Needle-guided ablation of locally advanced pancreatic cancer: cytoreduction or immunomodulation by in vivo vaccination?
Introduction
Pancreatic ductal adenocarcinoma (PDAC) is one of the most aggressive forms of cancer; with a 5-year survival rate of only 8%, it is the fourth leading cause of cancer-related death in Western Europe and the United States (1). Survival has hardly improved over the past 40 years. Resection is the only potentially curative therapy, however only <20% of patients present with a tumour that is eligible for surgical resection (2). Moreover, most patients will develop disease recurrence after curative-intent surgery, resulting in a 5-year survival rate of only 12–27% (3,4) and a median overall survival (OS) of 16.8 months (5). More than 50% of patients present with metastatic disease for which systemic chemotherapy is the only proven survival prolonging treatment (6). Advances have been made for these patients with the introduction of FOLFIRINOX (combination chemotherapy containing 5-fluorouracil, leucovorin (folinic acid), irinotecan, and oxaliplatin) yielding an increase in median OS up to 11.1 months compared to 6.8 months with the prior standard-of-care gemcitabine based regimens, but at the cost of greater toxicity (7). The Dutch Cancer Registration (NKR-IKNL) shows dismal survival data (8). Median OS of all patients diagnosed with metastatic PDAC (mPDAC) from 2012 to 2016 was 3.4 months, for those patients who received at least 1 cycle of chemotherapeutic treatment median OS was 6.4 months (see Table 1 and corresponding Figure 1). Nearly 30% of newly diagnosed patients with PDAC have locally advanced pancreatic carcinoma (LAPC). Due to their vascular ingrowth these tumours are considered unresectable. Patients with LAPC have a median OS of 5.1 months from the time of diagnosis (5). With the introduction of FOLFIRINOX, survival has improved and a pooled median OS from time of diagnosis of 24.2 monthswas reported in a well-conducted meta-analysis but due to inclusion of retrospective reports this outcome is probably influenced by selection and immortal time bias (9). The authors state that after first-line FOLFIRINOX, almost two-thirds of patients received additional local treatments including targeted ablation, radiotherapy, chemoradiation, and surgical resection. Although no significant association was found between OS and these additional therapies, studies reporting additional therapy in more than 50% of patients had a better median OS (24.5 months) than studies reporting additional therapies in less than 50% of patients (17.4 months) (9). More prospective data is needed before conclusions can be drawn from this observation but it carefully appears that additional local ablative treatment after chemotherapy is beneficial in selected patients.
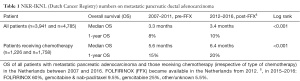
Full table
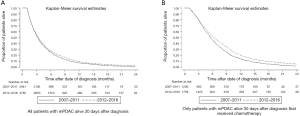
Overall, clinical treatment results remain poor for all stages of PDAC and for all standalone treatment modalities examined, including passive immunotherapy with monoclonal antibodies (mAbs) and active approaches employing checkpoint inhibitors, oncolytic viruses, toll-like receptor ligands, cytokines, adoptive cell transfer and peptide or dendritic cell (DC) vaccines. The discrepancy between preclinical data and clinical outcome motivates researchers to investigate novel combination strategies with the intent to enhance antigen presentation, provoke inflammation and inhibit checkpoints. In this regard, interventional radiology plays a pivotal role as tumour ablation may represent an attractive means to turn immunologically cold tumors, such as PDAC, into hot pro-immunogenic tumors.
This article addresses the following needle-guided interventional ablative modalities for the treatment of LAPC: radiofrequency ablation (RFA), microwave ablation (MWA), cryoablation and irreversible electroporation (IRE). In May 2019 a literature search was performed in MEDLINE for available publications. Cytoreductive and immunomodulating abilities are discussed for each technique.
Cytoreduction
The rationale for local therapy in patients with LAPC is that about 30% of patients die from local progression in the absence of metastatic disease (10). Patients who do not develop metastatic disease on chemotherapeutic treatment might therefore benefit from local control of the tumor. Local ablative therapies should only be used in patients who received chemotherapy upfront and without signs of distant progression after restaging (11). This way, chemotherapy can be used to sort out patients with biologically detrimental tumor types that are prone to metastasize and who are unlikely to benefit from local therapy (12). Patients should be evaluated in a tumor board consisting of at least medical oncologists, gastroenterologists, diagnostic and interventional radiologists, radiation oncologists and surgeons. Key papers on neelde-guided ablative cytoreduction for LAPC are summarized in Table 2.
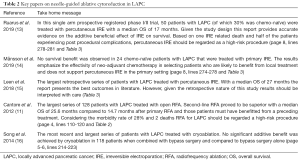
Full table
RFA
RFA leverages the development of heat for tumor destruction and cytoreduction by coagulation and protein denaturation. High-frequency alternating currents are applied usually through one needle electrode that is inserted into the core of the tumor (17). Oncological results on RFA for the treatment of LAPC, remain controversial. Several studies were published evaluating the technique however, except for one study (18), all were conducted at the university of Verona Hospital Trust. Studies were mostly retrospective and considering the overlapping date ranges patient cohorts are likely coinciding to some extent, introducing selection and population bias (11,19-23). One study used a percutaneous approach (23), 6 studies reported on RFA procedures performed during open laparotomy (11,18-21,24) and one study used an endoscopic ultrasound (EUS) guided transesophageal approach (22). The largest study by Cantore et al. compared primary RFA combined with palliative bypass surgery to RFA as a second-line treatment subsequently to chemo(radio)therapy (11). Patients receiving primary RFA had a significantly lower median OS of 14.7 months compared to 25.6 months for those where RFA was used as secondary treatment (P=0.004). The authors explain that the difference may reflect selection bias in some patients who received second-line RFA and must have benefited from a preceding treatment, being systemic chemotherapy and/or radio-chemotherapy and/or intra-arterial chemotherapy. In view of these scarce oncological results, the Dutch randomized-controlled PELICAN trial (NCT03690323), which compares OS data, is currently recruiting patients with non-progressive LAPC after 2 months of induction chemotherapy who are either treated with RFA plus chemotherapy or with chemotherapy alone, and might provide the ultimate answer in this matter.
