Stereotactic ablative body radiotherapy (SABR): an alternative to surgery in stage I-II non-small-cell cancer of the lung?
Introduction
In spite of the remarkable progress in the biological understanding, the pathological and clinical diagnosis and in the various treatments of non-small-cell lung cancer (NSCLC), its overall prognosis remains disappointing, even in early stages. For decades, surgery was considered to be the only standard therapy in early disease, however the 5-year survival rates after a surgical resection ranged 60% to 80% in stage I and only 40% to 50% in stage II (1). Surgery, like other curative treatments for NSCLC, including stereotactic ablative body radiotherapy (SABR), (see below) may be followed by important complications, and can even lead to a decline of quality of life (2), especially in elderly patients (3). Old age and the presence of multiple co-morbidities were, and still are, responsible for the undertreatment of early stage NSCLC in a significant proportion of patients. For example in the Netherlands, up to the late 1990’s, 32% of patients aged 75 years and older could not receive any curative local treatment for stage I NSCLC (4), but that was in an era before new treatments such as video-assisted thoracoscopic surgery (VATS), radiofrequency ablation, cryosurgery and SABR, were commonly available. However since the past decade, SABR has been more and more used in a large number of patients in a growing number of institutions. It is generally accepted that this technique represents now an alternative to surgery, under well-defined conditions, and can be administered to elderly patients and to patients with multiple co-morbidities, as reported by recent reviews (5-9). The present review is primarily intended for interested chest physicians, thoracic surgeons, medical oncologists and radiation oncologists not yet experienced in SABR, and who wish to become more familiar with this technique. In this article, the basic principles of SABR, its practical aspects, the definition of dose, and the results including tumor control and toxicity will be reviewed, and an attempt will be made to compare the results of SABR with those of surgery.
Stereotactic ablative body radiotherapy (SABR)
Rationale and indications
Long before the advent of SABR (also called SBRT for stereotactic body radiotherapy), conventional radiotherapy (RT) was sometimes given to patients with early stage NSCLC who were not candidates for surgery for medical reasons or who refused surgery (10). However, conventional RT techniques had their limitations. Sixty Gy, in conventional daily fractionation of 2 Gy, represents a biologically equivalent dose (BED10) of 72, which is clearly insufficient to control a NSCLC in most situations (see below). So even if some inoperable patients could be cured with conventional RT, the overall results were rather poor, with at least 40% local failures and 5-year overall survival (OS) rates of only 15-20% (10,11). Compared to conventionally fractionated RT, which for NSCLC typically delivers a dose of about 60 Gy in daily fractions of 1.8 to 2 Gy in 6 weeks, SABR represents a completely different philosophy, which delivers very high doses of highly conformal RT to relatively small volumes in a few days. This technique derives from the principles applied in intracranial stereotactic radiosurgery (SRS), which has been administered for decades for intracranial neoplasms (12), and thus uses rather similar technical tools and rather similar radiobiological principles. Thus SABR delivers very large doses, referred to by Timmerman et al., as “ablative” doses (13) of RT in a few high-dose fractionation schedules, typically in 3 to 8 fractions (see below). Compared to conventional RT, this implies major radiobiological re-considerations, referred to by Timmerman et al. in another paper as the “hypofractionated revolution” (14). Thus it is critical for the newcomers in the field to have a good understanding of these radiobiological principles, otherwise major problems and complications will be likely to be met. Besides this, in order to apply SABR, one needs to have at hand the most sophisticated technological tools, high competence in physics, imaging, RT planning and RT delivery, to administer safely this high, compact dose to the target. Each treatment should insure a steep gradient of dose for a maximum avoidance of normal sensitive structures, while hitting the target with the highest precision.
