Proton therapy for nasopharyngeal carcinoma
Introduction
Nasopharyngeal carcinoma (NPC) is both radio- and chemosensitive. The nasopharynx is an anatomically complex region in which surgical resection can be both technically challenging and result in substantial morbidity. As such, clinical practice guidelines list radiotherapy (RT) as the treatment of choice for early-stage NPC, and RT with chemotherapy as the treatment of choice for locally advanced NPC (1). Despite encouraging clinical outcomes, use of RT for NPC has several challenges. The nasopharynx is adjacent to several critical structures, including the salivary glands, auditory structures, pterygoid and pharyngeal constrictor muscles, optic pathways, brain and brainstem. Harm to these structures may result in significant impairments in the quality of life (QOL) for patients who undergo treatment and can include xerostomia, hearing impairment, trismus, dysphagia, optic neuropathy and temporal lobe necrosis (2).
As techniques for delivery of photon-based RT have advanced from two-dimensional conventional to three-dimensional conformal to intensity-modulated radiotherapy (IMRT), the corresponding improvements in dosimetric properties have allowed increased local control rates with decreased rates of toxicity (3-5). Because IMRT results in decreased dose delivery to the parotids and other major salivary glands, it became the current standard of care (1) after a phase III randomized-controlled trial showed decreased rates of xerostomia with IMRT (3). Even though IMRT allows increased conformality relative to 2D or 3D techniques, because inverse planning objectives are used to spare dose to the parotids, the entrance and exit dose must necessarily be distributed elsewhere. Therefore, the widespread adoption of IMRT for the treatment of NPC has been accompanied by increased toxicity along the beam path (6), which can have substantial effects on QOL (7,8). This has sparked interest in the development and use of techniques that might improve the therapeutic ratio even further for patients with NPC.
Enthusiasm for the use of proton radiotherapy (PRT) was first stimulated by early successes in the treatment of chordomas and chondrosarcomas of the skull base (9) and intraocular melanoma (10). Currently, there is a great deal of interest in the use of PRT for treating of cancers of the head and neck, including NPC (11). Owing to the characteristic sharp dose fall-off of protons, PRT allows delivery of a high therapeutic radiation dose to the tumor with minimal exit dose which improves sparing of normal tissues (11,12). Recent improvements in the dose delivery of protons, including intensity-modulated proton therapy (IMPT) could further reduce toxicity without compromising efficacy (13,14). Dosimetric comparative analyses have explored dosimetric advantages of planning comparisons of IMPT and IMRT and have suggested that lower doses can be delivered to non-target tissues in patients with NPC (15-18). Early findings from Loma Linda University Medical Center and MD Anderson Cancer Center have also described good clinical outcomes of PRT for patients with NPC (18-21).
Evidence of dosimetric advantages
Comparisons of treatment plans have shown that use of IMPT can better spare normal tissues while maintaining effective delivery of the dose to the target (15-18). In one such study, Taheri-Kadkhoda and colleagues found that IMPT plans for NPC were associated with lower mean doses to the larynx/esophagus, oral cavity, and parotid glands relative to IMRT plans (15). Meanwhile, Widesott and others compared plans for helical tomotherapy with those from IMPT and also found that IMPT produced lower mean doses to the bilateral parotid glands, but not to the larynx or esophagus (16).
At MD Anderson, we analyzed the treatment plans of the first nine patients treated with IMPT for NPC at our proton center and generated IMRT comparison plans for analysis (18). Coverage of the primary clinical target volume (CTV1) was similar and excellent for both IMPT and IMRT plans. Minimum and maximum doses to the CTV1 were also similar between IMRT and IMPT plans, although the mean dose to the CTV1 was significantly higher with IMPT. Although the D95 was similar between IMRT and IMPT plans, the D5 was higher with IMPT plans. No significant differences were found between plans in conformality or homogeneity indices.