A disadvantage of RFA in LAPC is the risk of collateral thermal damage considering the precarious location of the tumor near vital and delicate structures like the stomach, duodenum, bile ducts and several major vessels (25). Another drawback of RFA remains in the fact that elevation in tissue impedance, caused by excessive temperature heating, can inhibit further energy spreading through remaining tissue and may restrict the ablation zone (26). Additionally, it is challenging to maintain destructive temperatures if the ablated tumor is located near large blood vessels. This so called “heat-sink” effect occurs when thermal energy is dissipated from the ablation area by flowing blood or air, thereby decreasing RFA efficacy (27). The most common reported serious adverse events are gastric ulcer or fistula, acute pancreatitis, pancreatic fistula, duodenal injury, biliary injury, portal vein thrombosis, and peritoneal hemorrhages. Fegrachi et al. reported a major complication rate of 53% using the Clavien-Dindo classification and 6% mortality in a phase II study on intraoperative RFA for LAPC (18). Roughly half of these complications consisted of delayed gastric emptying (DGE), a grade 3a complication, that was treated with endoscopic feeding tube placement. The authors claimed that if DGE had not been considered a major complication (as other centers often place preventive feeding tubes intraoperatively or simply do not interpret DGE as grade 3 complication), the major complication rate would only have been 29%. This would be in line with other literature, reporting morbidity rates ranging between 0 and 28% and 30-day mortality between 0 and 3% (11,19-24). A summary of the literature of RFA for LAPC is presented in Table 3.
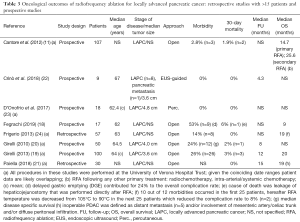
Full table
MWA
MWA produces frictional heating by the oscillation of polar molecules, which ultimately generates coagulative tissue necrosis (28). Microwaves propagate more effectively trough different tissue types like fibrous, charred and desiccated tissues and are less affected by rapid elevation in tissue impedance than radio frequency waves (29). A drawback of the technique is that heating precision and monitoring is less exquisite compared to thermal energies that are more readily absorbed (29). Literature on the use of MWA for LAPC is scarce, probably due to the controversy concerning the possible side effects of thermal ablation for this indication. Theoretically, the complex anatomy in combination with a less predictable ablation zone does not make MWA a very attractive ablative modality for LAPC. Ierardi et al. reported the treatment of 5 LAPC patients with ultrasound-guided percutaneous MWA (30). The procedure was feasible in all patients and no major complications were reported. However, contrast enhanced CT-scans at one month revealed partial responses in all patients, but progressive disease in 2 patients at 4 months according to RECIST criteria. During follow-up, 2 patients died at 6 months and 1 at 10 months as result of their primary cancer that was not further specified. Carrafiello et al. treated 5 patients percutaneously and another 5 patients with intraoperative MWA (28). Local tumor progression rates were 37.5% at 9 months and 62.5% at 1 year, according to RECIST criteria. Minor complications were reported in 20% of the patients. Major events that occurred were pancreatitis and a pseudoaneurysm of the gastroduodenal artery. Lygidakis et al. treated 15 patients with histologically proven LAPC with MWA and reported on feasibility and safety of the procedure (31). No major complications or mortality occurred related to the procedure, whilst partial necrosis was achieved in all patients.
Cryoablation
On the other side of the thermal spectrum lies cryoablation. The technique utilizes argon, or nitrogen and helium gases to induce instant freezing of the tumor tissue (32). Cryoablation causes coagulative necrosis and tumor apoptosis by direct physical damage during freezing. Coinciding tissue ischemia takes place due to progressive vasoconstriction and occlusion, and endothelial damage (32). A great advantage of cryoablation is the intraprocedural visibility of an ice ball around the tip of the cryoprobe that can be identified by ultrasound or CT and corresponds to the ablation zone. To date, 2 retrospective studies compared the safety and efficacy of cryoablation for LAPC (16,33). Li et al. compared 74 patients receiving cryoablation plus bypass surgery with 68 patients receiving only bypass surgery. Cryoablation effectively reduced tumor mass, but median OS did not significantly improve (350 versus 257 days; P=0.124) (33). Song et al. found similar results in 118 patients (5 months after cryoablation plus bypass surgery compared to 4 months after bypass surgery alone; P>0.05) (16). In both studies the post-procedural complication rates did not differ between study arms, except for increased DGE after cryoablation in the study performed by Li et al. (33). The most frequently encountered complications after intraoperative cryoablation included DGE, pancreatic or biliary leakage, pancreatitis or gastro-intestinal bleeding or obstruction (16,33).
IRE
IRE causes irreversible nanoscale defects in the cell membrane by the application of high-voltage electric pulses, leading to cell death through apoptosis and necrosis (34,35). The technique has been investigated for several solid cancer types (36) and provides a promising alternative to thermal ablation (37). IRE is based on the pulsatile application of electric energy delivered between 2 or more electrodes that are introduced in and around the tumor (34). Due to its primarily non-thermal mechanism of action, IRE leaves supporting extracellular matrix structures unaffected, preserving the structural tissue integrity of inlaying and nearby structures like vessels and bile ducts (34). This makes the technique ideal for the ablation of diffusely growing malignancies that surround these structures, such as LAPC. Several studies have investigated the safety and efficacy of open, laparoscopic, and percutaneous IRE for LAPC. Four of these studies used IRE in selected patients also as margin accentuation after surgical resection (38-41), other studies used IRE solely as primary tumor treatment. All are summarized in Table 4 (14,15,38-54). For primary IRE, median OS from diagnosis ranged between 10.2 and 27.0 months after a median follow-up of 1 to 29 months. The median OS from IRE treatment varied between 7.0 to 18.0 months. Some studies reported on the ability of IRE to downstage the tumor to resectability, ranging between 6.0% and 10.3% (15,43,46,47,55). Local progression after IRE varied between studies from 0 to 27.8% (14,15,38-42,44,46,47,50,52,53,55). Major complications (grade 3 or higher) varied between none (42) to 53% (51). Most frequently occurring complications were abdominal pain, nausea, anorexia, DGE or ileus, and (perforated) ulcers with or without gastro-intestinal bleeding, biliary stricture, portal vein thrombosis, bile leakage or cholangitis, pancreatic leakage, fistula or pancreatitis, and ascites. Reported mortality rates ranged from 0 to 13% and were caused by tumor progression, gastrointestinal (GI) bleeding from ulcer, portal vein thrombosis leading to liver failure, necrosis of the bile duct and duodenum, cardiopulmonary arrest, pneumonia, and pulmonary embolism. The large differences in survival outcomes between the studies may be explained by a variable time from diagnosis to IRE (range, 6.2–11.6 months), the exclusion of patients with occult metastases on diagnostic laparoscopy preceding open or laparoscopic IRE (38), and differing percentages of patients who were treated with upfront chemo(radio)therapy. There is increasing evidence that neo-adjuvant chemotherapy may be an effective way to select patients who are likely to benefit from IRE (12). The recently published prospective series of Månsson et al. underlined this argument as it failed to demonstrate survival benefit in 24 patients with LAPC that were treated with percutaneous IRE as first line treatment (14). On the other hand, the prospective randomized PANFIRE II trial that included 50 LAPC patients of which only two-thirds received upfront chemotherapy reports a median OS of 17 months (13). The main limitation of most studies is their retrospective nature, potentially leading to selection bias. Furthermore, overlapping patient cohorts may have induced population bias. Still, the overall improved median OS of LAPC for IRE-treated patients provides an encouraging non-variable endpoint. The ongoing randomized CROSSFIRE trial aims to compare the survival benefit of IRE to stereotactic body radiotherapy in with chemotherapy pretreated LAPC patients (NCT02791503) (56).