Target definition and treatment planning
After having confirmed the indication for SABR, the first technical step for planning SABR includes a careful identification of the target with the best currently available imaging tools, including a high-quality CT with appropriate windowing. Then, a planning 4 D CT is obtained, to define not only the gross tumor volume (GTV) and the clinical target volume (CTV), but also and most importantly the internal target volume (ITV, Figure 1), which represents the space occupied by the tumor during the whole respiratory cycle. Depending on the tumor volume and motion the radiation can be applied either (I) to the whole ITV, particularly in case of limited tumor volume and tumor motion, or (II) by using a “gating” technique, in which the irradiation is applied only during part of the respiratory cycle, or (III) using a “tracking” technology, in which the tumor is “followed” by the beam during the respiratory cycle. During the planning procedure, (like for any high-precision RT), it is essential to determine the best treatment plan by optimal dose-volume histograms (DVH). Normal tissue constraints values, which are defined specifically for large fractions, have to be used, and can be found in related papers (13). Although many different technical approaches have been used, to ensure the best distribution of dose high-technology linacs with intensity modulation RT (IMRT), or volumetric arc therapy (V-MAT) and image-guided RT (IGRT) technology, or other tools like the Tomotherapy or the Cyberknife systems, or even the proton-beam technology, have to be used. However at present, there are no data demonstrating the superiority of any of these treatment techniques over any other ones. Stable and reproducible positioning is essential, using either various frame systems to better immobilize the patients, or frameless systems using markers and image-guided systems.
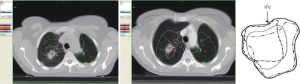
Definition of dose in SABR
SABR implies a large total dose in a few fractions. It should be remembered that what is called a radiobiological dose has not at all the same meaning as a physical dose. Due to the decreased or absent DNA repair when large individual doses per fraction are given to any tissue (tumor or normal tissue), a dose such as 20 Gy given in one fraction is much more efficient than 20 Gy given in 10 fraction (Figure 2). Similarly, 60 Gy in 3 fractions is much more “tumoricidal” (and hugely more toxic!) than 60 Gy given in 30 fractions. Thus, to establish RT protocols with biologically equivalent doses while using different fractionation schedules, various calculation formulae can be used, like the BED equation, where (3,10):
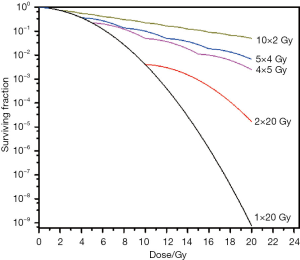
Some examples of SABR schedules and one of a conventional schedule of 60 Gy in 30 fractions, and their corresponding BED10 values are displayed on Table 1. One can see that any dose used for SABR is largely superior to that of the conventional RT scheme. The differences are even greater when taking an α/β value of 3 (BED3) for normal tissues. Specific normal tissue tolerance-dose constraints for 1-5 fractions schedules have to be used and recommendations can be found in the literature (13). This explains why, choosing a protocol for tumors in the vicinity of sensitive normal structures, most investigators today will carefully adapt the total dose and the dose per fraction to decrease the risk of major tissue complications (see below).
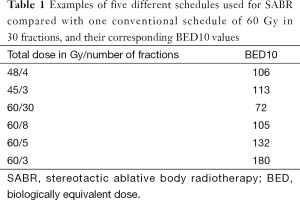
Full table
Results of SABR
Local control and survival
Following SABR for stage I and II NSCLC, several endpoints should be considered to evaluate its success or failure. Local control should ideally be the most important endpoint in SABR, as it should reflect directly the efficacy of the technique, especially when compared to surgery. However the interpretation of the radiographic response is often difficult. A complete disappearance of the tumor is observed only in a minority of patients, and even in case of permanent local control one can still identify some abnormalities, even months later (Figure 3). In a review, 60% to 100% of patients were expected to have radiographic changes after SABR (15).The changes observed on CT could be scored into five categories: (I) diffuse consolidation, (II) patchy consolidation and ground-glass opacities (GGO), (III) diffuse GGO, (IV) patchy GGO, and (V) no evidence of increased density (15). All these can mean permanent local control. FDG-PET may be helpful but inflammatory response may persist more than 12 months (15,16). Interestingly, late radiological changes may differ depending