Anaplastic lymphoma kinase inhibitors in brain metastases from ALK+ non-small cell lung cancer: hitting the target even in the CNS
Introduction
The diagnostic and therapeutic approach to patients with non-small cell lung cancer (NSCLC) has undergone a paradigm shift since 2003. Increasingly NSCLC is classified by the presence or absence of specific genomic alterations, several of which have immediate therapeutic implications. Rearrangements involving the anaplastic lymphoma kinase (ALK) gene were first appreciated as potent oncogenic drivers in 2007, spawning the development of ALK tyrosine kinase inhibitors (TKIs) (1,2). The clinicopathologic features of ALK-rearranged (ALK+) NSCLC are well described, and ALK+ disease is thought to represent 4-7% of all NSCLC (3-5). The clinical benefit and feasibility of targeting ALK was demonstrated first with the multitargeted TKI crizotinib, and subsequently confirmed in phase II and III trials (6-9). Second generation ALK inhibitors (ALK-i) may offer further improvements in overall response rate (ORR), median progression free survival (PFS), and overall survival (OS), and phase III trials are ongoing (10,11).
Increasing clinical experience and improved survival durations have led to the appreciation of failure patterns in patients treated with ALK-i. Understanding the landscape of acquired resistance to ALK-i has improved, but this has been almost exclusively studied from progression biopsies in extra-cranial disease sites. Patients with CNS disease represent a disproportionate minority in clinical trials despite the relative frequency of brain metastases in NSCLC (12,13). In unselected surgical series the frequency of ALK+ NSCLC approaches 3% in resected brain metastases, approaching the frequency across all NSCLC (14). The ability of ALK-directed therapies to control and prevent the development of CNS metastases remains incompletely studied, with early reports suggesting inefficient cerebrospinal fluid penetration for crizotinib (15). In the following review we discuss the CNS activity of FDA-approved and investigational ALK-directed therapies. Important future directions and correlative studies needed to refine the use of ALK-i in ALK+ patient with CNS disease are highlighted.
Crizotinib
Crizotinib is a small molecule TKI with activity against the receptor tyrosine kinases (RTK) ALK, ROS1, and MET (16-18). In pre-clinical ALK+ NSCLC cell line models the IC50 for crizotinib is 60-120 nM, well below the median steady state plasma concentration of 570 nM/L (274 ng/mL) achieved with the approved 250 mg twice daily oral dosing (Table 1) (19,29). Anecdotal reports have suggested a poor CSF penetration with a CSF concentration of only 1.4 nM/L suggesting a very poor CSF-to-plasma concentration ratio (Table 1) (15). Despite the low CSF-to-plasma ratio significant CNS radiographic responses to crizotinib have been described, suggesting factors beyond pharmacokinetic resistance alone.
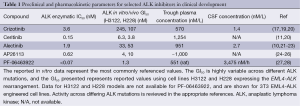
Full table
A recent retrospective analysis focusing on crizotinib in ALK+ patients with brain metastases who were enrolled in the PROFILE 1005 and 1007 trials highlights broader CNS TKI issues (13). Among the 888 patients in the pooled PROFILE 1005 and 1007 studies, 275 were known to have brain metastasis (BM) at study entry (109 untreated, 166 previously treated). The intracranial response rate was 18% in untreated BM and 33% in previously treated BM, significantly lower than the over 50% systemic ORR (13). Interestingly, the median intracranial time to progression (TTP) was nearly doubled in patients whose BM were treated prior to crizotinib initiation (7.0 vs. 13.2 months). Further, among the 275 patients with BM at study entry, the CNS was a site of progressive disease (PD) in 70% of cases of PD on crizotinib (13). This series confirms the clinical observation that the CNS is a common site of progression in ALK+ NSCLC, and is hypothesis generating for future studies and comparisons amongst ALK TKIs. Should all patients undergo CNS-directed therapies such as whole brain radiotherapy (WBRT) or stereotactic brain radiotherapy (SBRT) prior to or at progression on crizotinib? There is a strong suggestion of added benefit to continuing crizotinib after disease progression (30,31). However, in the phase II crizotinib trial the most common site for single organ PD was the CNS (32). While crizotinib has demonstrated CNS activity, next-generation ALK-i has demonstrated further improvements and is discussed below.