In terms of planned dose to non-target tissues, we found that IMPT delivered significantly lower mean doses to the whole brain, spinal cord and brainstem as well as other neurologic structures such as the area postrema and subthalamic nucleus, which are thought to play a role in radiation-induced nausea and vomiting (22,23). Mean dose to the cochleae and vestibules of the ear were also significantly lower in IMPT plans, with an absolute reduction of approximately 10 Gray-relative biological effectiveness [Gy(RBE)], which could minimize the risk of long-term sensorineural hearing loss (24). IMPT plans delivered approximately 10 Gy(RBE) less absolute dose to the mandible than did IMRT, which could decrease the risk of osteoradionecrosis of the jaw (25). IMPT plans achieved an absolute mean laryngeal dose reduction of 12 Gy(RBE) relative to IMRT, which indicates IMPT may be useful in decreasing the risk of laryngeal edema (26). Finally, IMPT achieved an absolute mean dose reduction of approximately 20 Gy(RBE) compared with IMRT to the oral cavity. This is likely the area of greatest potential impact on acute and chronic toxicities affecting patient QOL, such as acute mucositis, oral pain, odynophagia and dysphagia, which can be quite debilitating (27).
Based on the available dosimetric evidence, proton therapy, particularly IMPT, seems to be a promising new treatment option for NPC. Because published clinical findings are currently limited, further study is needed to determine if the dosimetric advantages conferred by IMPT indeed translate to reduced toxicity and an improved therapeutic index.
Evidence of clinical benefit
Few full manuscripts have been published reporting clinical outcomes of the use of PRT for patients with NPC. The first published report, from Loma Linda University Medical Center, described outcomes after using PRT to re-irradiate patients with recurrent NPC (19,20). All of the patients in that group had initially treated with photon-based RT as upfront therapy and then were then re-treated to 59.4–70.2 Gy(RBE) by using a 3D conformal PRT technique after developing local recurrence. Rates of overall survival and local-regional progression-free survival were both 50% at 2 years, but these patients had not received concurrent chemotherapy, which is now considered the standard of care. Recurrence and survival rates also varied widely according to the extent of target coverage. Dose-volume histograms were analyzed separately for those with “optimal coverage” (2-year OS 83%) versus “suboptimal coverage” (2-year OS 17%), where “optimal” was conservatively defined as 90% of the target volume receiving 90% of the prescribed dose (19,20).
Investigators from Massachusetts General Hospital (MGH) presented their early experience with using proton therapy for NPC in abstract form in 2004 (28). At 3 years, the local control rate was 92%, the progression-free survival rate 75%, and the overall survival rate 74% for 19 patients with T4 nasopharyngeal cancer, 10 of whom had received induction or concurrent chemotherapy. However, more than 10 years have elapsed since presentation of these findings, and it remains unclear when the full findings from that study will be available. MGH is currently conducting a phase II study evaluating combined proton-photon therapy to 70 Gy(RBE) in 35 daily fractions given with concurrent cisplatin and fluorouracil (NCT00592501). Preliminary results from 23 consecutive patients with stage III–IVB NPC in this trial were presented in abstract form in 2012. A 3D conformal PRT technique was used, with two posterior oblique fields used to spare the parotids with no exit dose into the oral cavity. At 28 months of follow-up, the local control rate was 100%; the 2-year disease-free survival rate was 90%; and the overall survival rate was 100%. Toxic effects included hearing loss (29%), weight loss (38%), and gastrostomy tube placement (48%), but no patient experienced grade ≥3 xerostomia (29).
Available studies describing IMPT for patients with NPC are even more limited. At MD Anderson, we published control and survival outcomes as well as acute and chronic toxicities experienced by the first nine patients treated with IMPT for NPC (18). With a median follow-up of just over 2 years, no patients in our cohort developed local or regional recurrence; however, one patient developed distant metastatic disease and subsequently died. No patients developed any acute or chronic grade 4 or 5 toxicity. Four patients developed acute grade 3 radiation dermatitis and one patient developed acute grade 3 mucositis. Eight patients experienced grade 2 mucositis; however, mucositis was seen only with in the treatment field and no patients developed mucositis in the anterior oral cavity. Xerostomia was the most common chronic toxicity, with six patients experiencing grade 1 xerostomia and two patients with grade 2 xerostomia. Patients lost a median of 6.0% (range, 4.2–14.0%) of their body weight during the course of IMPT. Five patients experienced grade 1 weight loss, and three patients had grade 2 weight loss. Two patients required feeding tube placement during or immediately after IMPT (18). Indeed, few patients required feeding tubes in our patients with NPC treated with IMPT compared with prior series of patients treated with IMRT (30,31). We later undertook a case-matched control study in which ten patients with NPC treated with IMPT were matched in a 2:1 fashion with 20 patients treated with IMRT at our institution during the same treatment era (21). Patients were matched by T-category, N-category, radiation dose, chemotherapy type, WHO classification, sex, and age. By the end of treatment, 20% of patients treated with IMPT required feeding tube insertion compared with 65% of patients treated with IMRT (P=0.020). In agreement with our previously published dosimetric analysis (18), patients receiving IMPT had significantly lower mean doses to the oral cavity, brainstem, whole brain and mandible. We also found higher mean doses to the oral cavity to be associated with higher rates of feeding tube placement (P<0.001). Partitioning analysis showed that no patient required a feeding tube if the mean oral cavity dose was <26 Gy, but all patients with a mean oral cavity dose >41.8 Gy ended up requiring a feeding tube. Treatment type (IMPT vs. IMRT), induction chemotherapy (yes vs. no), mean oral cavity dose, mean brainstem dose, and mean mandible dose were entered into a multivariable model, but only higher mean oral cavity dose remained significantly associated with higher feeding tube rates on multivariable analysis [odds ratio (OR) 1.31; 95% confidence interval (CI), 1.09–1.69 per 1 Gy or Gy(RBE) excess to the oral cavity; P=0.003] (21).