on the SABR technique that was used. Arc-SABR trended towards more pronounced radiological changes, with a different pattern, compared to changes seen after fixed-beams SABR (16). Overall, after SABR, permanent local control of the tumor is observed in 81% to 97% of treated patients (Table 2) (17-32). The next other major endpoint is survival: in the same series, survival at 3 years was reported to be between 52% and 64% (Table 2) (17-32). Examples of some typical series show fairly consistent results. Onishi et al. have treated 245 patients in 13 Japanese institutions, with a median BED dose of 108 Gy (57-180 Gy) (17). Local progression occurred in 14.5%, and the 3-year-survival was 56%, with a cause-specific survival of 78%, indicating that a significant proportion of deaths were not cancer-related but were due to other co-morbidities (17). Lagerwaard et al. from VUMC, Amsterdam, have reported on 206 patients treated with 3 schedules of 3×20 Gy, 5×12 Gy and 8×7.5 Gy, depending on T stage and proximity of sensitive structures (19) (see also below). Median survival was 34 months, local failures were observed only in 3% and regional failures in 9% (19). Bradley et al. prospectively registered and analyzed 91 patients from Washington University School of Medicine (WUSM) in St Louis, with 3×18 Gy for peripheral tumors and 5×9 Gy for tumors in close vicinity of critical structures (22). Most patients had either a poor performance status or poor lung functions. Two-year local control was achieved in 86% of patients (22). Crabtree et al. have reviewed 151 SABR patients, whom they compared to 458 surgical patients (31) (see below). BED10 varied between 85.5 and 151.2. For the SABR group, 3-year local control was 89% and OS 52% (31).
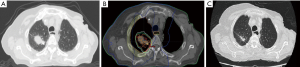
Optimal dose of SBRT
Since the first reports on SABR, many different schedules of dose have been explored, in order to find the best therapeutic ratio, taking into account the best probability of tumor control with a minimal risk of causing major complications.
One initial dose-escalation phase I study was initiated at Indiana University to assess toxicity and local control rates (33). Forty-seven patients were treated with SABR escalating from a starting dose of 24 Gy in three fractions up to 72 Gy in three fractions. Patients were stratified by T stage and tumor size. The maximum tolerated dose (MTD) was 66 Gy in 3 fractions for tumors larger than 5 cm and was not reached for T1 tumors at 60 Gy in 3 fractions or tumors less than 5 cm at 66 Gy in 3 fractions (33). Chi et al. have extensively reviewed and linked radiobiological modeling and clinical outcome from 9 series of patients (34). Their estimates indicate a clear dose-response relationship: for example with BED10 values of 72, 84, 106 (see corresponding doses and fractions in Gray on Table 1), the progression-free survival at 30 months (PFS30) is only 15%, 24%, and 34%, respectively (34). With higher BED10, the PFS30 increases markedly: BED10 values of 113 and 125 correspond to a PFS30 of 95% and 99%, respectively and then of course a plateau is reached (34). Beyond these BED10 values, one may question the necessity to administer higher RT doses, as the toxicity of normal tissues increases even more (see below). Kestin et al. have reviewed 505 T1 and T2 NSCLC in 483 patients treated by SABR in 5 institutions in the USA, Germany, The Netherlands and Canada (32). All were treated with on-line image-guidance RT (32). Five different schedules, with a median prescription BED10 of 132 Gy, were used. A clear dose-response relationship for local control was demonstrated, with an optimal BED10 > to 125 Gy (32). Zhang et al. have performed a meta-analysis on 2,587 patients from 34 observational studies (35). BED was divided into 4 dose groups: low (<83.2), medium (83.2-106), medium to high [106-146] and high (>146). As expected, overall 2- and 3-year survivals were higher in the medium dose and medium to high dose groups compared to the low dose group. However, and interestingly, the former two groups were also superior to the high dose group for OS (35). Not all studies however suggest the concept of the dose-response hypothesis in SABR. Van Baardwijk et al. in their systematic overview of 15 studies on SBRT (=SABR) found no correlation between the freedom from local progression and the EQD2,T, or equivalent dose in 2 Gy fractions (36). In any case, it seems clear from most studies that a BED10 beyond a certain value (around 120-130 or so) may not only be unnecessary, as one could estimate from Chi et al.’s data (34), but may be even detrimental, due to an increased toxicity.