Ceritinib
The second generation ALK-i ceritinib (LDK378) is a potent ATP-competitive inhibitor with increased activity against common ALK point mutations including L1196M, G1269A, S1206Y, and I1171T (20,33,34). Although less active against the uncommon ALK alterations C1156Y, L1152P, G1202R, F1174C and 1151T-ins ceritinib demonstrated approximately 20-fold greater efficiency in ALK+ NSCLC cell lines and prolonged activity in xenograft models (Table 1) (20,35). Phase I testing determined a plasma CMAX 800±205 ng/mL at a dose of 750 mg orally once per day (11). The activity of ceritinib in ALK+ NSCLC has been confirmed in phase I and II testing with ORR of 58% in patients receiving at least 400 mg daily, and 56% in the 80 patients previously treated with crizotinib (11). In the 34 crizotinib-naive patients who received at least 400 mg the ORR was 62%. Among the 114 ALK+ NSCLC patients receiving at least 400 mg daily the median PFS was 7.0 months, and was similar (6.9 months) in the crizotinib resistant subgroup (n=80) (11). Based on this data ceritinib was approved in April 2014 for ALK+ NSCLC patients who progressed or were intolerant to crizotinib. Subsequently, the European Medicines Agency (EMA) has recommended ceritinib for approval in Europe in March 2015.
The CNS activity of ceritinib is less well studied, and CSF measurements are not available (Table 1). However, 64 (49%) patients in the phase I ceritinib study had BM, and in the updated presentation the ORR was 54% in 124 patients with BM at study entry (36). Further, the ORR was 69.2% in ALK-i naive BM and 50.0% in patients previously exposed to ALK-directed therapy. Overall, the median PFS for patients with BM at entry was 6.9 months, not statistically different from the overall study population (37). The improved CNS activity likely reflects the improved chemical potency and/or improved CNS penetration. Routine CSF sampling can be cumbersome to incorporate into early phase clinical trials, but provides important details needed to refine ALK-directed therapy. Further, direct comparisons across ALK-i in terms of CNS activity are confounded by a lack of CSF pharmacokinetic parameters.
Alectinib
Alectinib (CH5424802, RO5424802) is another highly selective orally available ALK-i with favorable preclinical studies in ALK-driven cancer models. Alectinib demonstrates potent ALK inhibition with an in vitro kinase IC50 of 1.9 nM, and is also active against the F1174L, R1275Q, and gatekeeper L1196M ALK mutations (21-23). The clinical activity of alectinib was demonstrated in a phase I-II studies at doses of 300 and 600 mg twice daily. In the phase I study of crizotinib naive patients receiving alectinib 300 mg twice daily the plasma trough concentration is 463±369 ng/mL with a CMAX of 575±322 ng/mL, well above the levels needed for ALK inhibition (Table 1) (10). Of the 46 patients in the phase II portion of the alectinib trial the response rate was 93.5% with treatment duration over 7 months (10). Over 30% (33%, n=15) of the 46 patients in the phase II portion had known BM. Although 26% (n=12) with BM had undergone prior radiation, disease control in the CNS was favorable, with a median of 6.5 months in the original study. Subsequently, the activity of alecitnib in crizotinib resistant or intolerant patients has been confirmed in a phase 1/2 study (38). Twenty-one patients enrolled in this trial had BM at study entry, and the response rate in the CNS was 52% (n=11) across all CNS subgroups. In the four patients with no prior CNS radiation there two complete responses, one partial response, and one patient with stable disease. Paired steady state and CSF samples from alectinib at 600 and 900 mg twice daily demonstrate a CSF trough concentration of about 2.7 nM/L for the phase II dose of 600 mg orally twice per day (38). In animal models alectinib reaches the cerebral hemispheres and cerebellum at tissue concentrations comparable to the level in plasma (39).
The study by Gadgeel and colleagues raises several important considerations for the management of ALK+ NSCLC with CNS metastases. The response assessment in the CNS used standard RECIST criteria, in which only nine patients had measurable CNS lesions at baseline, thereby limiting the conclusions due to small sample size. The problems with RECIST evaluation of CNS lesions is further highlighted by an interesting patient in the phase I alectinib study. A patient with systemic partial response developed RECIST progression in a previously SBRT-treated occipital metastasis (40% enlargement) in the context of response in the two other known CNS lesions. Due to isolated progression this patient was taken for surgical resection with pathological examination indicating the lesion was entirely necrotic, with no viable tumor (38). Thus, this case represents pseudoprogression, possibly related to the use of RECIST, rather than a CNS specific response assessment system such as the RANO criteria (40-42). Overall, alectinib demonstrates CNS activity in both crizotinib naive and crizotinib resistant ALK+ NSCLC. Numerically the CNS response rates are comparable to ceritinib and more rigorously studied in the case of alectinib. However, the small sample sizes, use of imprecise assessment tools (RECIST), and relatively short follow up duration limit direct comparisons and conclusions.
