Strategies for preservation of memory function in patients with brain metastases
Introduction
Brain metastases account for the majority of intracranial malignancies and carry a high mortality. However, with improvements in treatment including surgery, chemotherapy, and radiotherapy, patients are living longer. In spite of these observations, for an unselected population of patients with brain metastases, which would represent the vast majority of these patients, median survival is still measured in months, rather than years. Further, the treatment regimens and the disease itself can have a significant impact on a patient’s cognition and quality of life. One of the most debilitating side effects associated with brain metastases is neurocognitive decline, most notably memory impairment.
The underlying pathology of cognitive decline in patients with brain metastases is multifactorial. A common cause is the cancer itself, and of course the occurrence of brain metastases. Medications associated with the symptomatic management of the consequences of brain metastases, systemic chemotherapeutic agents, and whole brain radiotherapies (WBRT) are contributory factors. Additionally, comorbid factors such as anxiety, fatigue, depression and tumor involvement of memory centers in the brain can contribute to memory decline. Various techniques have been and are being developed to prevent or treat neurocognitive decline in brain cancer patients. This review will focus on the strategies for memory preservation in patients with brain metastases as well as the treatment of neurocognitive decline.
Cognitive decline in patients with brain metastases: causes, prevention, and treatment
WBRT as a co-factor for memory decline
WBRT has been an integral part in the treatment of brain metastases for over half a century. Chao et al. [1954] demonstrated a 63% symptomatic improvement in patients treated with palliative WBRT (1). Improvements in surgical and radiosurgical techniques have increasingly led to applying WBRT as an adjuvant treatment following resection or radiosurgery to improve local, leptomeningeal, and regional control (2,3). Over the last decade, an increasing trend toward utilizing stereotactic radiosurgery (SRS) alone for patients with multiple brain metastases, and reserving WBRT as a treatment of “last resort” for salvage of widespread intracranial metastatic disease has emerged. Prophylactic WBRT is also employed in the treatment of malignancies with a high propensity for microscopic CNS spread such as small cell lung cancer (SCLC), where not only does WBRT decrease intracranial relapse, but doing so actually leads to a direct improvement in overall survival; further, in non-small cell lung cancer, prophylactic WBRT has categorically demonstrated a decrease in intracranial relapse as well, but without a corresponding increase in overall survival, a reflection of the need to be selective in its judicious application only to the highest risk subsets (4).
The use of WBRT in brain metastases can be associated with neuro-toxicity. Particularly detrimental to the patient’s neurocognitive function is the decline in declarative memory. Chang et al. [2009] compared patients treated with SRS alone to patients treated with SRS and WBRT, showing a greater decline in memory [as demonstrated by the Hopkins Verbal Learning Test-Revised (HVLT-R)] at 4 months, in the SRS plus WBRT group (5).
This decline in memory has been described as part of a biphasic pattern of cognitive loss. Following WBRT there is an initial deterioration in multiple domains of cognitive function that peaks around 4 months post treatment after which there is a transient recovery over the first year. The initial decline is thought to be due to damage to the white matter secondary to vascular injury and inflammation followed by remyelination, as well as loss of radiosensitive stem cells, mediated in part by a pro-inflammatory response (6). In the longer term, pathologic changes to small and medium sized vessels in the brain demonstrate wall thickening and luminal narrowing leading to decreased blood flow and ischemia to the sensitive myelin sheath. Damaged glial cells following radiation may also induce an inflammatory response, resulting in the initial decline in memory function (7).
Transient recovery of memory function is, in some patients, ultimately followed by an irreversible impairment most notably in declarative memory. The cause of this is less well understood, however, a number of sources have been proposed. One of the most widely accepted causes to the long term impairment in memory involves the hippocampus. Neural stem cells located in the subgranular zone of the hippocampus play a role in regenerative processes, including replenishing depleted neurons. When these cells are damaged, or in a pro-inflammatory environment, the stem cells shift from their classical neuronal differentiation ability to a predominantly glial proliferation pattern, thereby resulting in neurocognitive impairment (8). Damage is likely mediated through excitotoxicity following radiation resulting in decreased N-methyl-D-aspartate (NMDA) receptor density and increased gamma-Aminobutyric acid (GABA) receptor density. Animal models have demonstrated this reorganization in the dentate gyrus of the hippocampus which correlates with impairment in long term potentiation (LTP), critical for new memory formation (9).
Finally, global white matter changes have been demonstrated in patients treated with WBRT. A recent study compared radiographic changes on T2-weighted axial magnetic resonance imaging (MRI) in breast cancer patients treated with either SRS alone or SRS and WBRT. The patients were graded based on the degree of white matter change at the time of initial treatment and at a median of 1 year from radiation. Grade 1 was defined as little or no white matter change, grade 2 as limited perventricular hyperintensity, and grade 3 as diffuse white matter hyperintensity. At 1 year the SRS and WBRT group showed a 71.5% increase in white matter change compared to a 3.3% increase in the SRS alone group. While this study did not include formal neurocognitive and quality of life assessments, it clearly demonstrated an increased risk of persistent white matter changes in patients treated with SRS and WBRT (10).