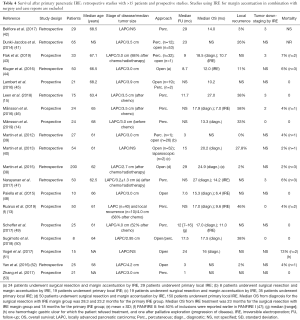
Full table
Immunomodulation
Immunogenic effects
Besides local tumor destruction, immunomodulating properties have been identified for all four needle-guided ablative modalities. Ablation may lead to systemic tumor control through the priming or boosting of tumor-specific adaptive immunity, in effect resulting in in vivo vaccination (57,58). Unlike with surgical resection, after ablative therapy the treated malignancy is not removed from the body, but apoptotic or necrotic cell remnants remain available for uptake by DCs, potentially leading to effective systemic antitumor immunity (59,60). Preclinically and clinically observed abscopal effects (i.e., tumor control at distant, untreated sites) are in line with this notion (61,62). While the exact mechanism of the abscopal effect in needle-guided ablation is not fully understood, it most likely involves the activity of specialized antigen-presenting cells (APCs), the so-called DCs. DCs regulate tolerance versus immunity (63,64). Immature DCs residing in tissues are extremely proficient in antigen uptake; however, when immature, they are poor stimulators of T cells. After exposure to endogenous danger signals released by dying cells following local ablation, i.e., damage associated molecular patterns (DAMPs), DCs mature and lose their ability to take up antigens but gain T cell stimulatory function. On the other hand, if a DC does not receive maturation signals it becomes tolerogenic, effecting the expansion of an immunosuppressive T cell subset; the regulatory T cells (Tregs) (63,64). Thus, exposure to sufficient quantities of antigens combined with maturation signals, may render DCs capable of inducing robust immune responses. Ablative tumor therapies result in massive tumor cell death, accompanied by the release of antigens, which are taken up by DCs (59,60). The release of DAMPs associated with tumor ablation properly activate the DCs, which then gain the ability to migrate to the draining lymph nodes where they may encounter and (re)activate tumor antigen-specific T cells. Subsequently, the activated tumor antigen-specific T cells may home to more distant sites in the body and eliminate (micro)metastases, thereby accounting for the aforementioned abscopal effect.
Pro-oncogenic effects
There is also increasing evidence that ablation can induce counteractive local and systemic inflammation (including cytokines IL-6 and IL-10) and pro-oncogenic growth factors (including vascular endothelial growth factor) that potentially promote aggressive tumor biology and stimulate tumor growth (65). These effects are especially seen in tumors that are treated sub-lethally. Thermally (heat or cold) ablated lesions can be divided into two zones (26). The central zone, directly next to the thermal source, is characterized by tissue coagulation and cell necrosis. These necrotic cells release DAMPs that mature DCs, thus effecting immunogenic cell death, resulting in tumor-specific immunity. The peripheral or translational zone is characterized by sub-lethal hyper- or hypo-thermia that induces cell apoptosis. These apoptotic cells may be cleared by macrophages, thus leaving insufficient DAMPs and antigens for activation and antigen uptake by DCs (an immunologically “silent” form of cell death). Immature DCs can trigger immunosuppressive signals that lead to T cell inactivation and immune tolerance (66,67). Clinical evidence for pro-oncogenic or so called off target effects of ablation exists for colorectal liver metastases, hepatocellular carcinoma, and renal tumors and has been linked to a higher rate of distant tumor development and overall worse patient outcomes (68-70). It is not yet fully understood how to induce immunogenic effects while avoiding pro-oncogenic effects. Different ablation techniques but also varying ablation parameters for the same technique might have opposite effects in the same tumor environment. Future research exploring the immunogenic effects of ablation should take the potential pro-oncogenic effects into consideration.
The pancreatic immune escape
Generally, PDAC is regarded as weakly immunogenic (71). This low immunogenic potential may in part be due to a lack of spontaneous cell death and collateral antigen release by cancer cells (72). Priming of tumor-specific T cells in PDAC is further hampered by active immune suppression perpetrated by the tumor (73). Recent reports have shown that the prevalence of suppressive Tregs is increased in several solid tumors, particularly in the tumor microenvironment (74). Data from Hiraoka et al. confirmed that Tregs indeed play a role in controlling the immune response in PDAC from the premalignant stage to established cancer (75). The prevalence of Tregs was significantly increased in the stroma of invasive PDAC compared with the stroma of non-neoplastic inflammation of the pancreas. Another important immunosuppressive subset consists of myeloid-derived suppressor cells (MDSCs), a heterogeneous population of immature myeloid cells that are increased in cancer patients and converted to highly suppressive cells (76). Gabitass et al. demonstrated a significant increase in circulating MDSCs in patients with PDAC which correlated with an elevation in Tregs and was an independent prognostic factor (77). Key papers on needle-guided ablative immunomodulation fin PDAC are summarized in Table 5.
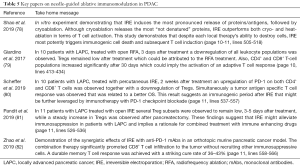
Full table
Evidence of ablative immunomodulation in PDAC
RFA
The ability of RFA to modulate the immune system and to induce anti-tumor immune responses has been demonstrated in several tumor types, such as hepatocellular carcinoma (83), colorectal and other metastatic liver tumors (77,84) and lung carcinoma (85). However, evidence for the advantageous immunomodulatory effects of RFA in PDAC remains scarce. RFA causes protein denaturation, cell necrosis, and vessel disruption through hyperthermia. The heat upregulates heat shock protein 70 (HSP-70) (86) that plays an important role in the activation of naive DCs (59). RFA can thus raise the frequency of activated DCs in PDAC leading to an adaptive T-cell response (87). Giardino et al. published the only clinical data on the influence of RFA for PDAC on the immune system (79). Peripheral blood samples of 10 patients with LAPC showed a systemic immune response after intra-operative RFA, which was attributed to a possible transitional zone of apoptosis-undergoing tumor tissue exposing tumor-specific antigens. Immediately following RFA (day 3) a downregulation of all leukocytic populations was observed, which can be either due to surgically-induced immunosuppression or the RFA treatment (88). After 30 days, CD4+ and CD8+ T-cell populations increased significantly compared to day 3, implying the activation of an adaptive T-cell response. However, there was no significant difference between pre-RFA (day 0) and frequencies at day 30, raising the question whether the CD4+ and CD8+ T cells had simply returned to baseline after being downregulated at day 3. Immunosuppressive Tregs remained stable 3 daysafter RFA, which was considered remarkable since laparotomy and heating could have favored their expansion. The authors speculate that RFA downregulates these immunosuppressive cells (79).