Treatment planning and delivery
Use of IMPT
The technology available for PRT treatment planning, delivery and evaluation varies by institution but in general includes passive scatter or active scanning proton delivery techniques. Active scanning is often called IMPT because the intensity of the proton beam can be varied from spot to spot to deliver the desired dose in the target volume after summing the contributions of all treatment fields. IMPT is different than IMRT in that the energy as well as the intensity can be varied. Planning techniques for IMPT include single-field optimization (SFO) and multiple-field optimization (MFO). SFO involves optimizing the spot-intensities for each beam individually, whereas MFO optimizes the intensities of all beams simultaneously to balance the doses to targets and organs-at-risk (OARs) based on stated objectives. At MD Anderson, the IMPT technique with MFO is used for all patients receiving PRT for NPC.
Patient set-up and immobilization
Treatment planning begins with positioning the patient supine on the simulation table with a head and neck extender board and fabrication of a custom thermoplastic mask and bite block for reproducible immobilization (Figure 1). Next, a non-contrast computed tomography (CT) scan is obtained for treatment planning purposes. An eclipse proton therapy treatment planning system (version 8.9, Varian Medical Systems, Palo Alto, CA, USA) is used for the generation of IMPT plans by expert dosimetrists specially trained in proton treatment planning (Figure 2).
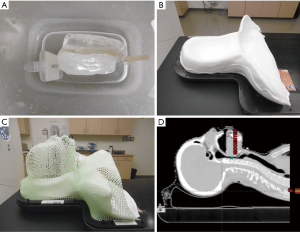
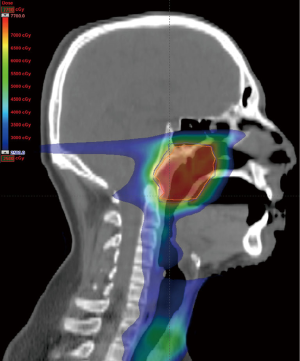
Target volume delineation
Target volumes and OARs are delineated on the treatment-planning CT scans by the treating radiation oncologist. At MD Anderson, CTV1 is defined as gross disease plus a 1-cm margin, CTV2 is defined as the high-risk nodal volume adjacent to gross disease in the neck, and CTV3 is defined as the uninvolved nodes in the neck considered to be at risk for harboring subclinical disease. A volumetric expansion of 3–5 mm is added to each CTV to generate the respective planning target volumes (PTVs). Prescribed doses to PTV1, PTV2 and PTV3 are 70, 63 and 57 Gy(RBE) (RBE =1.0 for photons and 1.1 for protons), respectively, to be given in 33–35 fractions. OARs with specified dose constraints are contoured for treatment planning, including the brainstem, spinal cord, cochleae, salivary glands, oral cavity, and larynx. All contours in all cases are reviewed for quality assurance purposes by a team of head and neck radiation oncology experts before treatment.
Robust planning
Typically, three fields from three different beam directions (combining gantry/couch angles) are used for full-field IMPT plans treating the nasopharynx and bilateral neck. These beam angles are selected using the process of “robust planning”, which consists of the thoughtful selection of beam angles such that the overall robustness of the plan can be maximized. When possible, we avoid passing beams through areas in which the tissues are heterogeneous. We also avoid directing beams in the direction of critical OARs. The three beam angles used for full-field IMPT plans typically include a posterior beam, a left anterior oblique beam and a right anterior oblique beam shifted slightly in the superior-inferior direction (couch kick 15° to 20°).