Metastatic involvement of the hippocampus as a cause for memory decline
Brain metastases have been demonstrated to involve or closely approximate the hippocampus which could directly damage neurons responsible for memory function. Progression of these lesions or treatment with local modalities would have a similar or worse effect on memory decline as WBRT. Fortunately, metastases to these regions are relatively rare. Gondi et al. reported that of 371 patients presenting with 1,133 brain metastases none demonstrated disease within the hippocampus itself and only 8.6% were within 5 mm of the hippocampus (11).
Depression and distress as a cause for memory decline
Depression and distress are common comorbidities in cancer patients especially those with brain metastases undergoing treatment. Cordes et al. explored the prevalence of depression and distress along with anxiety in patients who had brain metastases compared to a control breast cancer patient group without brain metastases. They reported a significant increase in distress in the brain metastases group prior to treatment based on the National Comprehensive Cancer Network’s (NCCN) Distress Thermometer (DT) (12).
Emotional distress and depression can significantly impact quality of life through a number of manifestations including depressed mood, insomnia, and fatigue among others. Recent research on the relationship between emotional state and cognition including memory function has been demonstrated. This link is largely mediated through motivation and may play a role in loss of memory function in patients with brain metastases that is likely additive to treatment related cognitive decline (13).
Alternative causes of memory decline
Additional sources of cognitive decline in patients with brain metastases include treatment medications and the disease itself. Nieder et al. performed a retrospective analysis of patients treated with WBRT and antiepileptic medication. They reported a strong correlation (P=0.01) between patients treated with carbamazepine and late radiation toxicity, defined as a deterioration in the neurologic condition more than 3 months after WBRT not related to residual or recurrent tumor (14). While it is unclear whether the use of antiepileptic medications contributes to the pathogenesis of radiation induced neurocognitive decline or if the effects are merely additive, there is a well-established neurotoxicity associated with their use. Meador et al. showed that healthy subjects treated with either carbamazepine or phenytoin for 1 month showed significantly impaired memory performance along with mild EEG slowing (15).
Neurotoxic chemotherapeutics such as nucleoside analogs and antifolates also contribute to a decline in memory function in patients treated for brain metastases. For example methotrexate has been shown to alter folate physiology in the brain resulting in increased levels of homocysteine which contributes to endothelial damage, occlusive vascular disease, and excitotoxicity. Additionally, elevated s-adenosylhomocysteine, a result of methotrexate, inhibits the methylation of the myelin sheath by s-adenosylmethionine leading to white matter damage (16).
Corticosteroid use in patients with brain metastases is another cause for cognitive dysfunction. Changes in mood and sleep disturbances related to steroid use certainly affect cognitive function but measurable effects on declarative memory even during short term steroid use have been reported. The severity of memory impairment, however, does correlate to both dose and duration of use (17). Steroids may play a role in directly affecting memory centers of the brain. Brown et al. compared hippocampal volumes in patients treated with ≥10 mg/d of prednisone for ≥6 months to those that had no recent prednisone exposure and found an 8% and 9% decrease in volume in the left and right hippocampi respectively along with lower scores on tests of memory and higher scores on tests for depression (18).
Finally, paraneoplastic syndromes associated with certain cancers such as SCLC may play a role in the development of cognitive dysfunction. Komaki et al. examined 30 patients who had received chemotherapy for SCLC prior to prophylactic cranial irradiation and found deficits in verbal memory, frontal lobe function, and motor coordination in 97%. The authors acknowledged that the deficits seen in these patients may be related to prior chemotherapy; however, the pattern resembled frontal-subcortical dysfunction, similar to that seen in paraneoplastic limbic encephalopathy (19). Furthermore, most reported neurotoxic effects of chemotherapy are acute, reversible, and not as prevalent as those seen in this cohort. In a group of 47 cancer patients with non-small cell solid tumors only 18% treated with non-biologic chemotherapy showed signs of impairment (20).
Prevention strategies for neurocognitive decline due to WBRT
Preventing neurocognitive decline due to WBRT involves avoiding or limiting the processes that damage healthy brain tissue. A number of strategies have been explored, including avoiding WBRT, avoiding the hippocampi during WBRT, protecting brain tissue from excitotoxicity, and preventing neurotransmitter receptor remodeling in the hippocampus.
SRS or surgery without adjuvant WBRT
One possibly effective method to prevent loss of memory function in patients with brain metastases is to avoid WBRT altogether. This could be accomplished by either surgical resection or SRS alone. Avoidance of WBRT would theoretically spare the hippocampal stem cell compartments and prevent the white matter changes seen following irradiation. This would be an ideal strategy for patients whose lesions are amenable to such focal therapeutic approaches and those who simultaneously have a low risk of microscopic spread, and hence subsequent emergence of brain metastatic disease. Patchell et al. compared patients with a single brain metastases who underwent surgical resection vs. resection followed by WBRT and showed no difference in overall survival, although the study was not powered for this endpoint. However, there was a significantly lower local (10% vs. 46%) and regional (18% vs. 70%) recurrence rate as well as a decrease in death due to neurological dysfunction in favor of adjuvant WBRT (21).