MWA
The first clinical immunomodulatory effects of MWA were found in patients with prostate cancer, where a transient increase in CD4+/CD8+ ratio was observed most severely at 2 months after ablation (89). Since MWA is often used for liver tumors, multiple studies investigating immunomodulation regarding this ablative modality have been performed in this organ. Increased infiltration rates of immune stimulatory cells like CD4+ T cells, CD8+ T cells, monocytes, NK cells were observed in biopsies of the ablation zone (90,91) and increased levels of CD3+, CD4+ and IL-12 were found in peripheral blood up to 30 days after the treatment whilst the immunosuppressive cytokines IL-4 and IL-10 decreased significantly (92). Most strikingly an increased OS and decreased recurrence rate were both associated with a high extent of immune cell infiltration (93). Studies investigating the immune response related to MWA for PDAC have yet to be performed.
Cryoablation
Of all needle-guided ablation methods the influence of cryoablation on the immune system has been studied most extensively and evidence for both immunostimulatory (94,95) and immunosuppressive (96) responses has been published suggesting that the extent to which the effects occur is subject to several factors like organ type, tumor type and ablation parameters. Nevertheless, robust preclinical work demonstrated that cryoablation induces an effective DAMP release and antigen depot resulting in maturation of DCs in a B16 mouse model. This leads to tumor-specific immune responses that protected half of the ablated mice against tumor challenge (59). In vitro results comparing the quality and quantity of proteins released by cryoablation versus heat-based ablation showed that cryoablation outperformed heat in terms of total protein release and of native (not denatured) protein release ensuing more effective priming of the immune system (78). The immunomodulatory effects have also been compared in the peripheral blood samples of patients with different tumor types by Takaki et al. The authors reported no change in Tregs, T1 or T2 helper cells before and after ablation for both modalities. However, there was an elevation of CD8+ T cells after heat-based ablation, but none was observed after cryoablation leading to the authors conclusion that heat-based ablation might be more effective to enhance systemic anti-tumor immunity (97). Clinical evidence of the immunomodulatory effects of cryoablation in PDAC is however nonexistent.
IRE
Two characteristics of IRE potentially make the technique a good candidate for the induction of a systemic antitumor immune response in PDAC. IRE-induced cell death and reduced tumor load, may lead to a simultaneous release of immunogenic tumor cell remnants and a reduction in tumor-associated immunosuppression. Secondly, because the larger vessels remain intact, APCs like DCs, with the ability to induce an immune response against the released tumor antigens, as well as subsequently primed and recruited effector T cells, should be able to infiltrate the lesion (34,98). Vessel patency could represent a great advantage compared to thermal ablation which destroys microvasculature. This was demonstrated by Bulvik et al. in an animal model, where leukocyte infiltration into the ablation zone was present in IRE-treated, but not in RFA-treated lesions (62).
The ability of IRE to induce immunogenic cell death has been investigated in vitro in B16 melanoma cell lysates and was compared to that of cryotherapy and heat-based ablation (78). After viability assessment cell lysates were collected and assessed for protein release, protein denaturation, antigen release, and T cell activation (antigen-specific CD8+ T cell proliferation). Results showed that IRE induced the most pronounced release of proteins/antigens, followed by cryoablation. Although cryoablation released the most “not denatured” proteins, IRE outperformed both cryo- and heat-ablation in terms of T cell activation. This study demonstrates that despite each local therapy’s ability to destroy cells, IRE most potently triggers immunogenic cell death and subsequent T cell induction (78).
In vivo evidence underlines this observation. In mice bearing implanted pancreatic tumors, IRE induced an increase in infiltrating macrophages and T cells that was 2 times greater compared to cryoablation at 6 hours, 12 hours and 24 hours after treatment (99).
To date 2 groups published clinical data about the immunomodulatory effects of IRE in patients with LAPC. Pandit et al. analyzed blood samples by flow cytometry of 15 patients for 3 clinically correlated Treg populations (81). In this study one group underwent intraoperative IRE (n=11) and the other group (n=4) underwent a pancreatectomy. Blood was drawn before treatment, 1 day after treatment and between 3 and 5 days after treatment. In both groups, all Treg subsets decreased 1 day after treatment followed by a steady increase in the pancreatectomy group, whereas Treg levels remained low in the IRE group between day 3 and day 5. This suggests that IRE may alleviate immunosuppression in LAPC.
Scheffer et al. performed flowcytometric profiling on the blood of 10 patients with LAPC who were treated with percutaneous IRE. Blood was collected pre-treatment, 2 weeks and 3 months after IRE (80). Frequencies and activation states of several immune subsets were analyzed, including CD4+ T cells, CD8+ T cells, Tregs, conventional and plasmacytoid DCs, monocytes, and MDSCs. At 2 weeks post IRE a transient decrease in systemic Treg rates and a simultaneous transient increase in activated PD-1+ T cells was found, consistent with the temporary reduction of tumor-related immunosuppression after IRE. The authors also found post-IRE boosting of a pre-existing Wilms tumor 1 (WT1) antigen, expressed by 75% of pancreatic tumors (100), specific T cell response by IFN-γ Elispot read-out in 2 out of 3 patients as well as a de novo induction of these responses in another 2 patients. There was a trend for these WT1 T cell responses to be related to longer OS (P=0.055). These findings demonstrate a systemic and tumor-specific immune stimulatory effect of IRE and in addition to the results of Pandit et al. show that the effect endures for at least 2 weeks.
Elaborating on these observations Zhao et al. demonstrated in an orthotopic murine pancreatic cancer model that IRE combined with checkpoint inhibition with anti-PD-1 mAbs promoted tumor infiltration by CD8+ cytotoxic T cells without recruiting other immunosuppressive cells to the tumor. This treatment combination significantly prolonged survival. Most strikingly, the IRE + anti-PD-1 treatment achieved a cure rate of 36–43% with a durable memory T cell response (82).
Future perspectives
Cancer immunotherapy is based on the premise that tumors can be recognized as foreign and can be effectively attacked by an activated immune system. Efficacy of immunotherapy in PDAC remains limited, probably due to this tumor type’s immunosuppressive microenvironment. Local ablation techniques may affect both the immunogenic release of pancreatic tumor-derived antigens and the alleviation of tumor-associated immunosuppression, thus achieving in vivo vaccination against the tumor.