Patterns of failures
As seen in Table 2, the rate of local control in most series is above 85%. Provided that a careful initial work up is made, to exclude nodal disease, the regional failure rate should also be low (see below). Thus the largest proportion of failures are represented by distant metastases (37). Bradley et al. in their series of 91 patients from WUSM, have reported that the majority of the failures were distant, with 19 of them being distant metastases, with or without some local (7 cases) and/or regional component (22). Distant failures negatively impacted the OS. In addition, 15 patients developed a second primary lung cancer (22). The largest study to date regarding the pattern of failure was published by Senthi et al. from VUMC Amsterdam (38). They have assessed 676 patients treated by SABR between 2003 and 2011 (38). Eighteen percent had a disease recurrence, with an actuarial 2-year rate of local, regional (nodal) and distant recurrence rate of 4.9%, 7.8%, and 14.7%, respectively, and with corresponding rates at 5 years of 10.5%, 12.7% and 19.9%. New pulmonary lesions, or second primary tumors, developed in 6% of all patients (38).
Toxicity
With SABR, like with high-dose, conventional RT, there is a potential risk of major complications, such as radiation pneumonitis, oesophagitis and brachial plexopathy. In addition, new severe and sometimes fatal complications have been reported with SABR, including tracheo-oesophagial fistulae, brocho-pulmonary fistulae, cardiotoxicity and chest wall necrosis (39). The latter complication, along with rib fractures, may be particularly severe and painful. Timmerman et al. in a seminal paper have reported their experience on 70 patients treated for T1 and T2 (<7 cm) with 60-66 Gy in 3 fractions (BED10: 180-211) (40). Significant grade 3 to 5 toxicity occurred in 14 patients, with 6 toxic deaths. Patients with peripheral lung tumors had a 2-year freedom from severe toxicity of 83% versus only 54% for centrally located tumors (40). They have defined an area referred to as the zone of the proximal bronchial tree, in which very high BED doses should be prohibited (40). In this regard, Lagerwaard et al. have designed a “risk-adapted” protocol taking into account tumor size and location (19). T1 peripheral tumors received 60 Gy in 3 fractions (BED10: 180), T1 with broad contact with the thoracic wall or T2 tumors received 60 Gy in 5 fractions (BED10: 132) and tumors adjacent to the heart, hilus and mediastinum received 60 Gy in 8 fractions (BED10: 105) (19). With this protocol, which reduces considerably the biological dose given to the normal “central” organs, severe toxicity was observed only in 3% of all patients, without compromizing the overall local control, which was excellent with only 3% crude local failure rate (19). Figure 4 shows two examples of central or peripheral lesions treated at our institution (CLS), with doses adapted to their location. Senthi et al. have reviewed the toxicity of SABR based on 20 publications including 563 central lung tumors (5). They confirm that with the above-mentionned precautions, tumor location did not impact OS or toxicity (5).
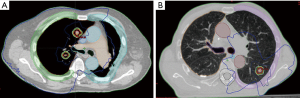
Medically operable or inoperable patients
At its beginning, SABR was intended for patients who were deemed inoperable because of age or multiple co-morbidities and inacceptable surgical risks. However patients who refused surgery were also candidates for this new procedure. Onishi et al. in their series of 245 patients, reported that 158 were considered to be inoperable and 87 to be operable (17). There was a highly significant difference in survival (P<0.01) between the two categories in favor of the operable patients, the latter having a 3-year-survival of 88%. In the group of inoperable patients, the rate of intercurrent deaths (deaths from other causes) was 19.1% versus only 3.4% for operable patients (17). This explains at least in part the large difference in OS between the two groups. Lagerwaard et al. found that in their prospective database of SABR, 177 patients (25% of their cases) were deemed potentially operable, using strict criteria (41). In this group of patients, the 1- and 3-year survival rates were 94.7% and 84.7%, respectively, and the local control rates 98% and 93%, respectively (41). Interestingly, in certain circumstances, a biopsy prior to SABR can be omitted. Verstegen et al. have analyzed a fairly large cohort of patients, with (209 patients) or without (382) a biopsy prior to SABR (42). Local control and OS were exactly the same in the two groups of patients (42). To not biopsy certain patients is based on the fact that only 1-4% of FDG-PET positive lesions undergoing surgery are benign (42), and thus with a careful imaging assessment, the risk without a biopsy of treating a benign lesion instead of a cancerous lesion is low.