A subsequent trial reported by Aoyama et al. showed similar results with an improvement in the 12 months recurrence rate of 46.8% vs. 76.4% in those treated with SRS and WBRT compared to SRS alone with no change in overall survival, but again with a relatively low number of patients, also making the study underpowered to demonstrate small differences in overall survival (22). In a larger randomized trial conducted by the European Organization for Research and Treatment of Cancer (EORTC), an improvement in local (27% vs. 59%) and regional (23% vs. 42%) recurrence rates with the addition of WBRT following resection or SRS was reported (23).
A patient-specific meta-analysis of the Chang, Patchell, and EORTC trials was recently published (5,22,23). This included 364 patients with 1 to 4 brain metastases treated with either SRS or surgery with or without adjuvant WBRT. Uniform, cohort-wide conclusions could not be drawn, and post-hoc, non pre-specified statistical analysis, were then performed to explore possible subset effects. As expected, the analysis demonstrated significantly better survival and lower risk of distant failure for patients with single metastases than those with 2-4; this observation is important, as several attempts have been made to suggest that the number of brain metastases is somewhat irrelevant, but a thorough review of the literature consistently throws up contradictory observations on this issue. Further, as has been demonstrated almost universally now, better local control across all groups was achieved with the addition of WBRT. Interestingly subgroup analysis showed that age was a significant modifier of overall survival and distant brain failure. Patients less than 50 years old had a significant decrease in mortality (P=0.04) when treated with SRS alone vs. SRS and WBRT while patients greater than 50 had no significant difference in overall survival. Conversely, age had the opposite effect on distant brain failure with no significant difference in patients less than 50 but an increase in distant failure (P=0.04) in those patients treated with SRS alone (24). The authors concluded that avoiding WBRT in patients less than 50 may be the preferred treatment, although of course it is crucial to recognize the retrospective post-hoc un-pre-defined nature of this conclusion, and especially recognize that the conclusions are based on increasingly smaller and smaller subsets of patients.
Hippocampal avoidance whole brain radiotherapy (HA-WBRT)
One of the most consistent domains of functional memory decline seen in patients treated with WBRT is declarative memory, which manifests as decline in delayed recall in a number of clinical trials. The hippocampus plays an integral role in memory formation and the neural stem cells located in the subgranular zone are particularly susceptible to radiation damage (25). When these stem cells are damaged, the neuro-regenerative capacity of the brain is diminished, and a decline in memory ensues. Fortunately, the hippocampi are infrequently involved with metastatic disease, and so it is reasonable to avoid them during WBRT, thereby preserving the neural stem cell compartment without jeopardizing the treatment benefit (11).
Hippocampal-avoidance WBRT (HA-WBRT) is a technique that involves conformal avoidance of the hippocampal stem cell compartments using intensity-modulated radiotherapy (IMRT). Specifically the subgranular zones of the hippocampi are contoured and the dose to this region is reduced while the remaining brain parenchyma receives a typical WBRT dose.
Guidelines for HA-WBRT using IMRT were developed for RTOG-0933, a phase II clinical trial that analyzed the impact of HA-WBRT on declarative memory. The subgranular zones of the hippocampi were contoured with a 5 mm expansion and the dose to 100% of the hippocampus was limited to 9 Gray (Gy) with a maximum dose to the hippocampus limited to 16 Gy (Figure 1). The remaining brain parenchyma received a dose of 30 Gy in 10 fractions (25).
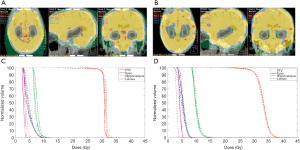
Treatment efficacy was demonstrated with only 4.5% of patients having progression in the hippocampal avoidance region, consistent with the expected event rate. Of the patients analyzed at 4 months there was a mean decline from baseline in HVLT delayed recall (HVLT-DR) of 7% compared to 30% observed in historical controls treated with traditional WBRT (P=0.0003). This low rate is also highly comparable to that observed with SRS alone at a similar point in time in the prospective Chang et al. trial. Quality of life, assessed using the Functional Assessment of Cancer Therapy-Brain (FACT-BR) subscale also showed improvement in emotional well-being over the course of 6 months following treatment with an improved, albeit non-significant, difference in median survival compared to the historical control. Interestingly, higher dose to 100% of the hippocampus (D100%) predicted for greater decline in HVLT-DR. Because HA-WBRT improved memory preservation at 4 and 6 months post treatment without any attenuation of benefit, further evaluation is planned in a phase III randomized trial (NRG CC001) (25,27).
