinoma or cholangiocarcinoma. Br J Cancer 2014;110:882-7. [Crossref] [PubMed]
- Moehler M, Maderer A, Schimanski C, et al. Gemcitabine plus sorafenib versus gemcitabine alone in advanced biliary tract cancer: A double-blind placebo-controlled multicentre phase II AIO study with biomarker and serum programme. Eur J Cancer 2014;50:3125-35. [Crossref] [PubMed]
- Bengala C, Bertolini F, Malavasi N, et al. Sorafenib in patients with advanced biliary tract carcinoma: a phase II trial. Br J Cancer 2010;102:68-72. [Crossref] [PubMed]
- Lee JK, Capanu M, O’Reilly EM, et al. A phase II study of gemcitabine and cisplatin plus sorafenib in patients with advanced biliary adenocarcinomas. Br J Cancer 2013;109:915-9. [Crossref] [PubMed]
- Yi JH, Thongprasert S, Lee J, et al. A phase II study of sunitinib as a second-line treatment in advanced biliary tract carcinoma: A multicentre, multinational study. Eur J Cancer 2012;48:196-201. [Crossref] [PubMed]
- Santoro A, Gebbia V, Pressiani T, et al. A randomized, multicenter, phase II study of vandetanib monotherapy versus vandetanib in combination with gemcitabine versus gemcitabine plus placebo in subjects with advanced biliary tract cancer: the VanGogh study. Ann Oncol 2015;26:542-7. [Crossref] [PubMed]
- Lin J, Yang X, Zhao S, et al. Lenvatinib plus PD-1 blockade in advanced bile tract carcinoma. In: ESMO Congress 2019. Available online: https://academic.oup.com/annonc/article/doi/10.1093/annonc/mdz253/5578113
- Zhuang X, Xiao Y, Tan L, et al. Efficacy and safety of chemotherapy with or without targeted therapy in biliary tract cancer: A meta-analysis of 7 randomized controlled trials. J Huazhong Univ Sci Technolog Med Sci 2017;37:172-8. [Crossref] [PubMed]
- Wu CE, Chen MH, Yeh CN. mTOR Inhibitors in Advanced Biliary Tract Cancers. Int J Mol Sci 2019. [Crossref] [PubMed]
- Deshpande V, Nduaguba A, Zimmerman SM, et al. Mutational profiling reveals PIK3CA mutations in gallbladder carcinoma. BMC Cancer 2011;11:60. [Crossref] [PubMed]
- Bogenberger JM, DeLeon TT, Arora M, et al. Emerging role of precision medicine in biliary tract cancers. npj Precis Oncol 2018;2:21.
- Herberger B, Puhalla H, Lehnert M, et al. Activated Mammalian Target of Rapamycin Is an Adverse Prognostic Factor in Patients with Biliary Tract Adenocarcinoma. Clin Cancer Res 2007;13:4795-9. [Crossref] [PubMed]
- Chung JY, Hong SM, Choi BY, et al. The expression of phospho-AKT, phospho-mTOR, and PTEN in extrahepatic cholangiocarcinoma. Clin Cancer Res 2009;15:660-7. [Crossref] [PubMed]
- Lee D, Do IG, Choi K, et al. The expression of phospho-AKT1 and phospho-MTOR is associated with a favorable prognosis independent of PTEN expression in intrahepatic cholangiocarcinomas. Mod Pathol 2012;25:131-9. [Crossref] [PubMed]
- Buzzoni R, Pusceddu S, Bajetta E, et al. Activity and safety of RAD001 (everolimus) in patients affected by biliary tract cancer progressing after prior chemotherapy: a phase II ITMO study. Ann Oncol 2014;25:1597-603. [Crossref] [PubMed]
- Kim RD, Alberts SR, Peña C, et al. Phase I dose-escalation study of copanlisib in combination with gemcitabine or cisplatin plus gemcitabine in patients with advanced cancer. Br J Cancer 2018;118:462-70. [Crossref] [PubMed]
- Peruzzi B, Bottaro DP. Targeting the c-Met Signaling Pathway in Cancer. Clin Cancer Res 2006;12:3657-60. [Crossref] [PubMed]
- Miyamoto M, Ojima H, Iwasaki M, et al. Prognostic significance of overexpression of c-Met oncoprotein in cholangiocarcinoma. Br J Cancer 2011;105:131-8. [Crossref] [PubMed]
- Heo MH, Kim HK, Lee H, et al. The Clinical Impact of c-MET Over-Expression in Advanced Biliary Tract Cancer (BTC). J Cancer 2017;8:1395-9. [Crossref] [PubMed]
- Goyal L, Zheng H, Yurgelun MB, et al. A phase 2 and biomarker study of cabozantinib in patients with advanced cholangiocarcinoma. Cancer 2017;123:1979-88. [Crossref] [PubMed]
- Pant S, Saleh M, Bendell J, et al. A phase I dose escalation study of oral c-MET inhibitor tivantinib (ARQ 197) in combination with gemcitabine in patients with solid tumors. Ann Oncol 2014;25:1416-21. [Crossref] [PubMed]
- He AR, Cohen RB, Denlinger CS, et al. First-in-Human Phase I Study of Merestinib, an Oral Multikinase Inhibitor, in Patients with Advanced Cancer. Oncologist 2019;24:e930-42. [Crossref] [PubMed]
- Home - ClinicalTrials.gov [Internet]. [cited 2019 Oct 9]. Available online: https://clinicaltrials.gov/ct2/home