Robust optimization
MFO is then done by the treatment planning system that simultaneously optimizes the spot intensities from all fields by using an algorithm having the set objective of covering 95% of the PTV with the prescribed dose while minimizing dose to the adjacent OARs. As described above, the PTV is generated by adding a uniform margin around each CTV. This method of optimization is called “PTV-based” optimization and is similar to the way IMRT is currently planned. Our group and others have also developed methods for robust optimization that take into account the “worst-case” dose distributions based on perturbations in the isocenter position and stopping ratio (32,33). In our robust optimization algorithm, the isocenter of the plan is rigidly shifted in the anterior-posterior, superior-inferior, and lateral directions, yielding six influence matrices. This process models the potential effects of set-up uncertainties. Next, the stopping power ratios are modified by −3.5% and 3.5% to generate two additional influence matrices corresponding to maximum and minimum proton ranges, respectively, to model the potential effects of stopping-power uncertainties. The “worst-case” dose distribution is identified as the minimum of the nine doses in each voxel in the CTV and the maximum of the nine doses in each voxel in the OARs (33), and then the objective function is computed for a given iteration by using this “worst-case” dose distribution (32,34,35). We do not yet use this robust optimization process routinely for all patients with NPC being treated with IMPT; however, because treatment planning studies have shown robust optimization to lead to superior OAR-sparing (33), we expect to use this technique increasingly more in the future.
Robust analysis
For NPC patients whose IMPT plans are optimized by using a PTV-based approach, robustness is evaluated with a process called “robust analysis”. Once the optimized IMPT treatment plan has been generated, plans are tested by incorporating the effects of isocenter shifting as well as stopping power variation on target coverage and dose to OARs. Isocenter shifts ±2 mm in the anterior-posterior, superior-inferior and lateral positions are tested to represent setup uncertainties, and stopping power ratios of ±3.5% are tested to represent range uncertainties. Dose-volume histograms are generated for the optimized plan as well as for each perturbation tested during the robust analysis process, thus generating a range of possible doses to each target or OAR. The treating physician can then evaluate whether the target coverage and dose to OARs are within the acceptable range for all scenarios tested.
Plan verification and adaptive planning
Orthogonal 2D kV X-ray images obtained daily are compared with digitally reconstructed radiographs generated by the treatment planning system from simulation CT images with the goal of aligning the patient for image guidance. Patients are seen in clinic weekly by their treating radiation oncologist so that any type of acute toxicity can be documented as well as appropriately monitored and treated.
Anatomic variations due to weight loss and tumor response to radiation can also lead to differences between the actual dose delivered compared with what was originally planned (36). Thus, our patients receive regular counseling with a dietician as well as close monitoring of their weight during treatment. If patients lose more than 10 pounds or 10% of their body weight, we often obtain a verification CT simulation scan and then use deformable registration to overlay the dose from the initial IMPT plan onto the verification CT scan. If CTV coverage degrades to <95%, if the plan maximum point dose exceeds 80 Gy(RBE), or if OAR constraints are exceeded, then off-line adaptive re-planning is done during the fourth week of treatment.
Future directions
The above-mentioned dosimetric and clinical studies have sparked further interest in prospective studies of PRT for NPC. MGH is currently enrolling patients in a non-randomized phase II trial of PRT given with chemotherapy for ≥ T2b or node-positive, non-metastatic NPC (NCT00592501). Treatment consists of 7 weeks of RT, given with cisplatin and fluorouracil once every 3 weeks during RT and then once every 4 weeks after radiation for a total of three cycles. The primary outcome is QOL, and the secondary outcome measures are rate and pattern of locoregional tumor recurrence. This trial began accruing patients in 2006 and is planned to be completed in 2015. The University of Florida is also currently accruing patients to another non-randomized phase II trial evaluating combined photon/proton radiation for non-metastatic NPC (NCT00797290).
Current evidence on the use of PRT for NPC suggests that this type of therapy is not only feasible and safe but may be superior in terms of reducing toxicity while maintaining or improving local tumor control. Continuing advances in the delivery of protons such as IMPT may reduce toxicity and enhance the conformality of target coverage even further. As the number of proton therapy centers opened, under construction or in development increases, we encourage clinicians to consider opening prospective clinical trials so that high-quality evidence can be collected to better use this form of treatment for patients with NPC in the future.