The role of the NMDA receptor
Mitigating the excitotoxicity in the hippocampus following radiation is another area of active research in the preservation of memory function. NMDA receptors in the hippocampus are activated by glutamate and play a role in learning and memory. Over-excitation of these receptors during radiotherapy causes an alteration in the ratio of NMDA to GABA receptors and may lead to neuronal cell death. This has been demonstrated in preclinical models following cranial irradiation and shown to impair LTP which is critical for memory formation (9). Prophylactic application of the noncompetitive NMDA open-channel blocker memantine has been shown to prevent receptor remodeling and preserve LTP in animal models (9).
Memantine’s neuroprotective potential in human patients following irradiation was examined in RTOG 0614. Administration of the drug commenced within 3 days of starting treatment and continued for 24 weeks escalating to a final dose of 10 mg BID. The study demonstrated a non-significant trend towards less decline in HVLT-DR at 24 weeks (P=0.059) in the treatment arm compared to placebo, however this was likely due to patient loss over the 24 weeks and reduced statistical power. Treatment with memantine did demonstrate a significantly longer time to cognitive decline with a probability of cognitive function failure at 24 weeks of 53.8% vs. 64.9% in the placebo arm (P=0.01). Superiority was also shown in the memantine arm for specific tests of executive function, processing speed, and memory. The authors concluded that memantine should be considered in clinical practice to delay cognitive decline with additional benefits in memory, executive function and processing speed (28).
Combination therapy with other strategies to prevent memory decline may further increase the efficacy of memantine. NRG CC001 is a National Cancer Institute approved phase III trial to determine whether hippocampal avoidance in patients treated with WBRT and memantine increases the time to neurocognitive failure (25,27).
RAAS blockade
The RAAS plays an important role throughout the body in maintaining homeostasis of fluid and electrolytes as well as cardiovascular function. Blocking the RAAS using either angiotensin converting enzyme inhibitors (ACE-I) or angiotensin II receptor antagonists (AT1RA) has long been used to modulate this system for blood pressure control and has shown additional benefit in preventing detrimental tissue remodeling in the setting of heart failure and diabetic nephropathy. ACE-I and AT1RA have also been shown to improve cognitive function in patients treated for hypertension and in preventing radiation induced pneumonitis, at least in pre-clinical models (29,30).
Robbins et al. reported on a preclinical study investigating the effect of L-158,809, an AT1RA, on cognitive function in rats treated with WBRT, showing improvement in a “novel object recognition task” designed to assess recognition memory at 26 and 52 weeks. Improvements in recognition memory were seen in rats treated for the entirety of the experiment as well as those treated for only 5 weeks post-irradiation, suggesting an early and persistent effect on cognitive function. They concluded that based on the safety profile, widespread use, antitumor effect and potential benefit on improving neurocognitive function AT1RA’s should be considered in clinical trials for patients receiving WBRT (31).
Treatment of patients with neurocognitive decline
Management of neurocognitive decline in patients with brain metastases is difficult as the damage has already been done and is ultimately irreversible. Treatment largely focuses on symptomatic relief and minimizing comorbidities that may negatively influence cognitive function. Currently no standard treatment exists, however, a number of strategies may be employed to improve the quality of life for patients including treatment of comorbid depression, inhibition of acetyl cholinesterase, neurocognitive rehabilitation strategies, and treating CNS glycolytic metabolic paresis.
Treatment of depression
The prevalence of depression is extremely high in cancer patients affecting as many as 44% of newly diagnosed cases and should be an element of any comprehensive treatment plan (32). This is especially true in the case of patients with brain malignancies where depression can exacerbate pre-existing cognitive dysfunction, further affecting quality of life. Treatment of depression in cancer can take many forms including standard treatment with antidepressants and psychiatric counseling as well as controlling any pain that may exacerbate depression (33).
Patients with brain metastases may benefit from stimulant medications for both the treatment of depression and cognitive slowing associating with brain irradiation. Methylphenidate acts as a stimulant dopamine receptor agonist improving fatigue, concentration, and depression in patients with radiation induced cognitive decline (34). Meyers et al. reported on a series of brain tumor patients with progressive disease following chemotherapy and radiation treated with methylphenidate and showed significant symptomatic improvement in cognition, daily functioning, energy, mood, and ambulation. The authors concluded that the dramatic improvement in cognitive function could not simply be explained by improved mood but by increased motivation leading to improvements in goal directed behavior (35).
Acetyl cholinesterase inhibitors
Similarities between Alzheimer’s dementia (AD) and radiation induced neurocognitive decline clinically and at the neurotransmitter level suggest a potential overlap in symptomatic treatment (36). Donepezil is an acetyl cholinesterase inhibitor that results in elevated levels of the neurotransmitter acetylcholine in the neural synapse and has proven effective in delaying cognitive impairment in AD in multiple clinical trials (37).