At this point the question is raised whether SBRT could be an alternative to surgery, even in the most favorable category of patients with early NSCLC.
Surgery versus SABR for stage I and II NSCLC
Evidently to compare the efficiency of SABR vis-à-vis surgery, randomized controlled trials are needed. Unfortunately, three major initiatives in the USA and Europe have failed recently, due to poor accrual (43). One has thus to rely on matched-paired analyses, in which carefully matched patients in each comparative groups are analyzed. Verstegen et al. have matched SABR patients and patients treated by VATS from six hospitals in The Netherlands (44). The cohort consisted of 64 SABR and 64 VATS. Post SABR local control rates were superior at 1 and 3 years (96.8% and 93%, versus 86.9% and 82.6% respectively, P=0.04), but distant recurrence and OS were not different (44). Crabtree et al. from WUSM compared 462 surgical patients to 76 receiving SABR, and found that surgical patients were healthier and had a better tumor control compared with those receiving SABR (31). However when they did a propensity analysis, they found that local recurrence and disease-specific survival were similar in the two groups (31). A meta-analysis of six studies containing 864 matched-paired patients was performed by Zhang et al. (45). Pooled data at 1 and 3 years indicate a better long-term OS with surgery. However the rate of cancer deaths was the same in the two groups of patients, which strongly suggests that in spite of the matching of patients, those undergoing SABR may have been less healthy than the surgical patients. This was indirectly demonstrated by the fact that there was no significant difference in cause-specific survival, disease-free survival or local control between the SABR and the surgical patients (45). Solda et al. have reviewed 45 reports containing 3,771 patients treated with SABR and compared them to 2,038 surgical patients (46). They found that the 2-year survival was 70% after SABR versus 68% after surgery (46). As regards performance status (PS) and comorbidity as independent prognostic factors which may be used for treatment decisions, Louie et al. have constructed univariate and multivariate models to establish recursive partitioning analyses (RPA) classes and a nomogram (47). RPA identified two risk classes based on tumor diameter, age, PS and co-morbidity index, but performed poorly in surgical patients, whereas the nomogram retained a strong performance for surgery and SABR (47).
Finally, the enthusiasm generated by all the “positive” data on SBRT should be tempered by a more critical assessment of this new technique. Brada et al. in a recent editorial (48) have expressed a series of reservations vis-à-vis the “overconfidence” and “self-congratulation” around SABR (48). For example they remind that other newer approaches, like new surgical techniques (VATS), radiofrequency or thermal ablation may provide equivalent tumor control as SABR (48). They also underscore that the local control after SABR may be overestimated, given for example the difficulty to assess this endpoint with the current imaging means (see above), and that long-term toxicity may be underestimated as well. They question whether SABR impacts on the natural history of co-morbid situations. They also emphasize that more studies are needed to better define a series of unsolved or insufficiently solved issues, for example on respiratory and cardiac co-morbidities, on the optimal dose and fractionation and on long-term toxicities (48).
Conclusions
SABR is now a well-established technique for the treatment of early stage NSCLC, which requires a high quality of the teams and of the techniques to be used. Besides this high technology, a good understanding of the radiobiological principles is of paramount importance, in order to decrease the risk of severe complications. A dose-adapted scheme has to be used in each institution practicing SABR. Probably a BED10 dose over 120-130 is unnecessary for peripheral lesions, and should be even lower for centrally located tumors, probably not beyond 110. Results show at this point a very good local control, and an acceptable toxicity, provided a proper overall evaluation is made and the appropriate biological effective dose is selected. SABR is now a first choice for medically inoperable patients. For operable patients, at the present time, surgery remains the standard, but SABR can be a good second option for patients who refuse surgery. At the present time though, more studies are needed because a number of problems have not been entirely solved and longer follow-ups are required.