The clinical findings presented by Giardino et al., Pandit et al., and Scheffer et al. and the preclinical work published by Zhao et al. support the combination of pancreatic tumor ablation with therapeutic immunomodulation (79-82). Ablation may provide a window of opportunity for potentiating the clinical efficacy of immunotherapy. In particular, the observation of transiently increased PD-1 expression on T cells after IRE in patients with LAPC (80) and the durable memory T cell response achieved in mice (82) point towards IRE as a particularly powerful technique in this regard. The studies strongly support the combined application of IRE with check point inhibition for PDAC (see Figure 2 for a research setup example combining IRE ablation with intratumoral and/or systemic immunotherapy).
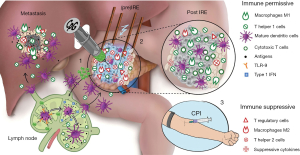
Several factors concerning the combination of ablation and immunotherapy remain uncertain. Especially the timing and sequence of ablation and the application of immune stimulatory drugs seem to be of importance and warrant further investigation (101).
Conclusions
This review gave an overview of the currently available literature on needle-guided ablative techniques for the treatment of patients with LAPC and their effects on the immune system. In general, the safety profile of pancreatic ablation is defined as acceptable. However, major adverse events do occur and even mortality has been reported for all ablative techniques. High-quality evidence for survival benefit for any local ablative modality over chemotherapy alone is scarce. Nevertheless, the promising survival in several studies where effective chemo(radio)therapy preceded ablation, warrants completion of the ongoing randomized controlled phase III trials to provide closure on needle-guided ablation’s additive value. Emerging preclinical and clinical data on the capability of ablation to induce in vivo vaccination should motivate researchers to design smart studies with combinations of local ablation and immunotherapies to investigate their efficacy and to fill remaining knowledge gaps concerning timing and sequencing. Effective combination of ablation and immunotherapy could hypothetically turn the immune suppressed tumor microenvironment of PDAC into an immune permissive environment and ultimately result in a longer lasting survival benefit for patients.
Acknowledgments
The authors thank the registration team of The Netherlands Comprehensive Cancer Organization (IKNL) for the collection of data for The Netherlands Cancer Registry as well as IKNL staff for scientific advice and their support in providing Figure 1. Figure 2 was illustrated by Dana Hamers, Scientific Art and composed with financial support of AngioDynamics.
Footnote
Conflicts of Interest: MR Meijerink is a paid consultant for AngioDynamics. The other authors have no conflicts of interest to declare.
Ethical Statement: The authors are accountable for all aspects of the work in ensuring that questions related to the accuracy or integrity of any part of the work are appropriately investigated and resolved.
References
- Siegel RL, Miller KD, Jemal A. Cancer statistics, 2019. CA Cancer J Clin 2019;69:7-34. [Crossref] [PubMed]
- Kamisawa T, Wood LD, Itoi T, et al. Pancreatic cancer. Lancet 2016;388:73-85. [Crossref] [PubMed]
- Ferrone CR, Pieretti-Vanmarcke R, Bloom JP, et al. Pancreatic ductal adenocarcinoma: long-term survival does not equal cure. Surgery 2012;152:S43-9. [Crossref] [PubMed]
- Katz MH, Wang H, Fleming JB, et al. Long-term survival after multidisciplinary management of resected pancreatic adenocarcinoma. Ann Surg Oncol 2009;16:836-47. [Crossref] [PubMed]
- van Erning FN, Mackay TM, van der Geest LGM, et al. Association of the location of pancreatic ductal adenocarcinoma (head, body, tail) with tumor stage, treatment, and survival: a population-based analysis. Acta Oncol 2018;57:1655-62. [Crossref] [PubMed]
- Niederhuber JE, Brennan MF, Menck HR. The national cancer data base report on pancreatic cancer. Cancer 1995;76:1671-7. [Crossref] [PubMed]
- Conroy T, Desseigne F, Ychou M, et al. FOLFIRINOX versus gemcitabine for metastatic pancreatic cancer. N Engl J Med 2011;364:1817-25. [Crossref] [PubMed]
- Netherlands Comprehensive Cancer Organisation (IKNL). 2019. Available online: http://www.iknl.nl/netherlands-cancer-registryNetherlands Comprehensive Cancer Organisation (IKNL). 2019.
- Suker M, Beumer BR, Sadot E, et al. FOLFIRINOX for locally advanced pancreatic cancer: a systematic review and patient-level meta-analysis. Lancet Oncol 2016;17:801-10. [Crossref] [PubMed]
- Iacobuzio-Donahue CA, Fu B, Yachida S, et al. DPC4 gene status of the primary carcinoma correlates with patterns of failure in patients with pancreatic cancer. J Clin Oncol 2009;27:1806-13. [Crossref] [PubMed]
- Cantore M, Girelli R, Mambrini A, et al. Combined modality treatment for patients with locally advanced pancreatic adenocarcinoma. Br J Surg 2012;99:1083-8. [Crossref] [PubMed]
- Khushman M, Dempsey N, Maldonado JC, et al. Full dose neoadjuvant FOLFIRINOX is associated with prolonged survival in patients with locally advanced pancreatic adenocarcinoma. Pancreatology 2015;15:667-73. [Crossref] [PubMed]
- Ruarus AH, Vroomen LGPH, Geboers B, et al. Percutaneous Irreversible Electroporation in Locally Advanced and Recurrent Pancreatic Cancer (PANFIRE-2): A Multicenter, Prospective, Single-Arm, Phase II Study. Radiology 2020;294:212-20. [PubMed]
- Månsson C, Brahmstaedt R, Nygren P, et al. Percutaneous Irreversible Electroporation as First-line Treatment of Locally Advanced Pancreatic Cancer. Anticancer Res 2019;39:2509-12. [Crossref] [PubMed]
- Leen E, Picard J, Stebbing J, et al. Percutaneous irreversible electroporation with systemic treatment for locally advanced pancreatic adenocarcinoma. J Gastrointest Oncol 2018;9:275-81. [Crossref] [PubMed]