Recently donepezil has been investigated in the treatment of radiation induced neurocognitive decline in an open label phase II trial on patients that received either partial brain radiation or WBRT. Shaw et al. reported significant improvements on tests of concentration, verbal memory, and figural memory with a trend toward significant improvement in verbal fluency (38). A follow up randomized controlled phase III trial found no significant improvement on cognitive function in brain tumor survivors following radiation compared to placebo. Subset analysis of patients with more cognitive problems at baseline did, however, demonstrate significant improvement in verbal memory (39).
Neurocognitive rehabilitation strategies
Neurocognitive rehabilitation is a broad topic aimed at improving declining cognitive function be it through normal aging, mild cognitive impairment (MCI), traumatic brain injury (TBI), dementia, or any other condition affecting cognition (40). Rehabilitation involves participation in exercise as well as socially and cognitively stimulating activities and may be tailored to the individual patient’s needs. For example, reinforcement of previously learned behaviors, creating novel cognitive mechanisms to compensate for impaired neurologic systems, utilizing external support mechanisms to compensate for cognitive deficits, and enabling adaptation to their cognitive disability (41).
A retrospective analysis of Alzheimer’s patients treated with donepezil showed an improvement in mini-mental state examination (MMSE) score from 21.7 to 24.0 (P<0.001) in patients that received cognitive rehabilitation including physical therapy, occupational therapy and speech therapy for 1-2 hours once or twice a week over the course of 1 year. The group that was treated with donepezil alone showed no improvement in MMSE score (21.5) (42). The Finnish Geriatric Intervention Study to Prevent Cognitive Impairment and Disability (FINGER) showed similar results with long term multidomain intervention to prevent cognitive decline including dietary changes, exercise, cognitive training, and vascular risk monitoring. Ngandu et al. reported significant improvements in executive functioning (P=0.04) and processing speed (P=0.03) although less of an improvement in the memory domain (P=0.36). Ad hoc analysis of an abbreviated memory domain involving associative memory and logical memory did show significant improvement in the treatment group (P=0.04) (43).
The Veterans Affairs San Diego Healthcare System conducted a randomized prospective study on 50 veterans that had suffered mild to moderate TBI with documented cognitive impairment comparing the use of a 3-month cognitive rehabilitation program called cognitive symptom management and rehabilitation therapy (CogSMART) to control therapy. The domains targeted by CogSMART and their associated strategies are shown in Table 1. Twamley et al. reported significant reductions in postconcussive symptoms (Cohen d =0.97) and improvements in prospective memory function (Cohen d =0.72) in the treatment arm. Smaller effect sizes were noted for posttraumatic stress disorder symptom severity, depressive symptom severity, and attainment of competitive work within 14 weeks (Cohen d =0.35-0.49). Subjectively, the patients receiving CogSMART rated it highly and the authors suggested that further evaluation in a larger trial needs to be done (44).
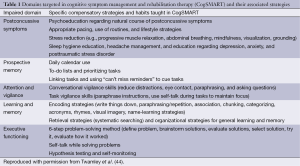
Full table
Improving CNS glycolytic metabolism
Research into the relationship between type 2 diabetes mellitus (T2DM) and AD has revealed a common pathology of insulin resistance and amyloidogenesis leading to impaired glucose metabolism and increased oxidative stress, which in the brain leads to cellular toxicity, impaired energy metabolism, and cognitive deficits (45). Administering insulin intranasally allows it to directly enter the CSF reducing the risk of systemic hypoglycemia while overcoming insulin resistance in the brain (46).
A phase II clinical trial, SNIFF 120, investigated the neurocognitive effect of intranasal insulin on patients with AD and amnestic mild cognitive impairment (aMCI). The study randomized 111 patients to receive intranasal insulin, at either 20 or 40 international units (IU) per day or placebo. The 20 and 40 IU treatment arms both demonstrated preserved caregiver-rated functional ability (P<0.01), general cognition assessed by the ADAS-cog score and preserved functional ability assessed by the ADCS-ADL scale (P<0.05). Interestingly, only the 20 IU treatment arm showed improved delayed memory recall (P<0.05) suggesting an optimal effect not realized with a higher or lower dose. A subset of patients also underwent F-18 fluordeoxyglucose positron emission tomography (18F-FDG PET) at 4 months into treatment which demonstrated preserved or improved glucose uptake in both treatment arms and decreased glucose uptake in the placebo arm (47).
Intranasal insulin has also been shown to modify resting state connectivity between hippocampal regions and the default mode network (DMN) including the medial frontal cortex (MFC) (48). Network activity between the hippocampi and the DMN is thought to be responsible for attending to environmental stimuli, reviewing past knowledge in preparation of future action, and episodic memory processing (49). Activity in this network has been shown to be deficient in both AD and T2DM (48,49).
Zhang et al. demonstrated alterations in network connectivity after the administration of 40 IU of intranasal insulin in older patients with T2DM compared to healthy controls using functional magnetic resonance imaging (fMRI). Diabetic patients treated with placebo showed a lower resting state connectivity than healthy controls (P=0.02) while those treated with intranasal insulin showed a similar connectivity to control. Cognitive performance was correlated to higher resting state connectivity (48).