AP26113 and PF-06463922
AP26113 is a next-generation ALK and EGFR (mutant EGFR only) inhibitor with activity across all known crizotinib resistant ALK mutations. Preclinical studies have identified potent ALK inhibition with sub-nanomolar in vitro kinase IC50 and achievable plasma concentrations well above the GI50 for several cell lines models (Table 1) (24-26). Early clinical activity is comparable to alectinib, and among 72 evaluable ALK+ NSCLC the response rate is 72% (n=52) with a median response duration of 49 weeks (43). A similar response rate of 69% and median PFS of 47.3 weeks was seen in the ALK+ NSCLC with prior crizotinib therapy (n=65). In the 14 patients with untreated or progressing BM the response rate was 71% (n=10) with four complete CNS responses (43). The CNS responses occurred almost exclusively in patients at the 180 mg daily dosing, and have not yet been confirmed at the 90 mg daily dose moving forward in phase II testing. The promising phase I-II results led the FDA to assign AP26113 breakthrough designation in October 2014. Further studies including the phase II ALTA trial in refractory ALK+ NSCLC are ongoing and will provide further data on optimal dose schedule (90 mg daily or 90 mg lead in followed by 180 mg daily) as well as clarify CNS activity.
PF-06463922 is another next-generation ALK-i structurally engineered to have improved CNS penetration. The p-glycoprotein (Pgp) mediated efflux mechanism is a known barrier to achieving optimial CNS penetration and optimal CSF to free plasma ratios (44,45). The macrocyclic chemical structure, lipophilicity, relatively lower molecular weight (406.4 g/M), and area under the curve ratio of CSF to free plasma of 0.31 demonstrated favorable CNS penetration with a PF-06463922 (27). By way of comparison, the CSF to plasma ratio is 0.0026 for crizotinib (plasma concentration, 273 ng/mL; CSF, 0.616 ng/mL) (15). Further suggestion of CNS penetration can be extrapolated from the activity of PF-06463922 in the transgenic FIG-ROS1-driven glioblastoma mouse model where significant tumor regression has been shown (28). Beyond the CNS PF-06463922, like AP26113 is a potent ALK-i with promising preclinical efficacy against the gatekeeper L1196M ALK mutation (Table 1) (28,46). Based on promising pre-clinical systemic and CNS activity PF-06463922 is currently in early phase clinical trials in ALK+ NSCLC.
Conclusions
The biologic understanding and therapeutic options in NSCLC have changed drastically in the last 10 years. The identification of a subset of NSCLC driven by ALK-rearrangements has led to the rapid development and approval of ALK-directed therapies that have already drastically improved patient outcomes compared to chemotherapy (8,9). Despite improved outcomes, therapy is not curative and resistance universally develops, with the CNS as the most common site of PD. Not surprisingly, the CNS activity of ALK-i tends to parallel the chemical potency of the compound. Although incompletely studied, the idea that in the event of equal CNS pharmacokinetic parameters, the more potent inhibitor is likely to have improved clinical activity appears to bear out in early clinical investigations. The next-generation ALK-i has improved CNS activity over crizotinib but several important issues warrant consideration moving forward.
Increasingly, whether through tissue or blood-based assays, progression biopsies are informing subsequent therapeutic choices. The current next-generation inhibitors have highly variable inhibitory activity across differing ALK mutations. With the anticipated approval of multiple next-generation ALK-i will the choice of therapy, including BM, be based on pharmacokinetic (PK) parameters in the observed resistance mutation? There is a further paucity of data studying whether the molecular determinants of ALK-i resistance are the same in CNS and extracranial disease. Increasing use of surgical resection, and possible CSF sampling, may help to answer these questions.
Similarly, the current criteria used to assess CNS response in solid tumors are imperfect. The proposed Response Assessment in Neuro-Oncology (RANO) group criteria accounts for pseudoprogression resulting from radiation necrosis within 3 months of radiotherapy completion in primary CNS malignancies such a glioblastoma multiforme (40). However, neither RECIST nor the RANO criteria outline pseudoprogression determination in non-primary CNS solid tumors such as NSCLC. We expect that with the CNS activity of next-generation ALK-i the incidence of pseudoprogression will increase, and further diagnostic modalities (steroid challenge, short interval repeat MRI, MR spectroscopy, and surgical biopsy/resection) will need to be explored prior to removing a potentially active ALK-i from a given patients’ therapy (47).