- Song ZG, Hao JH, Gao S, et al. The outcome of cryoablation in treating advanced pancreatic cancer: a comparison with palliative bypass surgery alone. J Dig Dis 2014;15:561-9. [Crossref] [PubMed]
- Ahmed M, Brace CL, Lee FT Jr, et al. Principles of and advances in percutaneous ablation. Radiology 2011;258:351-69. [Crossref] [PubMed]
- Fegrachi S, Walma MS, de Vries JJJ, et al. Safety of radiofrequency ablation in patients with locally advanced, unresectable pancreatic cancer: A phase II study. Eur J Surg Oncol 2019;45:2166-72. [Crossref] [PubMed]
- Girelli R, Frigerio I, Giardino A, et al. Results of 100 pancreatic radiofrequency ablations in the context of a multimodal strategy for stage III ductal adenocarcinoma. Langenbecks Arch Surg 2013;398:63-9. [Crossref] [PubMed]
- Girelli R, Frigerio I, Salvia R, et al. Feasibility and safety of radiofrequency ablation for locally advanced pancreatic cancer. Br J Surg 2010;97:220-5. [Crossref] [PubMed]
- Paiella S, Malleo G, Cataldo I, et al. Radiofrequency ablation for locally advanced pancreatic cancer: SMAD4 analysis segregates a responsive subgroup of patients. Langenbecks Arch Surg 2018;403:213-20. [PubMed]
- Crinò SF, D'Onofrio M, Bernardoni L, et al. EUS-guided Radiofrequency Ablation (EUS-RFA) of Solid Pancreatic Neoplasm Using an 18-gauge Needle Electrode: Feasibility, Safety, and Technical Success. J Gastrointestin Liver Dis 2018;27:67-72. [PubMed]
- D'Onofrio M, Crosara S, De Robertis R, et al. Percutaneous Radiofrequency Ablation of Unresectable Locally Advanced Pancreatic Cancer: Preliminary Results. Technol Cancer Res Treat 2017;16:285-94. [Crossref] [PubMed]
- Frigerio I, Girelli R, Giardino A, et al. Short term chemotherapy followed by radiofrequency ablation in stage III pancreatic cancer: results from a single center. J Hepatobiliary Pancreat Sci 2013;20:574-7. [Crossref] [PubMed]
- Pezzilli R, Serra C, Ricci C, et al. Radiofrequency ablation for advanced ductal pancreatic carcinoma: is this approach beneficial for our patients? A systematic review. Pancreas 2011;40:163-5. [Crossref] [PubMed]
- Chu KF, Dupuy DE. Thermal ablation of tumours: biological mechanisms and advances in therapy. Nat Rev Cancer 2014;14:199-208. [Crossref] [PubMed]
- Whelan WM, Wyman DR, Wilson BC. Investigations of large vessel cooling during interstitial laser heating. Med Phys 1995;22:105-15. [Crossref] [PubMed]
- Carrafiello G, Ierardi AM, Fontana F, et al. Microwave ablation of pancreatic head cancer: safety and efficacy. J Vasc Interv Radiol 2013;24:1513-20. [Crossref] [PubMed]
- Brace CL. Microwave tissue ablation: biophysics, technology, and applications. Crit Rev Biomed Eng 2010;38:65-78. [Crossref] [PubMed]
- Ierardi AM, Biondetti P, Coppola A, et al. Percutaneous microwave thermosphere ablation of pancreatic tumours. Gland Surg 2018;7:59-66. [Crossref] [PubMed]
- Lygidakis NJ, Sharma SK, Papastratis P, et al. Microwave ablation in locally advanced pancreatic carcinoma--a new look. Hepatogastroenterology 2007;54:1305-10. [PubMed]
- Cazzato RL, Garnon J, Ramamurthy N, et al. Percutaneous image-guided cryoablation: current applications and results in the oncologic field. Med Oncol 2016;33:140. [Crossref] [PubMed]
- Li J, Chen X, Yang H, et al. Tumour cryoablation combined with palliative bypass surgery in the treatment of unresectable pancreatic cancer: a retrospective study of 142 patients. Postgrad Med J 2011;87:89-95. [Crossref] [PubMed]
- Rubinsky B, Onik G, Mikus P. Irreversible electroporation: a new ablation modality--clinical implications. Technol Cancer Res Treat 2007;6:37-48. [Crossref] [PubMed]
- Ringel-Scaia VM, Beitel-White N, Lorenzo MF, et al. High-frequency irreversible electroporation is an effective tumor ablation strategy that induces immunologic cell death and promotes systemic anti-tumor immunity. EBioMedicine 2019;44:112-25. [Crossref] [PubMed]
- Ruarus A, Vroomen L, Puijk R, et al. Locally Advanced Pancreatic Cancer: A Review of Local Ablative Therapies. Cancers (Basel) 2018. [Crossref] [PubMed]
- Scheffer HJ, Nielsen K, de Jong MC, et al. Irreversible electroporation for nonthermal tumor ablation in the clinical setting: a systematic review of safety and efficacy. J Vasc Interv Radiol 2014;25:997-1011. [Crossref] [PubMed]
- Martin RC 2nd, Kwon D, Chalikonda S, et al. Treatment of 200 locally advanced (stage III) pancreatic adenocarcinoma patients with irreversible electroporation: safety and efficacy. Ann Surg 2015;262:486-94. [Crossref] [PubMed]
- Martin RC 2nd, McFarland K, Ellis S, et al. Irreversible electroporation therapy in the management of locally advanced pancreatic adenocarcinoma. J Am Coll Surg 2012;215:361-9. [Crossref] [PubMed]
- Martin RC 2nd, McFarland K, Ellis S, et al. Irreversible electroporation in locally advanced pancreatic cancer: potential improved overall survival. Ann Surg Oncol 2013;20 Suppl 3:S443-9. [Crossref] [PubMed]
- Dunki-Jacobs EM, Philips P, Martin RC 2nd. Evaluation of resistance as a measure of successful tumor ablation during irreversible electroporation of the pancreas. J Am Coll Surg 2014;218:179-87. [Crossref] [PubMed]
- Belfiore G, Belfiore MP, Reginelli A, et al. Concurrent chemotherapy alone versus irreversible electroporation followed by chemotherapy on survival in patients with locally advanced pancreatic cancer. Med Oncol 2017;34:38. [Crossref] [PubMed]
- Flak RV, Stender MT, Jensen TM, et al. Treatment of locally advanced pancreatic cancer with irreversible electroporation - a Danish single center study of safety and feasibility. Scand J Gastroenterol 2019;54:252-8. [Crossref] [PubMed]
- Kluger MD, Epelboym I, Schrope BA, et al. Single-Institution Experience with Irreversible Electroporation for T4 Pancreatic Cancer: First 50 Patients. Ann Surg Oncol 2016;23:1736-43. [Crossref] [PubMed]