Intranasal insulin has yet to be explored in patients with cognitive decline due to brain radiation. However, the similarities with AD in symptomatology and treatment response and the well described role the hippocampus has in radiation induced cognitive decline make intranasal insulin an attractive area of investigation, especially in patients in whom the baseline FDG-PET demonstrates impaired activity.
Conclusions
The diagnosis of metastatic cancer to the brain is devastating in of itself; however, living with the sequelae of treatment can also be burdensome. Cognition and memory are two of the most essential human traits and to lose them significantly impacts a patient’s quality of life. Neurocognitive decline following treatment of brain metastases is largely multifactorial, and the treatments used in controlling the disease contribute to this, especially WBRT. However there are many cases were WBRT is critical for a patient’s survival, or for controlling compartmental disease. Therefore it is essential that strategies are developed and implemented to prevent the onset of neurocognitive decline as far as possible, and when not possible, treat the symptoms as they arise.
Primary prevention strategies include avoiding WBRT, implementing HA-WBRT, using the NMDA receptor modulator memantine prophylactically, or blocking the RAAS cascade prophylactically. In certain circumstances avoiding WBRT in lieu of a focal treatment such as resection or SRS may be advantageous, particularly in younger patients (<50). However, these patients are at greater risk of local failure requiring salvage if WBRT is withheld. A strong case can be made to use adjuvant WBRT in patients with more than three brain metastases given the risk of distant brain failure and the absence of any level 1 evidence evaluating the overall survival impact of withholding WBRT. Patients who require WBRT may benefit from HA-WBRT, but this warrants further evaluation in a phase III study (NRG CC001). Avoiding the areas of the hippocampus that contain essential neuroregenerative stem cells was significantly effective at reducing neurocognitive decline 6 months out from treatment without attenuating the effect of treatment. Neuroprotective agents like memantine and RAAS blockers also show promise in preventing the magnitude of neurocognitive decline following brain irradiation and are both well tolerated pharmaceuticals making them excellent candidates as prophylactic treatment during WBRT.
Unfortunately primary prevention will not entirely eliminate neurocognitive decline so it is necessary to find suitable treatments to improve the quality of life in patients. Depression is common in cancer patients and can exacerbate pre-existing deficits in cognition so screening should occur and appropriate pharmaceutical or psychological treatment should be used if indicated. AD and radiation induced neurocognitive decline share many similarities including symptomatology, radiographic findings, and neurotransmitter changes so it is reasonable to look for similar treatments. The acetyl cholinesterase inhibitor donepezil showed significant improvement in verbal memory in patients with more cognitive problems at baseline so may be appropriate for those patients, although it failed to show significance overall. Neurocognitive rehabilitation has also been used successfully for patients with dementia, TBI, and other forms of cognitive decline. It is relatively inexpensive however it does require compliance from the patient and the caregiver. Finally intranasal insulin has produced some exciting results for cognitive improvement in AD by increasing glucose metabolism in the brain as well as in T2DM by altering resting state activity in neural networks thought to be responsible for attention, episodic memory, and recall. While it has not been tested in radiation induced cognitive decline the results in AD and T2DM warrant consideration.
All of these prevention and treatment strategies have shown benefit in their own right. The optimum strategy to prevent neurocognitive decline will be to create a combination of primary prevention strategies and treatments based on the individual patient’s needs.
Acknowledgements
Disclosure: Nicholas B. Dye—no relationship to disclose; Vinai Gondi—teaching honoraria: US Oncology; Minesh P. Mehta—leadership: Pharmacyclics; stock or other ownership: Pharmacyclics; consulting: Cavion, Elekta, Novartis, Novocure; research funding: Novocure, Novellos.