Finally, the optimal sequence of ALK-i remains to be determined. Next-generation agents such as alectinib can salvage CNS disease in patients treated with both crizotinib and ceritinib (48). Larger head to head trials such as the phase III ALEX trial (alectinib vs. crizotinib) will directly investigate PFS in the CNS and may provide further information to inform treatment decisions for ALK+ patients with BM. Overall, the outcomes for ALK+ with CNS disease are expected to continue to improve with the introduction of increasingly effective therapies. Here we have reviewed the current data in ALK+ NSCLC with CNS disease and suggested several CNS-specific considerations that are important to determine the optimal management of BM moving forward.
Acknowledgements
Disclosure: The authors declare no conflict of interest.
References
- Soda M, Choi YL, Enomoto M, et al. Identification of the transforming EML4-ALK fusion gene in non-small-cell lung cancer. Nature 2007;448:561-6. [PubMed]
- Ou SH, Bartlett CH, Mino-Kenudson M, et al. Crizotinib for the treatment of ALK-rearranged non-small cell lung cancer: a success story to usher in the second decade of molecular targeted therapy in oncology. Oncologist 2012;17:1351-75. [PubMed]
- Rodig SJ, Mino-Kenudson M, Dacic S, et al. Unique clinicopathologic features characterize ALK-rearranged lung adenocarcinoma in the western population. Clin Cancer Res 2009;15:5216-23. [PubMed]
- Shaw AT, Yeap BY, Mino-Kenudson M, et al. Clinical features and outcome of patients with non-small-cell lung cancer who harbor EML4-ALK. J Clin Oncol 2009;27:4247-53. [PubMed]
- Inamura K, Takeuchi K, Togashi Y, et al. EML4-ALK fusion is linked to histological characteristics in a subset of lung cancers. J Thorac Oncol 2008;3:13-7. [PubMed]
- Kwak EL, Bang YJ, Camidge DR, et al. Anaplastic lymphoma kinase inhibition in non-small-cell lung cancer. N Engl J Med 2010;363:1693-703. [PubMed]
- Camidge DR, Bang YJ, Kwak EL, et al. Activity and safety of crizotinib in patients with ALK-positive non-small-cell lung cancer: updated results from a phase 1 study. Lancet Oncol 2012;13:1011-9. [PubMed]
- Shaw AT, Kim DW, Nakagawa K, et al. Crizotinib versus chemotherapy in advanced ALK-positive lung cancer. N Engl J Med 2013;368:2385-94. [PubMed]
- Solomon BJ, Mok T, Kim DW, et al. First-line crizotinib versus chemotherapy in ALK-positive lung cancer. N Engl J Med 2014;371:2167-77. [PubMed]
- Seto T, Kiura K, Nishio M, et al. CH5424802 (RO5424802) for patients with ALK-rearranged advanced non-small-cell lung cancer (AF-001JP study): a single-arm, open-label, phase 1-2 study. Lancet Oncol 2013;14:590-8. [PubMed]
- Shaw AT, Kim DW, Mehra R, et al. Ceritinib in ALK-rearranged non-small-cell lung cancer. N Engl J Med 2014;370:1189-97. [PubMed]
- Heon S, Yeap BY, Britt GJ, et al. Development of central nervous system metastases in patients with advanced non-small cell lung cancer and somatic EGFR mutations treated with gefitinib or erlotinib. Clin Cancer Res 2010;16:5873-82. [PubMed]
- Costa DB, Shaw AT, Ou SH, et al. Clinical Experience With Crizotinib in Patients With Advanced ALK-Rearranged Non-Small-Cell Lung Cancer and Brain Metastases. J Clin Oncol 2015;33:1881-8. [PubMed]
- Preusser M, Berghoff AS, Ilhan-Mutlu A, et al. ALK gene translocations and amplifications in brain metastases of non-small cell lung cancer. Lung Cancer 2013;80:278-83. [PubMed]
- Costa DB, Kobayashi S, Pandya SS, et al. CSF concentration of the anaplastic lymphoma kinase inhibitor crizotinib. J Clin Oncol 2011;29:e443-5. [PubMed]
- Christensen JG, Zou HY, Arango ME, et al. Cytoreductive antitumor activity of PF-2341066, a novel inhibitor of anaplastic lymphoma kinase and c-Met, in experimental models of anaplastic large-cell lymphoma. Mol Cancer Ther 2007;6:3314-22. [PubMed]
- Zou HY, Li Q, Lee JH, et al. An orally available small-molecule inhibitor of c-Met, PF-2341066, exhibits cytoreductive antitumor efficacy through antiproliferative and antiangiogenic mechanisms. Cancer Res 2007;67:4408-17. [PubMed]
- McDermott U, Iafrate AJ, Gray NS, et al. Genomic alterations of anaplastic lymphoma kinase may sensitize tumors to anaplastic lymphoma kinase inhibitors. Cancer Res 2008;68:3389-95. [PubMed]
- Tan W, Wilner KD, Bang Y, et al. Pharmacokinetics (PK) of PF-02341066, a dual ALK/MET inhibitor after multiple oral doses to advanced cancer patients. J Clin Oncol 2010;28:abstr 2596.