- Lambert L, Horejs J, Krska Z, et al. Treatment of locally advanced pancreatic cancer by percutaneous and intraoperative irreversible electroporation: general hospital cancer center experience. Neoplasma 2016;63:269-73. [PubMed]
- Månsson C, Brahmstaedt R, Nilsson A, et al. Percutaneous irreversible electroporation for treatment of locally advanced pancreatic cancer following chemotherapy or radiochemotherapy. Eur J Surg Oncol 2016;42:1401-6. [Crossref] [PubMed]
- Narayanan G, Hosein PJ, Beulaygue IC, et al. Percutaneous Image-Guided Irreversible Electroporation for the Treatment of Unresectable, Locally Advanced Pancreatic Adenocarcinoma. J Vasc Interv Radiol 2017;28:342-8. [Crossref] [PubMed]
- Paiella S, Butturini G, Frigerio I, et al. Safety and feasibility of Irreversible Electroporation (IRE) in patients with locally advanced pancreatic cancer: results of a prospective study. Dig Surg 2015;32:90-7. [Crossref] [PubMed]
- Scheffer HJ, Vroomen LG, de Jong MC, et al. Ablation of Locally Advanced Pancreatic Cancer with Percutaneous Irreversible Electroporation: Results of the Phase I/II PANFIRE Study. Radiology 2017;282:585-97. [Crossref] [PubMed]
- Sugimoto K, Moriyasu F, Tsuchiya T, et al. Irreversible Electroporation for Nonthermal Tumor Ablation in Patients with Locally Advanced Pancreatic Cancer: Initial Clinical Experience in Japan. Intern Med 2018;57:3225-31. [Crossref] [PubMed]
- Vogel JA, Rombouts SJ, de Rooij T, et al. Induction Chemotherapy Followed by Resection or Irreversible Electroporation in Locally Advanced Pancreatic Cancer (IMPALA): A Prospective Cohort Study. Ann Surg Oncol 2017;24:2734-43. [Crossref] [PubMed]
- Yan L, Chen YL, Su M, et al. A Single-institution Experience with Open Irreversible Electroporation for Locally Advanced Pancreatic Carcinoma. Chin Med J (Engl) 2016;129:2920-5. [Crossref] [PubMed]
- Zhang Y, Shi J, Zeng J, et al. Percutaneous Irreversible Electroporation for Ablation of Locally Advanced Pancreatic Cancer: Experience From a Chinese Institution. Pancreas 2017;46:e12-4. [Crossref] [PubMed]
- Narayanan G, Bhatia S, Echenique A, et al. Vessel patency post irreversible electroporation. Cardiovasc Intervent Radiol 2014;37:1523-9. [Crossref] [PubMed]
- Belfiore MP, Ronza FM, Romano F, et al. Percutaneous CT-guided irreversible electroporation followed by chemotherapy as a novel neoadjuvant protocol in locally advanced pancreatic cancer: Our preliminary experience. Int J Surg 2015;21 Suppl 1:S34-9. [Crossref] [PubMed]
- CROSSFIRE Trial: Comparing the Efficacy of Irreversible Electroporation With Radiotherapy. Available online: https://ClinicalTrials.gov/show/NCT02791503
- Bastianpillai C, Petrides N, Shah T, et al. Harnessing the immunomodulatory effect of thermal and non-thermal ablative therapies for cancer treatment. Tumour Biol 2015;36:9137-46. [Crossref] [PubMed]
- O'Brien MA, Power DG, Clover AJ, et al. Local tumour ablative therapies: opportunities for maximising immune engagement and activation. Biochim Biophys Acta 2014;1846:510-23. [PubMed]
- den Brok MH, Sutmuller RP, Nierkens S, et al. Efficient loading of dendritic cells following cryo and radiofrequency ablation in combination with immune modulation induces anti-tumour immunity. Br J Cancer 2006;95:896-905. [Crossref] [PubMed]
- Shan CC, Shi LR, Ding MQ, et al. Cytokine-induced killer cells co-cultured with dendritic cells loaded with the protein lysate produced by radiofrequency ablation induce a specific antitumor response. Oncol Lett 2015;9:1549-56. [Crossref] [PubMed]
- Dromi SA, Walsh MP, Herby S, et al. Radiofrequency ablation induces antigen-presenting cell infiltration and amplification of weak tumor-induced immunity. Radiology 2009;251:58-66. [Crossref] [PubMed]
- Bulvik BE, Rozenblum N, Gourevich S, et al. Irreversible Electroporation versus Radiofrequency Ablation: A Comparison of Local and Systemic Effects in a Small-Animal Model. Radiology 2016;280:413-24. [Crossref] [PubMed]
- Palucka K, Banchereau J. Dendritic-cell-based therapeutic cancer vaccines. Immunity 2013;39:38-48. [Crossref] [PubMed]
- Garg AD, Romano E, Rufo N, et al. Immunogenic versus tolerogenic phagocytosis during anticancer therapy: mechanisms and clinical translation. Cell Death Differ 2016;23:938-51. [Crossref] [PubMed]
- Erinjeri JP, Fine GC, Adema GJ, et al. Immunotherapy and the Interventional Oncologist: Challenges and Opportunities-A Society of Interventional Oncology White Paper. Radiology 2019;292:25-34. [Crossref] [PubMed]
- Chan JK, Roth J, Oppenheim JJ, et al. Alarmins: awaiting a clinical response. J Clin Invest 2012;122:2711-9. [Crossref] [PubMed]
- Basu S, Binder RJ, Suto R, et al. Necrotic but not apoptotic cell death releases heat shock proteins, which deliver a partial maturation signal to dendritic cells and activate the NF-kappa B pathway. Int Immunol 2000;12:1539-46. [Crossref] [PubMed]
- Kang TW, Kim JM, Rhim H, et al. Small Hepatocellular Carcinoma: Radiofrequency Ablation versus Nonanatomic Resection--Propensity Score Analyses of Long-term Outcomes. Radiology 2015;275:908-19. [Crossref] [PubMed]
- Tanis E, Nordlinger B, Mauer M, et al. Local recurrence rates after radiofrequency ablation or resection of colorectal liver metastases. Analysis of the European Organisation for Research and Treatment of Cancer #40004 and #40983. Eur J Cancer 2014;50:912-9. [Crossref] [PubMed]
- Taylor M, Rothwax J, Linehan WM, et al. #SUO14 - Session Highlights: Accelerated growth rate of multifocal tumors after initial radiofrequency ablation. Available online: https://www.urotoday.com/recent-abstracts/urologic-oncology/renal-cancer/77186-suo14-session-highlights-accelerated-growth-rate-of-multifocal-tumors-after-initial-radiofrequency-ablation.html. Published 2014.