References
- Chao JH, Phillips R, Nickson JJ. Roentgen-ray therapy of cerebral metastases. Cancer 1954;7:682-9. [PubMed]
- Gaspar LE, Mehta MP, Patchell RA, et al. The role of whole brain radiation therapy in the management of newly diagnosed brain metastases: a systematic review and evidence-based clinical practice guideline. J Neurooncol 2010;96:17-32. [PubMed]
- Kondziolka D, Patel A, Lunsford LD, et al. Stereotactic radiosurgery plus whole brain radiotherapy versus radiotherapy alone for patients with multiple brain metastases. Int J Radiat Oncol Biol Phys 1999;45:427-34. [PubMed]
- Slotman B, Faivre-Finn C, Kramer G, et al. Prophylactic cranial irradiation in extensive small-cell lung cancer. N Engl J Med 2007;357:664-72. [PubMed]
- Chang EL, Wefel JS, Hess KR, et al. Neurocognition in patients with brain metastases treated with radiosurgery or radiosurgery plus whole-brain irradiation: a randomised controlled trial. Lancet Oncol 2009;10:1037-44. [PubMed]
- Armstrong C, Ruffer J, Corn B, et al. Biphasic patterns of memory deficits following moderate-dose partial-brain irradiation: neuropsychologic outcome and proposed mechanisms. J Clin Oncol 1995;13:2263-71. [PubMed]
- Crossen JR, Garwood D, Glatstein E, et al. Neurobehavioral sequelae of cranial irradiation in adults: a review of radiation-induced encephalopathy. J Clin Oncol 1994;12:627-42. [PubMed]
- Hua K, Schindler MK, McQuail JA, et al. Regionally distinct responses of microglia and glial progenitor cells to whole brain irradiation in adult and aging rats. PLoS One 2012;7:e52728. [PubMed]
- Wu PH, Coultrap S, Pinnix C, et al. Radiation induces acute alterations in neuronal function. PLoS One 2012;7:e37677. [PubMed]
- Stokes TB, Niranjan A, Kano H, et al. White matter changes in breast cancer brain metastases patients who undergo radiosurgery alone compared to whole brain radiation therapy plus radiosurgery. J Neurooncol 2015;121:583-90. [PubMed]
- Gondi V, Tomé WA, Mehta MP. Why avoid the hippocampus? A comprehensive review. Radiother Oncol 2010;97:370-6. [PubMed]
- Cordes MC, Scherwath A, Ahmad T, et al. Distress, anxiety and depression in patients with brain metastases before and after radiotherapy. BMC Cancer 2014;14:731. [PubMed]
- Son Y, Yang M, Wang H, et al. Hippocampal dysfunctions caused by cranial irradiation: a review of the experimental evidence. Brain Behav Immun 2015;45:287-96. [PubMed]
- Nieder C, Leicht A, Motaref B, et al. Late radiation toxicity after whole brain radiotherapy: the influence of antiepileptic drugs. Am J Clin Oncol 1999;22:573-9. [PubMed]
- Meador KJ, Loring DW, Abney OL, et al. Effects of carbamazepine and phenytoin on EEG and memory in healthy adults. Epilepsia 1993;34:153-7. [PubMed]
- Cole PD, Kamen BA. Delayed neurotoxicity associated with therapy for children with acute lymphoblastic leukemia. Ment Retard Dev Disabil Res Rev 2006;12:174-83. [PubMed]
- Brown ES. Effects of glucocorticoids on mood, memory, and the hippocampus. Treatment and preventive therapy. Ann N Y Acad Sci 2009;1179:41-55. [PubMed]
- Brown ES. Hippocampal volume, spectroscopy, cognition, and mood in patients receiving corticosteroid therapy. Biol Psychiatry 2004;55:538-45. [PubMed]
- Komaki R, Meyers CA, Shin DM, et al. Evaluation of cognitive function in patients with limited small cell lung cancer prior to and shortly following prophylactic cranial irradiation. Int J Radiat Oncol Biol Phys 1995;33:179-82. [PubMed]
- Meyers CA, Abbruzzese JL. Cognitive functioning in cancer patients: effect of previous treatment. Neurology 1992;42:434-6. [PubMed]
- Patchell RA, Tibbs PA, Regine WF, et al. Postoperative radiotherapy in the treatment of single metastases to the brain: a randomized trial. JAMA 1998;280:1485-9. [PubMed]
- Aoyama H, Shirato H, Tago M, et al. Stereotactic radiosurgery plus whole-brain radiation therapy vs stereotactic radiosurgery alone for treatment of brain metastases: a randomized controlled trial. JAMA 2006;295:2483-91. [PubMed]
- Kocher M, Soffietti R, Abacioglu U, et al. Adjuvant whole-brain radiotherapy versus observation after radiosurgery or surgical resection of one to three cerebral metastases: results of the EORTC 22952-26001 study. J Clin Oncol 2011;29:134-41. [PubMed]
- Sahgal A, Aoyama H, Kocher M, et al. Phase 3 trials of stereotactic radiosurgery with or without whole-brain radiation therapy for 1 to 4 brain metastases: individual patient data meta-analysis. Int J Radiat Oncol Biol Phys 2015;91:710-7. [PubMed]
- Gondi V, Pugh SL, Tome WA, et al. Preservation of memory with conformal avoidance of the hippocampal neural stem-cell compartment during whole-brain radiotherapy for brain metastases (RTOG 0933): a phase II multi-institutional trial. J Clin Oncol 2014;32:3810-6. [PubMed]
- Dye NB, Gondi V, Brown PD, et al. Whole brain radiotherapy: is the therapeutic window enlarging? In: Hayat MA, editor. Brain metastases from primary tumors. volume 2, 1st edition. Amsterdam: Academic Press-Elsevier, 2015.