- Friboulet L, Li N, Katayama R, et al. The ALK inhibitor ceritinib overcomes crizotinib resistance in non-small cell lung cancer. Cancer Discov 2014;4:662-73. [PubMed]
- Kogita A, Togashi Y, Hayashi H, et al. Hypoxia induces resistance to ALK inhibitors in the H3122 non-small cell lung cancer cell line with an ALK rearrangement via epithelial-mesenchymal transition. Int J Oncol 2014;45:1430-6. [PubMed]
- Kinoshita K, Asoh K, Furuichi N, et al. Design and synthesis of a highly selective, orally active and potent anaplastic lymphoma kinase inhibitor (CH5424802). Bioorg Med Chem 2012;20:1271-80. [PubMed]
- Sakamoto H, Tsukaguchi T, Hiroshima S, et al. CH5424802, a selective ALK inhibitor capable of blocking the resistant gatekeeper mutant. Cancer Cell 2011;19:679-90. [PubMed]
- Rivera VM, Anjum R, Wang F, et al. Efficacy and pharmacodynamic analysis of AP26113, a potent and selective orally active inhibitor of Anaplastic Lymphoma Kinase (ALK). Cancer Res 2010;70:abstr 3623.
- Zhang S, Wanf F, Keats J, et al. AP26113, a potent ALK inhibitor, overcomes mutations in EML4-ALK that confer resistance to PF-02341066 (PF1066). Cancer Res 2010;70:abstr LB-298.
- Shakespeare WC, Fantin VR, Wang F, et al. Discovery of potent and selective orally active inhibitors of anaplastic lymphoma kinase (ALK). AACR 2009:abstr 3738.
- Johnson TW, Richardson PF, Bailey S, et al. Discovery of (10R)-7-amino-12-fluoro-2,10,16-trimethyl-15-oxo-10,15,16,17-tetrahydro-2H-8,4-(metheno)pyrazolo(4,3-h)(2,5,11)-benzoxadiazacyclotetradecine-3-carbonitrile (PF-06463922), a macrocyclic inhibitor of anaplastic lymphoma kinase (ALK) and c-ros oncogene 1 (ROS1) with preclinical brain exposure and broad-spectrum potency against ALK-resistant mutations. J Med Chem 2014;57:4720-44. [PubMed]
- Zou HY, Li Q, Engstrom LD, et al. PF-06463922 is a potent and selective next-generation ROS1/ALK inhibitor capable of blocking crizotinib-resistant ROS1 mutations. Proc Natl Acad Sci U S A 2015;112:3493-8. [PubMed]
- Ou SH. Crizotinib: a novel and first-in-class multitargeted tyrosine kinase inhibitor for the treatment of anaplastic lymphoma kinase rearranged non-small cell lung cancer and beyond. Drug Des Devel Ther 2011;5:471-85. [PubMed]
- Ou SH, Jänne PA, Bartlett CH, et al. Clinical benefit of continuing ALK inhibition with crizotinib beyond initial disease progression in patients with advanced ALK-positive NSCLC. Ann Oncol 2014;25:415-22. [PubMed]
- Weickhardt AJ, Scheier B, Burke JM, et al. Local ablative therapy of oligoprogressive disease prolongs disease control by tyrosine kinase inhibitors in oncogene-addicted non-small-cell lung cancer. J Thorac Oncol 2012;7:1807-14. [PubMed]
- Otterson GA, Riely GJ, Shaw AT, et al. Clinical characteristics of ALK+ NSCLC patients (pts) treated with crizotinib beyond disease progression (PD): Potential implications for management. Proc Am Soc Clin Oncol 2012;30:abstr 7600.