- Sideras K, Braat H, Kwekkeboom J, et al. Role of the immune system in pancreatic cancer progression and immune modulating treatment strategies. Cancer Treat Rev 2014;40:513-22. [Crossref] [PubMed]
- Lutz ER, Wu AA, Bigelow E, et al. Immunotherapy converts nonimmunogenic pancreatic tumors into immunogenic foci of immune regulation. Cancer Immunol Res 2014;2:616-31. [Crossref] [PubMed]
- Schnurr M, Duewell P, Bauer C, et al. Strategies to relieve immunosuppression in pancreatic cancer. Immunotherapy 2015;7:363-76. [Crossref] [PubMed]
- Li M, Bharadwaj U, Zhang R, et al. Mesothelin is a malignant factor and therapeutic vaccine target for pancreatic cancer. Mol Cancer Ther 2008;7:286-96. [Crossref] [PubMed]
- Hiraoka N, Onozato K, Kosuge T, et al. Prevalence of FOXP3+ regulatory T cells increases during the progression of pancreatic ductal adenocarcinoma and its premalignant lesions. Clin Cancer Res 2006;12:5423-34. [Crossref] [PubMed]
- Diaz-Montero CM, Salem ML, Nishimura MI, et al. Increased circulating myeloid-derived suppressor cells correlate with clinical cancer stage, metastatic tumor burden, and doxorubicin-cyclophosphamide chemotherapy. Cancer Immunol Immunother 2009;58:49-59. [Crossref] [PubMed]
- Gabitass RF, Annels NE, Stocken DD, et al. Elevated myeloid-derived suppressor cells in pancreatic, esophageal and gastric cancer are an independent prognostic factor and are associated with significant elevation of the Th2 cytokine interleukin-13. Cancer Immunol Immunother 2011;60:1419-30. [Crossref] [PubMed]
- Shao Q, O'Flanagan S, Lam T, et al. Engineering T cell response to cancer antigens by choice of focal therapeutic conditions. Int J Hyperthermia 2019;36:130-8. [Crossref] [PubMed]
- Giardino A, Innamorati G, Ugel S, et al. Immunomodulation after radiofrequency ablation of locally advanced pancreatic cancer by monitoring the immune response in 10 patients. Pancreatology 2017;17:962-6. [Crossref] [PubMed]
- Scheffer HJ, Stam AG, Geboers B, et al. Irreversible electroporation of locally advanced pancreatic cancer transiently alleviates immune suppression and creates a window for antitumor T cell activation. OncoImmunology 2019. [Crossref] [PubMed]
- Pandit H, Hong YK, Li Y, et al. Evaluating the Regulatory Immunomodulation Effect of Irreversible Electroporation (IRE) in Pancreatic Adenocarcinoma. Ann Surg Oncol 2019;26:800-6. [Crossref] [PubMed]
- Zhao J, Wen X, Tian L, et al. Irreversible electroporation reverses resistance to immune checkpoint blockade in pancreatic cancer. Nat Commun 2019;10:899. [Crossref] [PubMed]
- Zerbini A, Pilli M, Laccabue D, et al. Radiofrequency thermal ablation for hepatocellular carcinoma stimulates autologous NK-cell response. Gastroenterology 2010;138:1931-42. [Crossref] [PubMed]
- Hansler J, Wissniowski TT, Schuppan D, et al. Activation and dramatically increased cytolytic activity of tumor specific T lymphocytes after radio-frequency ablation in patients with hepatocellular carcinoma and colorectal liver metastases. World J Gastroenterol 2006;12:3716-21. [Crossref] [PubMed]
- Fietta AM, Morosini M, Passadore I, et al. Systemic inflammatory response and downmodulation of peripheral CD25+Foxp3+ T-regulatory cells in patients undergoing radiofrequency thermal ablation for lung cancer. Hum Immunol 2009;70:477-86. [Crossref] [PubMed]
- Teng LS, Jin KT, Han N, et al. Radiofrequency ablation, heat shock protein 70 and potential anti-tumor immunity in hepatic and pancreatic cancers: a minireview. Hepatobiliary Pancreat Dis Int 2010;9:361-5. [PubMed]
- Rovere-Querini P, Manfredi AA. Tumor destruction and in situ delivery of antigen presenting cells promote anti-neoplastic immune responses: implications for the immunotherapy of pancreatic cancer. JOP 2004;5:308-14. [PubMed]
- Novitsky YW, Litwin DE, Callery MP. The net immunologic advantage of laparoscopic surgery. Surg Endosc 2004;18:1411-9. [Crossref] [PubMed]
- Szmigielski S, Sobczynski J, Sokolska G, et al. Effects of local prostatic hyperthermia on human NK and T cell function. Int J Hyperthermia 1991;7:869-80. [Crossref] [PubMed]
- Dong B, Zhang J, Liang P, et al. Influencing factors of local immunocyte infiltration in hepatocellular carcinoma tissues pre- and post-percutaneous microwave coagulation therapy. Zhonghua Yi Xue Za Zhi 2002;82:393-7. [PubMed]
- Zhang J, Dong B, Liang P, et al. Significance of changes in local immunity in patients with hepatocellular carcinoma after percutaneous microwave coagulation therapy. Chin Med J (Engl) 2002;115:1367-71. [PubMed]
- Zhang H, Hou X, Cai H, et al. Effects of microwave ablation on T-cell subsets and cytokines of patients with hepatocellular carcinoma. Minim Invasive Ther Allied Technol 2017;26:207-11. [Crossref] [PubMed]
- Dong BW, Zhang J, Liang P, et al. Sequential pathological and immunologic analysis of percutaneous microwave coagulation therapy of hepatocellular carcinoma. Int J Hyperthermia 2003;19:119-33. [Crossref] [PubMed]
- Blackwood CE, Cooper IS. Response of experimental tumor systems to cryosurgery. Cryobiology 1972;9:508-15; discussion 492-4. [Crossref] [PubMed]
- Bagley DH, Faraci RP, Marrone JC, et al. Lymphocyte mediated cytotoxicity after cryosurgery of a murine sarcoma. J Surg Res 1974;17:404-6. [Crossref] [PubMed]
- Hoffmann NE, Coad JE, Huot CS, et al. Investigation of the mechanism and the effect of cryoimmunology in the Copenhagen rat. Cryobiology 2001;42:59-68. [Crossref] [PubMed]
- Takaki H, Imai N, Thomas CT, et al. Changes in peripheral blood T-cell balance after percutaneous tumor ablation. Minim Invasive Ther Allied Technol 2017;26:331-7. [Crossref] [PubMed]
- José A, Sobrevals L, Ivorra A, et al. Irreversible electroporation shows efficacy against pancreatic carcinoma without systemic toxicity in mouse models. Cancer Lett 2012;317:16-23. [Crossref] [PubMed]
- White SB, Zhang Z, Chen J, et al. Early Immunologic Response of Irreversible Electroporation versus Cryoablation in a Rodent Model of Pancreatic Cancer. J Vasc Interv Radiol 2018;29:1764-9. [Crossref] [PubMed]
- Oji Y, Nakamori S, Fujikawa M, et al. Overexpression of the Wilms' tumor gene WT1 in pancreatic ductal adenocarcinoma. Cancer Sci 2004;95:583-7. [Crossref] [PubMed]
- Silvestrini MT, Ingham ES, Mahakian LM, et al. Priming is key to effective incorporation of image-guided thermal ablation into immunotherapy protocols. JCI Insight 2017;2:e90521. [Crossref] [PubMed]