Acknowledgements
None.
Footnote
Conflicts of Interest: The authors have no conflicts of interest to declare.
References
- NCCN Clinical Practice Guidelines in Oncology (NCCN Guidelines®) Head and Neck Cancers. Available online: www.nccn.org/head-and-neck.pdf. Accessed 11/25/2015.
- Zackrisson B, Mercke C, Strander H, et al. A systematic overview of radiation therapy effects in head and neck cancer. Acta Oncol 2003;42:443-61. [Crossref] [PubMed]
- Nutting CM, Morden JP, Harrington KJ, et al. Parotid-sparing intensity modulated versus conventional radiotherapy in head and neck cancer (PARSPORT): a phase 3 multicentre randomised controlled trial. Lancet Oncol 2011;12:127-36. [Crossref] [PubMed]
- Kim JW, Cho JH, Keum KC, et al. IMRT with simultaneous integrated boost and concurrent chemotherapy for nasopharyngeal cancer: plan evaluation and treatment outcome. Jpn J Clin Oncol 2012;42:1152-60. [Crossref] [PubMed]
- Kuang WL, Zhou Q, Shen LF. Outcomes and prognostic factors of conformal radiotherapy versus intensity-modulated radiotherapy for nasopharyngeal carcinoma. Clin Transl Oncol 2012;14:783-90. [Crossref] [PubMed]
- Rosenthal DI, Chambers MS, Fuller CD, et al. Beam path toxicities to non-target structures during intensity-modulated radiation therapy for head and neck cancer. Int J Radiat Oncol Biol Phys 2008;72:747-55. [Crossref] [PubMed]
- Chambers MS, Garden AS, Kies MS, et al. Radiation-induced xerostomia in patients with head and neck cancer: pathogenesis, impact on quality of life, and management. Head Neck 2004;26:796-807. [Crossref] [PubMed]
- Zheng Y, Han F, Xiao W, et al. Analysis of late toxicity in nasopharyngeal carcinoma patients treated with intensity modulated radiation therapy. Radiat Oncol 2015;10:17. [Crossref] [PubMed]
- Catton C, O'Sullivan B, Bell R, et al. Chordoma: long-term follow-up after radical photon irradiation. Radiother Oncol 1996;41:67-72. [Crossref] [PubMed]
- Gragoudas ES, Goitein M, Verhey L, et al. Proton beam irradiation. An alternative to enucleation for intraocular melanomas. Ophthalmology 1980;87:571-81. [Crossref] [PubMed]
- Holliday EB, Frank SJ. Proton radiation therapy for head and neck cancer: a review of the clinical experience to date. Int J Radiat Oncol Biol Phys 2014;89:292-302. [Crossref] [PubMed]
- van de Water TA, Bijl HP, Schilstra C, et al. The potential benefit of radiotherapy with protons in head and neck cancer with respect to normal tissue sparing: a systematic review of literature. Oncologist 2011;16:366-77. [Crossref] [PubMed]
- Frank SJ, Cox JD, Gillin M, et al. Intensity modulated proton therapy for head-and-neck cancer: The first clinical experience. Int J Radiat Oncol Biol Phys 2012;84:S475-6. [Crossref]
- Frank SJ, Cox JD, Gillin M, et al. Multifield optimization intensity modulated proton therapy for head and neck tumors: a translation to practice. Int J Radiat Oncol Biol Phys 2014;89:846-53. [Crossref] [PubMed]
- Taheri-Kadkhoda Z, Björk-Eriksson T, Nill S, et al. Intensity-modulated radiotherapy of nasopharyngeal carcinoma: a comparative treatment planning study of photons and protons. Radiat Oncol 2008;3:4. [Crossref] [PubMed]
- Widesott L, Pierelli A, Fiorino C, et al. Intensity-modulated proton therapy versus helical tomotherapy in nasopharynx cancer: planning comparison and NTCP evaluation. Int J Radiat Oncol Biol Phys 2008;72:589-96. [Crossref] [PubMed]
- Brown AP, Urie MM, Chisin R, et al. Proton therapy for carcinoma of the nasopharynx: a study in comparative treatment planning. Int J Radiat Oncol Biol Phys 1989;16:1607-14. [Crossref] [PubMed]
- Lewis GD, Holliday EB, Kocak-Uzel E, et al. Intensity-modulated proton therapy for nasopharyngeal carcinoma: Decreased radiation dose to normal structures and encouraging clinical outcomes. Head Neck 2015. [Epub ahead of print]. [Crossref] [PubMed]