- Ramalingam SS, Khuri FR. Second-generation ALK inhibitors: filling the non “MET” gap. Cancer Discov 2014;4:634-6. [PubMed]
- Li S, Qi X, Huang Y, et al. Ceritinib (LDK378): a potent alternative to crizotinib for ALK-rearranged non-small-cell lung cancer. Clin Lung Cancer 2015;16:86-91. [PubMed]
- Marsilje TH, Pei W, Chen B, et al. Synthesis, structure-activity relationships, and in vivo efficacy of the novel potent and selective anaplastic lymphoma kinase (ALK) inhibitor 5-chloro-N2-(2-isopropoxy-5-methyl-4-(piperidin-4-yl)phenyl)-N4-(2-(isopropylsulfonyl)phenyl)pyrimidine-2,4-diamine (LDK378) currently in phase 1 and phase 2 clinical trials. J Med Chem 2013;56:5675-90. [PubMed]
- Kim DW, Mehra R, Tan DS, et al. Ceritinib in advanced anaplastic lymphoma kinase (ALK)-rearranged (ALK+) non-small cell lung cancer (NSCLC): Results of the ASCEND-1 trial. J Clin Oncol 2014;32:abstr 8003.
- Shaw AT, Mehra R, Tan DS, et al. Evaluation of Ceritinib-treated patients with anaplastic lymphoma kinase rearranged (ALK+) non-small cell lung cancer (NSCLC) and brain metastases in the ASCEND-1 study. Ann Oncol 2014;25:abstr 1293P.
- Gadgeel SM, Gandhi L, Riely GJ, et al. Safety and activity of alectinib against systemic disease and brain metastases in patients with crizotinib-resistant ALK-rearranged non-small-cell lung cancer (AF-002JG): results from the dose-finding portion of a phase 1/2 study. Lancet Oncol 2014;15:1119-28. [PubMed]
- Kodama T, Hasegawa M, Takanashi K, et al. Antitumor activity of the selective ALK inhibitor alectinib in models of intracranial metastases. Cancer Chemother Pharmacol 2014;74:1023-8. [PubMed]
- Lin NU, Lee EQ, Aoyama H, et al. Challenges relating to solid tumour brain metastases in clinical trials, part 1: patient population, response, and progression. A report from the RANO group. Lancet Oncol 2013;14:e396-406. [PubMed]
- Lin NU, Wefel JS, Lee EQ, et al. Challenges relating to solid tumour brain metastases in clinical trials, part 2: neurocognitive, neurological, and quality-of-life outcomes. A report from the RANO group. Lancet Oncol 2013;14:e407-16. [PubMed]
- Ou SH, Klempner SJ, Azada MC, et al. Radiation necrosis presenting as pseudoprogression (PsP) during alectinib treatment of previously radiated brain metastases in ALK-positive NSCLC: Implications for disease assessment and management. Lung Cancer 2015;88:355-9. [PubMed]
- Gettinger S, Bazhenova L, Salgia R, et al. Updated efficacy and safety of the ALK inhibitor AP26113 in patients (pts) with advanced malignancies, including ALK+ non-small cell lung cancer (NSCLC). J Clin Oncol 2014;32:abstr 8047.
- He H, Lyons KA, Shen X, et al. Utility of unbound plasma drug levels and P-glycoprotein transport data in prediction of central nervous system exposure. Xenobiotica 2009;39:687-93. [PubMed]
- Kodaira H, Kusuhara H, Fujita T, et al. Quantitative evaluation of the impact of active efflux by p-glycoprotein and breast cancer resistance protein at the blood-brain barrier on the predictability of the unbound concentrations of drugs in the brain using cerebrospinal fluid concentration as a surrogate. J Pharmacol Exp Ther 2011;339:935-44. [PubMed]
- Nguyen KS, Neal JW, Wakelee H. Review of the current targeted therapies for non-small-cell lung cancer. World J Clin Oncol 2014;5:576-87. [PubMed]
- Brandsma D, Stalpers L, Taal W, et al. Clinical features, mechanisms, and management of pseudoprogression in malignant gliomas. Lancet Oncol 2008;9:453-61. [PubMed]
- Gainor JF, Sherman CA, Willoughby K, et al. Alectinib salvages CNS relapses in ALK-positive lung cancer patients previously treated with crizotinib and ceritinib. J Thorac Oncol 2015;10:232-6. [PubMed]