- NRG Oncology. Memantine hydrocholoride and whole-brain radiotherapy with or without hippocampal avoidance in reducing neurocognitive decline in patients with brain metastases. Available online: http://clinicaltrials.gov/ct2/show/NCT02360215
- Brown PD, Pugh S, Laack NN, et al. Memantine for the prevention of cognitive dysfunction in patients receiving whole-brain radiotherapy: a randomized, double-blind, placebo-controlled trial. Neuro Oncol 2013;15:1429-37. [PubMed]
- Tedesco MA, Ratti G, Di Salvo G, et al. Does the angiotensin II receptor antagonist losartan improve cognitive function? Drugs Aging 2002;19:723-32. [PubMed]
- Molteni A, Moulder JE, Cohen EF, et al. Control of radiation-induced pneumopathy and lung fibrosis by angiotensin-converting enzyme inhibitors and an angiotensin II type 1 receptor blocker. Int J Radiat Biol 2000;76:523-32. [PubMed]
- Robbins ME, Payne V, Tommasi E, et al. The AT1 receptor antagonist, L-158,809, prevents or ameliorates fractionated whole-brain irradiation-induced cognitive impairment. Int J Radiat Oncol Biol Phys 2009;73:499-505. [PubMed]
- Derogatis LR, Morrow GR, Fetting J, et al. The prevalence of psychiatric disorders among cancer patients. JAMA 1983;249:751-7. [PubMed]
- Rodin G, Katz M, Lloyd N, et al. Treatment of depression in cancer patients. Curr Oncol 2007;14:180-8. [PubMed]
- Weitzner MA, Meyers CA, Valentine AD. Methylphenidate in the treatment of neurobehavioral slowing associated with cancer and cancer treatment. J Neuropsychiatry Clin Neurosci 1995;7:347-50. [PubMed]
- Meyers CA, Weitzner MA, Valentine AD, et al. Methylphenidate therapy improves cognition, mood, and function of brain tumor patients. J Clin Oncol 1998;16:2522-7. [PubMed]
- Dimberg Y, Vazquez M, Söderström S, et al. Effects of X-irradiation on nerve growth factor in the developing mouse brain. Toxicol Lett 1997;90:35-43. [PubMed]
- Wolfson C, Oremus M, Shukla V, et al. Donepezil and rivastigmine in the treatment of Alzheimer's disease: a best-evidence synthesis of the published data on their efficacy and cost-effectiveness. Clin Ther 2002;24:862-86; discussion 837. [PubMed]
- Shaw EG, Rosdhal R, D'Agostino RB Jr, et al. Phase II study of donepezil in irradiated brain tumor patients: effect on cognitive function, mood, and quality of life. J Clin Oncol 2006;24:1415-20. [PubMed]
- Rapp SR, Case D, Peiffer A, et al. Phase III randomized, double-blind, placebo-controlled trial of donepezil in irradiated brain tumor survivors. J Clin Oncol 2013;31:A2006.
- Basford JR, Malec JF. Brief overview and assessment of the role and benefits of cognitive rehabilitation. Arch Phys Med Rehabil 2015;96:977-80. [PubMed]
- Cicerone KD, Dahlberg C, Kalmar K, et al. Evidence-based cognitive rehabilitation: recommendations for clinical practice. Arch Phys Med Rehabil 2000;81:1596-615. [PubMed]
- Matsuzono K, Hishikawa N, Takao Y, et al. Combination benefit of cognitive rehabilitation plus donepezil for Alzheimer's disease patients. Geriatr Gerontol Int 2015. [Epub ahead of print]. [PubMed]
- Ngandu T, Lehtisalo J, Solomon A, et al. A 2 year multidomain intervention of diet, exercise, cognitive training, and vascular risk monitoring versus control to prevent cognitive decline in at-risk elderly people (FINGER): a randomised controlled trial. Lancet 2015. [Epub ahead of print]. [PubMed]
- Twamley EW, Jak AJ, Delis DC, et al. Cognitive Symptom Management and Rehabilitation Therapy (CogSMART) for veterans with traumatic brain injury: pilot randomized controlled trial. J Rehabil Res Dev 2014;51:59-70. [PubMed]
- Zhao WQ, Townsend M. Insulin resistance and amyloidogenesis as common molecular foundation for type 2 diabetes and Alzheimer's disease. Biochim Biophys Acta 2009;1792:482-96.
- Gottschalk A, Ellger B. Whenever you lose connection, take intranasal insulin? Diabetes 2015;64:687-8. [PubMed]
- Craft S, Baker LD, Montine TJ, et al. Intranasal insulin therapy for Alzheimer disease and amnestic mild cognitive impairment: a pilot clinical trial. Arch Neurol 2012;69:29-38. [PubMed]
- Zhang H, Hao Y, Manor B, et al. Intranasal insulin enhanced resting-state functional connectivity of hippocampal regions in type 2 diabetes. Diabetes 2015;64:1025-34. [PubMed]
- Greicius MD, Srivastava G, Reiss AL, et al. Default-mode network activity distinguishes Alzheimer's disease from healthy aging: evidence from functional MRI. Proc Natl Acad Sci U S A 2004;101:4637-42. [PubMed]