- Lin R, Slater JD, Yonemoto LT, et al. Nasopharyngeal carcinoma: repeat treatment with conformal proton therapy--dose-volume histogram analysis. Radiology 1999;213:489-94. [Crossref] [PubMed]
- Feehan PE, Castro JR, Phillips TL, et al. Recurrent locally advanced nasopharyngeal carcinoma treated with heavy charged particle irradiation. Int J Radiat Oncol Biol Phys 1992;23:881-4. [Crossref] [PubMed]
- Holliday EB, Garden AS, Rosenthal DI, et al. Proton therapy reduces treatment-related toxicities for patients with nasopharyngeal cancer: A case-match control study of intensity-modulated proton therapy and intensity-modulated photon therapy. Int J Particle Therapy 2015;2:19-28. [Crossref]
- Ciura K, McBurney M, Nguyen B, et al. Effect of brain stem and dorsal vagus complex dosimetry on nausea and vomiting in head and neck intensity-modulated radiation therapy. Med Dosim 2011;36:41-5. [Crossref] [PubMed]
- Kocak-Uzel E, Gunn GB, Colen RR, et al. Beam path toxicity in candidate organs-at-risk: assessment of radiation emetogenesis for patients receiving head and neck intensity modulated radiotherapy. Radiother Oncol 2014;111:281-8. [Crossref] [PubMed]
- Cheraghi S, Nikoofar P, Fadavi P, et al. Short-term cohort study on sensorineural hearing changes in head and neck radiotherapy. Med Oncol 2015;32:200. [Crossref] [PubMed]
- Lee IJ, Koom WS, Lee CG, et al. Risk factors and dose-effect relationship for mandibular osteoradionecrosis in oral and oropharyngeal cancer patients. Int J Radiat Oncol Biol Phys 2009;75:1084-91. [Crossref] [PubMed]
- Debelleix C, Pointreau Y, Lafond C, et al. Normal tissue tolerance to external beam radiation therapy: larynx and pharynx. Cancer Radiother 2010;14:301-6. [Crossref] [PubMed]
- Capuano G, Grosso A, Gentile PC, et al. Influence of weight loss on outcomes in patients with head and neck cancer undergoing concomitant chemoradiotherapy. Head Neck 2008;30:503-8. [Crossref] [PubMed]
- Chan AW, Liebsch LJ, Deschler DG, et al. Proton radiotherapy for T4 nasopharyngeal carcinoma. J Clin Oncol 2004;22:abstr 5574.
- Chan A, Adams JA, Weyman E, et al. A phase II trial of proton radiation therapy with chemotherapy for nasopharyngeal carcinoma. Int J Radiat Oncol Biol Phys 2012;84:S151-2. [Crossref]
- Cheng SS, Terrell JE, Bradford CR, et al. Variables associated with feeding tube placement in head and neck cancer. Arch Otolaryngol Head Neck Surg 2006;132:655-61. [Crossref] [PubMed]
- Mangar S, Slevin N, Mais K, et al. Evaluating predictive factors for determining enteral nutrition in patients receiving radical radiotherapy for head and neck cancer: a retrospective review. Radiother Oncol 2006;78:152-8. [Crossref] [PubMed]
- Lomax AJ, Pedroni E, Rutz H, et al. The clinical potential of intensity modulated proton therapy. Z Med Phys 2004;14:147-52. [Crossref] [PubMed]
- Liu W, Frank SJ, Li X, et al. PTV-based IMPT optimization incorporating planning risk volumes vs robust optimization. Med Phys 2013;40:021709. [Crossref] [PubMed]
- Liu W, Zhang X, Li Y, et al. Robust optimization of intensity modulated proton therapy. Med Phys 2012;39:1079-91. [Crossref] [PubMed]
- Pflugfelder D, Wilkens JJ, Oelfke U. Worst case optimization: a method to account for uncertainties in the optimization of intensity modulated proton therapy. Phys Med Biol 2008;53:1689-700. [Crossref] [PubMed]
- Simone CB 2nd, Ly D, Dan TD, et al. Comparison of intensity-modulated radiotherapy, adaptive radiotherapy, proton radiotherapy, and adaptive proton radiotherapy for treatment of locally advanced head and neck cancer. Radiother Oncol 2011;101:376-82. [Crossref] [PubMed]