Acknowledgements
The author would like to thank Mrs Stefania Bressan who has provided figures 1,3 and 4 in this article.
Footnote
Conflicts of Interest: The author has no conflicts of interest to declare.
References
- Scott WJ, Howington J, Feigenberg S, et al. Treatment of non-small cell lung cancer stage I and stage II: ACCP evidence-based clinical practice guidelines (2nd edition). Chest 2007;132:234S-242S.
- Schulte T, Schniewind B, Dohrmann P, et al. The extent of lung parenchyma resection significantly impacts long-term quality of life in patients with non-small cell lung cancer. Chest 2009;135:322-9. [PubMed]
- Rueth NM, Parsons HM, Habermann EB, et al. Surgical treatment of lung cancer: predicting postoperative morbidity in the elderly population. J Thorac Cardiovasc Surg 2012;143:1314-23. [PubMed]
- Haasbeek CJ, Palma D, Visser O, et al. Early-stage lung cancer in elderly patients: a population-based study of changes in treatment patterns and survival in the Netherlands. Ann Oncol 2012;23:2743-7. [PubMed]
- Senthi S, Haasbeek CJ, Slotman BJ, et al. Outcomes of stereotactic ablative radiotherapy for central lung tumours: a systematic review. Radiother Oncol 2013;106:276-82. [PubMed]
- Palma DA, Senan S. Improving outcomes for high-risk patients with early-stage non-small-cell lung cancer: insights from population-based data and the role of stereotactic ablative radiotherapy. Clin Lung Cancer 2013;14:1-5. [PubMed]
- Senan S, Paul MA, Lagerwaard FJ. Treatment of early-stage lung cancer detected by screening: surgery or stereotactic ablative radiotherapy? Lancet Oncol 2013;14:e270-4. [PubMed]
- Boujelbene N, Elloumi F, Kamel ME, et al. Stereotactic body radiation therapy in stage I inoperable lung cancer: from palliative to curative options. Swiss Med Wkly 2013;143:w13780. [PubMed]
- Louie AV, Palma DA, Dahele M, et al. Management of early-stage non-small cell lung cancer using stereotactic ablative radiotherapy: controversies, insights, and changing horizons. Radiother Oncol 2015;114:138-47. [PubMed]
- Dosoretz DE, Katin MJ, Blitzer PH, et al. Radiation therapy in the management of medically inoperable carcinoma of the lung: results and implications for future treatment strategies. Int J Radiat Oncol Biol Phys 1992;24:3-9. [PubMed]
- Wisnivesky JP, Bonomi M, Henschke C, et al. Radiation therapy for the treatment of unresected stage I-II non-small cell lung cancer. Chest 2005;128:1461-7. [PubMed]
- Kushnirsky M, Patil V, Schulder M. The history of stereotactic radiosurgery. In: Chin LS, Ragine WR, editors. Principles and practice of stereotactic radiosurgery. Springer, 2015:3-10.
- Timmerman RD. An overview of hypofractionation and introduction to this issue of seminars in radiation oncology. Semin Radiat Oncol 2008;18:215-22. [PubMed]
- Timmerman RD, Herman J, Cho LC. Emergence of stereotactic body radiation therapy and its impact on current and future clinical practice. J Clin Oncol 2014;32:2847-54. [PubMed]
- Bradley J. Radiographic response and clinical toxicity following SBRT for stage I lung cancer. J Thorac Oncol 2007;2:S118-24. [PubMed]
- Senthi S, Dahele M, van de Ven PM, et al. Late radiologic changes after stereotactic ablative radiotherapy for early stage lung cancer: a comparison of fixed-beam versus arc delivery techniques. Radiother Oncol 2013;109:77-81. [PubMed]
- Onishi H, Araki T, Shirato H, et al. Stereotactic hypofractionated high-dose irradiation for stage I nonsmall cell lung carcinoma: clinical outcomes in 245 subjects in a Japanese multiinstitutional study. Cancer 2004;101:1623-31. [PubMed]
- Xia T, Li H, Sun Q, et al. Promising clinical outcome of stereotactic body radiation therapy for patients with inoperable Stage I/II non-small-cell lung cancer. Int J Radiat Oncol Biol Phys 2006;66:117-25. [PubMed]
- Lagerwaard FJ, Haasbeek CJ, Smit EF, et al. Outcomes of risk-adapted fractionated stereotactic radiotherapy for stage I non-small-cell lung cancer. Int J Radiat Oncol Biol Phys 2008;70:685-92. [PubMed]
- Chen Y, Guo W, Lu Y, et al. Dose-individualized stereotactic body radiotherapy for T1-3N0 non-small cell lung cancer: long-term results and efficacy of adjuvant chemotherapy. Radiother Oncol 2008;88:351-8. [PubMed]
- Baumann P, Nyman J, Hoyer M, et al. Outcome in a prospective phase II trial of medically inoperable stage I non-small-cell lung cancer patients treated with stereotactic body radiotherapy. J Clin Oncol 2009;27:3290-6. [PubMed]
- Bradley JD, El Naqa I, Drzymala RE, et al. Stereotactic body radiation therapy for early-stage non-small-cell lung cancer: the pattern of failure is distant. Int J Radiat Oncol Biol Phys 2010;77:1146-50. [PubMed]
- Timmerman R, Paulus R, Galvin J, et al. Stereotactic body radiation therapy for inoperable early stage lung cancer. JAMA 2010;303:1070-6. [PubMed]
- Ricardi U, Filippi AR, Guarneri A, et al. Stereotactic body radiation therapy for early stage non-small cell lung cancer: results of a prospective trial. Lung Cancer 2010;68:72-7. [PubMed]
- Matsuo Y, Shibuya K, Nagata Y, et al. Prognostic factors in stereotactic body radiotherapy for non-small-cell lung cancer. Int J Radiat Oncol Biol Phys 2011;79:1104-11. [PubMed]
- Widder J, Postmus D, Ubbels JF, et al. Survival and quality of life after stereotactic or 3D-conformal radiotherapy for inoperable early-stage lung cancer. Int J Radiat Oncol Biol Phys 2011;81:e291-7. [PubMed]
- Takeda A, Kunieda E, Sanuki N, et al. Stereotactic body radiotherapy (SBRT) for solitary pulmonary nodules clinically diagnosed as lung cancer with no pathological confirmation: comparison with non-small-cell lung cancer. Lung Cancer 2012;77:77-82. [PubMed]
- Shibamoto Y, Hashizume C, Baba F, et al. Stereotactic body radiotherapy using a radiobiology-based regimen for stage I nonsmall cell lung cancer: a multicenter study. Cancer 2012;118:2078-84. [PubMed]
- Taremi M, Hope A, Dahele M, et al. Stereotactic body radiotherapy for medically inoperable lung cancer: prospective, single-center study of 108 consecutive patients. Int J Radiat Oncol Biol Phys 2012;82:967-73. [PubMed]
- Hamamoto Y, Kataoka M, Yamashita M, et al. Factors affecting the local control of stereotactic body radiotherapy for lung tumors including primary lung cancer and metastatic lung tumors. Jpn J Radiol 2012;30:430-4. [PubMed]
- Crabtree TD, Puri V, Robinson C, et al. Analysis of first recurrence and survival in patients with stage I non-small cell lung cancer treated with surgical resection or stereotactic radiation therapy. J Thorac Cardiovasc Surg 2014;147:1183-1191; discussion 1191-2. [PubMed]
- Kestin L, Grills I, Guckenberger M, et al. Dose-response relationship with clinical outcome for lung stereotactic body radiotherapy (SBRT) delivered via online image guidance. Radiother Oncol 2014;110:499-504. [PubMed]
- Timmerman R, Papiez L, McGarry R, et al. Extracranial stereotactic radioablation: results of a phase I study in medically inoperable stage I non-small cell lung cancer. Chest 2003;124:1946-55. [PubMed]
- Chi A, Tom55.a phase I study in medically inoperable stage I non-small cell lung cancer. Chest 2003;124:1946-55.;124:1946-55.3;124:1946-55.124:1946-55.6-55.5.l Clin Oncol 2011;34:432-41.
- Zhang J, Yang F, Li B, et al. Which is the optimal biologically effective dose of stereotactic body radiotherapy for Stage I non-small-cell lung cancer? A meta-analysis. Int J Radiat Oncol Biol Phys 2011;81:e305-16. [PubMed]
- van Baardwijk A, Tomjk A. Is high-dose stereotactic body radiotherapy (SBRT) for stage I non-small cell lung cancer (NSCLC) overkill? A systematic review. Radiother Oncol 2012;105:145-9. [PubMed]
- Chi A, Liao Z, Nguyen NP, et al. Systemic review of the patterns of failure following stereotactic body radiation therapy in early-stage non-small-cell lung cancer: clinical implications. Radiother Oncol 2010;94:1-11. [PubMed]
- Senthi S, Lagerwaard FJ, Haasbeek CJ, et al. Patterns of disease recurrence after stereotactic ablative radiotherapy for early stage non-small-cell lung cancer: a retrospective analysis. Lancet Oncol 2012;13:802-9. [PubMed]
- Thibault I, Chiang A, Erler D, et al. Predictors of Chest Wall Toxicity after Lung Stereotactic Ablative Radiotherapy. Clin Oncol (R Coll Radiol) 2015. [Epub ahead of print].
- Timmerman R, McGarry R, Yiannoutsos C, et al. Excessive toxicity when treating central tumors in a phase II study of stereotactic body radiation therapy for medically inoperable early-stage lung cancer. J Clin Oncol 2006;24:4833-9. [PubMed]
- Lagerwaard FJ, Verstegen NE, Haasbeek CJ, et al. Outcomes of stereotactic ablative radiotherapy in patients with potentially operable stage I non-small cell lung cancer. Int J Radiat Oncol Biol Phys 2012;83:348-53. [PubMed]
- Verstegen NE, Lagerwaard FJ, Haasbeek CJ, et al. Outcomes of stereotactic ablative radiotherapy following a clinical diagnosis of stage I NSCLC: comparison with a contemporaneous cohort with pathologically proven disease. Radiother Oncol 2011;101:250-4. [PubMed]
- Moghanaki D, Karas T. Surgery versus SABR for NSCLC. Lancet Oncol 2013;14:e490-1. [PubMed]
- Verstegen NE, Oosterhuis JW, Palma DA, et al. Stage I-II non-small-cell lung cancer treated using either stereotactic ablative radiotherapy (SABR) or lobectomy by video-assisted thoracoscopic surgery (VATS): outcomes of a propensity score-matched analysis. Ann Oncol 2013;24:1543-8. [PubMed]
- Zhang B, Zhu F, Ma X, et al. Matched-pair comparisons of stereotactic body radiotherapy (SBRT) versus surgery for the treatment of early stage non-small cell lung cancer: a systematic review and meta-analysis. Radiother Oncol 2014;112:250-5. [PubMed]
- Solda: F, Lodge M, Ashley S, et al. Stereotactic radiotherapy (SABR) for the treatment of primary non-small cell lung cancer; systematic review and comparison with a surgical cohort. Radiother Oncol 2013;109:1-7.
- Louie AV, Haasbeek CJ, Mokhles S, et al. Predicting Overall Survival After Stereotactic Ablative Radiation Therapy in Early-Stage Lung Cancer: Development and External Validation of the Amsterdam Prognostic Model. Int J Radiat Oncol Biol Phys 2015;93:82-90. [PubMed]
- Brada M, Pope A, Baumann M. SABR in NSCLC--the beginning of the end or the end of the beginning? Radiother Oncol 2015;114:135-7. [PubMed]